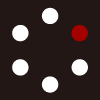
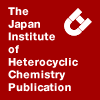
HETEROCYCLES
An International Journal for Reviews and Communications in Heterocyclic ChemistryWeb Edition ISSN: 1881-0942
Published online by The Japan Institute of Heterocyclic Chemistry
e-Journal
Full Text HTML
Received, 30th July, 2012, Accepted, 16th August, 2012, Published online, 17th August, 2012.
DOI: 10.3987/COM-12-12558
■ SYNTHESIS OF DIhydrodiBENZo[b,f]furo[2,3-d]OXEPINs AND RELATED REACTIONS
Yasunobu Maemura, Yuka Tanoue, and Hiroshi Nishino*
Department of Chemistry, Graduate School of Science and Technology, Kumamoto University, Kurokami 2-39-1, Chûou-Ku, Kumamoto 860-8555, Japan
Abstract
Nine dihydrodibenzo[b,f]furo[2,3-d]oxepin derivatives were synthesized by the Mn(III)-based oxidative addition of dibenz[b,f]oxepins at C10-C11 with acetic anhydride, acetylacetone, dialkyl malonates, and malonamide. Oxidative ring-expansion of methyl hydrogen thioxanthenylmalonate with manganese(III) acetate did not give the desired dibenzo[b,f]thiepincarboxylate but produced 2-acetoxy-2-(thioxanthen-9-yl)acetate and thioxanthone.INTRODUCTION
Dibenz[b,f]oxepin derivatives are one of the unique heterocyclic compounds1 which were isolated from a natural source, for example, Bauhinia racenosa,2 Salvia chinensis,3 Artocarpus rigida,4 Bauhinia saccocalyx,5 Bauhinia purpurea,6 and Cercis chinensis,7 and exhibit an important number of biological activities. Therefore, some of them are used as pharmaceutical drugs and, to date, still are synthetic targets from the pharmaceutical point of view.8 The dibenz[b,f]oxepins are usually prepared by the intramolecular C-O ether bond formation of 2-styrylphenols and the cyclodehydration of preformed diaryl ether intermediates.1 Recently, we reported a new approach to the dibenz[b,f]oxepin skeleton via an unusual Mn(III)-based oxidative 1,2-arylradical rearrangement (Scheme 1).9 Because the dibenz[b,f]oxepin includes an alkenic double bond between C-10 and C-11, we decided to explore the oxidative addition of some active methylene compounds using manganese(III) acetate in order to construct a more annulated ring system. In addition, the synthesis of a dibenzothiepin derivative was also attempted according to the method described in Scheme 1.
RESULTS AND DISCUSSION
We first investigated lactone-annulation to the alkenic double bond of dibenzoxepincarboxylate 1a (Scheme 2 and Table 1).10 The reaction was carried out under typical reaction conditions in the presence of acetic anhydride.11 To our delight, using a stoichiometric amount of manganese(III) acetate afforded the desired lactone 2a together with 1a unchanged (Table1, entry 1). In order to optimize the reaction conditions, we examined the molar ratio of the oxidant and volume of the solvent and finally obtained 2a in the best yield (entry 7).
The structure of the lactone 2a was deduced by spectroscopic methods. A characteristic absorption band assigned to a γ-lactone carbonyl group appeared at 1781 cm-1 in the IR spectrum. A vinyl proton at δ 7.90 ppm corresponding to H-11 of 1a disappeared and instead showed a typical AB2 pattern at δ 4.50 (1H, t, J = 10.0 Hz) and 3.01 ppm (2H, d, J = 10.0 Hz) due to H-3a methyne and H-3 methylene protons. In the 13C NMR spectrum, signals at δ 138.1 and 131.2 ppm corresponding to sp2 carbons in 1a disappeared and a quaternary, a methyne, and a methylene carbon newly appeared at δ 86.1, 44.4, and 33.6 ppm, respectively. In the MS spectrum, the corresponding molecular ion peak appeared at m/z 310 which supported the molecular weight C18H14O5. Because the combustion analysis of the product 2a was consistent with the molecular formula C18H14O5, the product 2a was finally determined to be methyl 2-oxo-2,3,3a,12b-tetrahydrodibenzo[b,f]furo[2,3-d]oxepin-12b-carboxylate (see Experimental). In addition, the stereochemistry between the 3a-H proton and the methoxycarbonyl group was a cis configuraton based on the NOESY spectrum; that is, the lactone ring underwent cis addition to the oxepin ring.
It is well-known that the addition of acetic anhydride caused acceleration of the reaction rate,11 and therefore, it is obvious that carboxymethyl radicals A are generated via a single electron-transfer (SET) oxidation of an acetic anhydride-Mn(III) enolate complex similar to that of the normal 1,3-dicarbonyl-Mn(III) enolate system (Scheme 3).12 The radical A attacks the alkenic double bond of 1a at C-11 to give a tertiary oxepin radical which would be easily oxidized and cyclized. Because the carboxymethyl radical A is electron-deficient, it is advantageous for an electron-rich alkene, that is, an alkene having a lower ionization potential (IP), to undergo the lactonization. Thus, we converted the electron-withdrawing methoxycarbonyl group of 1a (IP = 7.12 eV)13 into an electron-donating methoxymethyl group by reduction followed by methylation. With the methoxymethyldibenzoxepin 1b (IP = 6.67 eV)13 in hand, we explored a similar lactonization of 1b. As a result, the yield increased more than 10% as expected (entry 8).
We next investigated the Mn(III)-based annulation of dibenzoxepins 1a,b using 1,3-dicarbonyl compounds such as malonic acid,14 acetylacetone,15 dialkyl malonates,16 and malonamide.17
Although the reaction of 1a with malonic acid (3a) was examined under various reaction conditions, only malonic acid (3a) was oxidized and unchanged 1a was recovered (Table 2, entry 1); thus the annulation using 3a failed. The reaction of 1a with acetylacetone (3b) resulted in an intractable mixture even though the reaction time was relatively shorter (entry 2). However, the desired dihydrofuran-fused oxepin 4bb was obtained by the reaction using methoxymethyl-substituted oxepin 1b (Scheme 4 left and Table 2, entry 3). When the reaction of dibenzoxepins 1a,b was carried out using dimethyl (3c) and diethyl malonate (3d), a longer reaction time was needed to consume the oxidant. After a normal work-up, the corresponding butenolide-fused oxepins 4ac and 4ad from 1a were obtained in good yields, while the reaction of 1b was complicated and similar oxepins 4bc and 4bd were isolated in poor yields (Scheme 4 right and Table 2, entries 4, 5, 7, and 8). Probably, the methoxymethyl 1b having a lower ionization potential (IP = 6.67 eV) might be more sensitive under the oxidation conditions than the methoxycarbonyl 1a (IP = 7.12 eV). Finally, the reaction using malonamide (3e) gave similar butenolide-annulated oxepins 4ae and 4be entries 6 and 9). Unfortunately, a pyrrol-2(5H)-one-fused
oxepin derived derived from N-cyclization of the carbamoyl group was not isolated.17
A variety of compounds containing the dibenzo[b,f]thiepin framework is also attractive based on their unique biological activity,8,18 and we next explored a similar synthesis using 9H-thioxanthen-9-one instead of xanthone as shown in Scheme 5. Thioxanthone was reduced in a manner similar to that described above to afford thioxanthene in 35% unoptimized yield, which was oxidized with manganese(III) acetate in the presence of dimethyl malonate followed by controlled hydrolysis, giving methyl hydrogen thioxanthenylmalonate (5). With the monoester 5 in hand, the oxidative ring-expansion was investigated under various reaction
conditions. Unfortunately, the desired dibenzo[b,f]thiepincarboxylate 6 was not obtained, but 2-acetoxy-2-(thioxanthen-9-yl)acetate 7 and thioxanthone were produced in 63% and 16% yields, respectively.
In order to prevent the substitution of an acetoxy group, the reaction was also conducted using manganese(III) tris(2-pyridinecarboxylate), [Mn(pic)3], in dimethylformamide or ethanol. However, only a small amount of decarboxylated thioxanthenes 8 and 9 together with thioxanthone was isolated (Scheme 6), and all attempts failed (see Experimental). Probably, the thioxanthenemalonate 5 must be a boat conformation B because of the large sulfur atom,19 and then the enolization from B to C might be difficult due to the steric repulsion.9a Therefore, the oxidative decarboxylation from B predominantly occurred (D) and the acetate 7 was successively produced (compare Scheme 6 with Scheme 1).
CONCLUSION
We achieved the annulation of dibenzoxepins 1 at C10-C11 using the typical Mn(III)-based oxidative radical cyclization, and the lactone- and dihydrofuran-fused dibenzoxepin derivatives 2 and 4 were synthesized. Although the Mn(III)-based oxidative ring-expansion of thioxanthenylmalonate 5 failed and the desired dibenzo[b,f]thiepincarboxylate 6 was not obtained, we found that the thioxanthenylacetate 7 and thioxanthone were generated. It is thought that the oxidative decarboxylation of 5 occurred before the ring-expansion. The biological screening of 2 and 4 such as antibacterial and herbicidal activity is currently underway.
EXPERIMENTAL
General Information. Melting points were taken using a Yanagimoto micromelting point apparatus and are uncorrected. The IR spectra were measured in CHCl3 or KBr using a Shimadzu FTIR-8400 spectrometer and expressed in wave numbers (cm-1). All of the NMR spectra were obtained on a JNM AL300 or ECX 500 FT-NMR spectrometer at 300 or 500 MHz for 1H and 75 or 125 MHz for 13C, with tetramethylsilane as internal standard. Chemical shifts are reported in δ values (ppm downfield from tetramethylsilane) and the coupling constants in Hz. The EI MS spectra were acquired on a Shimadzu QP-5050A gas chromatograph-mass spectrometer at an ionizing voltage of 70 eV. The high-resolution mass spectra and the elemental analysis were performed at the Instrumental Analysis Center, Kumamoto University, Kumamoto, Japan.
Materials. Manganese(II) acetate tetrahydrate, Mn(OAc)2•4H2O, 9-xanthenone, acetic anhydride, malonic acid, dimethyl malonate, diethyl malonate, and acetylacetone were purchased from Wako Pure Chemical Ind., Ltd., and were used as received. Manganese(III) acetate dihydrate, Mn(OAc)3•2H2O,9-12,14-17 and manganese(III) tris(2-pyridinecarboxylate), [Mn(pic)3],20 were prepared according to the method described in the literature. 9-Thioxanthenone and malonamide were purchased from Kanto Chemical Co., Inc., and Tokyo Chemical Industry Co., Ltd., respectively, and were used as received. Methyl dibenz[b,f]oxepin-10-carboxylate (1a) was prepared by the method described in the literature.9 Flash column chromatography was performed on silica gel 60N (40-50 µm) which was purchased form Kanto Chemical Co., Inc., and thin layer chromatography (TLC) on Wakogel B-10 (45µm) from Wako Pure Chemical Ind., Ltd.
10-(Methoxymethyl)dibenz[b,f]oxepin (1b). To a dried Et2O (100 mL) at 0 °C in a 300-mL round-bottomed flask, LiAlH4 (0.50 g, 13 mmol) was slowly added for 15 min, and 1a (0.83 g, 3.3 mmol) was then added. The mixture was stirred at room temperature for 3 h, and the reaction was quenched by adding water (0.4 mL) at 0 °C. To the resulting aqueous mixture, 15%NaOH aqueous solution (0.4 mL) and additional water (1.2 mL) were added, and the mixture was further stirred at room temperature for 30 min. The resulting mixture was filtered through a Celite column eluting with Et2O. The filtrate was dried over anhydrous MgSO4 and concentrated to dryness, giving 10-(hydroxymethyl)dibenz[b,f]oxepin (0.74 g, quantitative).
To a solution of 10-(hydroxymethyl)dibenz[b,f]oxepin (0.43 g, 1.9 mmol) in dry THF (40 mL), NaH (0.46 g, 19 mmol) was added at 0 °C, and the mixture was stirred for 15 min. Methyl iodide (0.24 mL, 3.9 mmol) was then added, and the mixture was heated under reflux for 2 h. The reaction was quenched by adding water at 0 °C, and the resulting aqueous mixture was extracted with CHCl3; the extract was dried over anhydrous MgSO4 and concentrated to dryness. The residue was separated on silica gel chromatography eluting with CHCl3/hexane (6:4 v/v), giving 10-(methoxymethyl)dibenz[b,f]oxepin (1b).
Data of 10-(Hydroxymethyl)dibenz[b,f]oxepin: Yield (0.74 g, 100%); colorless oil; IR (CHCl3) ν 3603, 3550-3150 (OH), 1603 (C=C); 1HNMR (CDCl3) δ = 7.40-7.08 (8H, m, arom H), 6.90 (1H, s, H-11), 4.67 (2H, s, O-CH2), 2.08 (1H, s, OH); 13CNMR (CDCl3) δ = 158.3, 157.7 (C-4a and C-5a), 139.0 (C-10), 130.1, 130.0, 129.9 (arom C), 129.7, 129.3, 127.6, 126.5, 125.1, 124.9, 121.6, 120.8 (arom CH and C-11), 65.7 (CH2); MS m/z (rel intensity) 224 (M+, 71), 195 (35), 181 (100).
Data of 1b: Yield (0.43 g, 93%); colorless oil; IR (CH3Cl) ν 1603 (C=C), 1094 (C-O-C); 1HNMR (CDCl3) δ = 7.45-7.11 (8H, m, arom H), 6.90 (1H, s, H-11), 4.47 (2H, s, O-CH2), 3.44 (3H, s, OMe); 13CNMR (CDCl3) δ = 158.2, 157.9 (C-4a and C-5a), 136.1 (C-10), 130.3, 130.1, 129.9 (arom C), 129.7, 129.3, 129.2, 126.8, 125.0, 124.8, 121.5, 120.8 (arom CH and C-11), 75.2 (CH2), 57.8 (OMe); MS m/z (rel intensity) 238 (M+, 100), 223 (50), 207 (80), 195 (45), 181 (55), 165 (30), 152 (20); FAB HRMS (acetone/NBA) calcd for C16H14O2 238.0994 (M+). Found 238.0999.
Methyl Hydrogen Thioxanthenylmalonate (5). To a mixture of LiAlH4 (0.97 g, 25 mmol) and dried Et2O (150 mL) in a 500 mL-round-bottomed flask, 9-thioxanthone (5.41 g, 25 mmol) was dropwise-added, and the mixture was heated under reflux for 3 h. After cooling, AlCl3 (3.40 g, 26.5 mmol) was added to the resulting mixture at 0 °C, and the mixture was again heated under reflux for 8 h. After cooling, 6M HCl was added and the resulting solution was extracted with CHCl3. The combined organic layers were washed with a saturated aqueous solution of NaHCO3, water, dried over anhydrous MgSO4, and concentrated. Flash chromatography of the residue on silica gel eluted by CHCl3 gave thioxanthene (4.75 g, 94%), mp 124-127 °C (lit.,21 mp 126-127 °C). The obtained thioxanthene (1.98 g, 10 mmol), dimethyl malonate (5.31 g, 40 mmol), and Mn(OAc)3•2H2O (10.72 g, 40 mmol) were added to glacial AcOH (125 mL) in a 300-mL round-bottomed flask, and the mixture was heated under reflux until the opaque dark-brown color of the solution became transparent brown. After cooling, the solvent was removed in vacuo, and the residue was treated with 2M HCl, extracted with CHCl3, and separated on silica gel column chromatography eluted by CHCl3, affording dimethyl (9-thioxanthenyl)malonate (2.30 g, 70%), mp 109-112 °C (lit.,22 mp 110.5-111 °C). The obtained dimethyl (9-thioxanthenyl)malonate (1.37 g, 4.2 mmol) was hydrolyzed by KOH (0.26 g, 4.6 mmol) in MeOH (42 mL) at room temperature for 24 h. The mixture was acidified with concentrated HCl at 0 °C and extracted with EtOAc. The extract was washed with a saturated aqueous solution of NaHCO3, and the aqueous layer was acidified with concentrated HCl, then again extracted with EtOAc, giving methyl hydrogen thioxanthenylmalonate (5).
Data of 5: Yield (0.81 g, 62%); colorless needles (from MeOH); mp 190-193 °C; IR (CHCl3) ν 3400-2200 (OH), 1746, 1709 (C=O); 1HNMR (CDCl3) δ = 13.0 (1H, br.s, COOH), 7.58-7.36 (8H, m, arom H), 4.93 (1H, d, J = 11.0 Hz, CH), 3.99 (1H, d, J = 11.0 Hz, CH), 3.47 (3H, s, OMe); 13CNMR (CDCl3) δ = 172.8, 172.6 (C=O), 139.4, 139.3, 137.7, 137.6 (arom C), 135.6, 135.1, 132.5 (2C), 132.2, 132.1, 131.8 (2C) (arom CH), 57.2 (OMe), 56.6 (CH), 52.7 (CH); MS m/z (rel intensity) 314 (M+, 4), 281 (4), 270 (8), 210 (16), 197 (100). FAB HRMS (acetone/NBA/NaI) calcd for C17H14O4SNa 337.0511 (M+Na). Found 337.0514.
Oxidation of Dibenz[b,f]oxepins 1 with Mn(OAc)3 in the Presence of Acetic Anhydride. Dibenzoxepin 1 (0.1 mmol) and Mn(OAc)3•2H2O (0.107 g, 0.4 mmol) were heated under reflux in a mixture of AcOH (5 mL) and Ac2O (1.25 mL) until the brown color of Mn(III) disappeared (Table 1). The solvent was removed in vacuo and the residue was extracted with CHCl3. The combined organic layers were washed with a saturated aqueous solution of NaHCO3, water, dried over anhydrous MgSO4, and concentrated to dryness. The residue was separated on TLC (EtOAc:hexane = 4:6 v/v), affording lactone 2 in the yield shown in Table 1.
Methyl 2-Oxo-2,3,3a,12b-tetrahydrodibenzo[b,f]furo[2,3-d]oxepin-12b-carboxylate (2a): Yield (21.7 mg, 70%); colorless microcrystals (from CHCl3/hexane); mp 151-153 °C; IR (CHCl3) ν 1781, 1752 (C=O); 1HNMR (CDCl3) δ = 7.43-7.14 (8H, m, arom H), 4.50 (1H, t, J = 10.0 Hz, CH), 3.75 (3H, s, OMe), 3.01 (2H, d, J = 10.0 Hz, CH2); 13CNMR (CDCl3) δ = 173.1, 169.4 (C=O), 156.7, 155.8 (arom C-O), 131.0, 130.9, 130.2, 129.3, 129.0, 125.6, 121.7, 121.4 (arom CH), 127.6, 127.4 (arom C), 86.1 (C-12b), 53.4 (OMe), 44.4 (CH), 33.6 (CH2); MS m/z (rel intensity) 310 (M+, 12), 252 (30), 251 (100), 223 (26), 209 (88), 205 (58), 181 (70), 165 (18), 152 (20). Anal. Calcd for C18H14O5: C, 69.67; H, 4.55. Found: C, 69.64; H, 4.47.
12b-(Methoxymethyl)-3,3a-dihydrodibenzo[b,f]furo[2,3-d]oxepin-2(12bH)-one (2b): Yield (69.7 mg, 80%); colorless oil; IR (CHCl3) ν 1780 (C=O); 1HNMR (CDCl3) δ = 7.55-7.53 (1H, m, arom H), 7.31-7.12 (7H, m, arom H), 4.44 (1H, dd, J = 12.0, 8.0 Hz, -CH<), 4.07 (1H, d, J = 11.5 Hz, CH2-O), 3.90 (1H, d, J = 11.5 Hz, CH2-O), 3.43 (1H, s, OMe), 3.05 (1H, dd, J = 16.5, 8.0 Hz, CH2), 2.75 (1H, dd , J = 16.5, 12.0 Hz, CH2); 13CNMR (CDCl3) δ = 174.4 (C=O), 155.7, 155.5 (arom CO), 130.9, 130.4, 128.7, 128.2, 125.5, 125.1, 121.7, 121.5 (arom CH), 129.1, 127.7 (arom C), 88.5 (C-12b), 74.4 (CH2-O), 59.7 (OMe), 41.2 (CH), 35.5 (CH2); FAB MS (acetone/NBA) m/z (rel intensity) 297 (M+H, 28), 265 (36), 251 (82), 237 (100), 207 (35), 181 (54); FAB HRMS (acetone/NBA) calcd for C18H17O4 297.1127 (M+H). Found 297.1108.
Oxidation of Dibenz[b,f]oxepins 1 with Mn(OAc)3 in the Presence of Active Methylene Compounds. A mixture of dibenzoxepin 1 (0.4 mmol), an active methylene compound, and Mn(OAc)3•2H2O was heated under reflux in AcOH (18 mL) until the opaque dark-brown color of the solution became transparent yellow. The molar ratio of the reagents is shown in Table 2. After the work-up described above, the corresponding dihydrofuran- and butenolide-fused oxepins 4 were obtained.
Dimethyl 2-Oxo-2,12b-dihydrodibenzo[b,f]furo[2,3-d]oxepin-3,12b-dicarboxylate (4ac): Yield (81.4 mg, 56%); colorless cubes (from CHCl3/hexane); mp 176-178 °C; IR(CHCl3) ν 1778, 1767, 1730 (C=O); 1HNMR (CDCl3) δ = 7.68-7.21 (8H, m, arom H), 3.93 (3H, s, OMe), 3.68 (3H, s, OMe); 13CNMR(CDCl3) δ = 167.4, 165.5, 162.9, 159.3 (3 x C=O and C-12a), 154.4, 153.1(arom C-O), 134.7, 131.12, 131.05, 126.3, 124.9, 124.2, 122.0, 121.0 (arom CH), 127.1, 117.5, 115.6 (arom C and C-3), 86.9 (C-12b), 53.9, 53.0 (OMe); MS m/z (rel intensity) 366 (M+, 44), 322 (10), 307 (100), 279 (56), 211 (38), 163 (25). Anal. Calcd for C20H14O7: C, 65.57; H, 3.85. Found: C, 65.35; H, 3.78.
3-Ethyl 12b-Methyl 2-Oxo-2,12b-dihydrodibenzo[b,f]furo[2,3-d]oxepin-3,12b-dicarboxylate (4ad): Yield (116.0 mg, 77%); colorless brocks (from CHCl3/hexane); mp 124-126 °C; IR(CHCl3) ν 1778, 1744, 1732 (C=O); 1HNMR (CDCl3) δ = 7.70-7.20 (8H, m, arom H), 4.40 (2H, q, J = 7.0 Hz, CH2), 3.68 (3H, s, OMe), 1.35 (3H, t, J = 7.0 Hz, Me); 13CNMR(CDCl3) δ = 167.5, 165.6, 162.5, 158.5 (3 x C=O and C-12a), 154.4, 153.1 (arom C-O), 134.5, 131.2, 131.0, 126.3, 125.0, 124.2, 122.0 (arom CH), 121.0, 117.7, 116.1 (arom C and C-1), 86.9 (C-12b), 62.4 (CH2), 53.8 (OMe), 13.9 (Me); MS m/z (rel intensity) 380 (M+, 28), 322 (22), 321 (100), 293 (24), 221 (21), 163 (16). FAB HRMS (acetone/NBA) calcd for C21H17O7 381.0974 (M+H). Found 381.0958.
Methyl 3-Carbamoyl-2-oxo-2,12b-dihydrodibenzo[b,f]furo[2,3-d]oxepin-12b-carboxylate (4ae): Yield (81.9 mg, 59%); colorless microcrystals (from CHCl3); mp 205-207 °C; IR(CHCl3) ν 3460, 3281, 3209 (NH2), 1767, 1747, 1676 (C=O); 1HNMR (CDCl3) δ = 8.10-8.08 (1H, m, arom H), 7.67 (1H, s, NH2), 7.60-7.18 (7H, m, arom H), 6.20 (1H, s, NH2), 3.65 (3H, s, OMe); 13CNMR(CDCl3) δ = 170.8, 165.5, 163.8, 161.8 (3 x C=O and C-12a), 154.1, 152.7 (arom C-O), 134.9, 133.5, 131.1, 126.0, 124.9, 123.3, 121.04, 121.01 (arom CH), 125.7, 116.7, 114.6 (arom C and C-1), 86.9 (C-12b), 53.9 (OMe); MS m/z (rel intensity) 351 (M+, 44), 292 (100), 275 (18), 221 (50), 165 (23). FAB HRMS (acetone/NBA) calcd for C19H14NO6 352.0821 (M+H). Found 352.0817.
1-Acetyl-12b-(methoxymethyl)-2-methyl-3a,12b-dihydrodibenzo[b,f]furo[2,3-d]oxepin (4bb): Yield (62.8 mg, 32%); colorless needles (from CHCl3/hexane); mp 141-142 °C; IR(CHCl3) ν 1674 (C=O); 1HNMR (CDCl3) δ = 7.50-7.49 (1H, m, arom H), 7.24-7.04 (7H, m, arom H), 4.81 (1H, s, H-3a), 3.72 (1H, d, J = 9.5 Hz, CHaHb), 3.66 (1H, d, J = 9.5 Hz, CHaHb), 3.30 (3H, s, OMe), 2.41 (3H, s, Me), 2.23 (3H, s, Me); 13CNMR(CDCl3) δ = 194.9 (C=O), 167.7 (C-2), 160.0, 157.6 (arom C-O), 131.2, 129.8 (arom C), 129.7, 129.4, 129.3, 128.5, 125.1, 125.0, 121.0, 120.4 (arom CH), 114.5 (C-3), 90.0 (C-12b), 78.4 (CH2-O), 59.9 (OMe), 48.5 (C-3a), 29.4, 15.4 (Me). MS m/z (rel intensity) 336 (M+, 8), 304 (20), 291 (100), 245 (45), 205 (60). FAB HRMS (acetone/NBA) calcd for C21H21O4 337.1440 (M+H). Found 337.1453.
Methyl 12b-(Methoxymethyl)-2-oxo-2,12b-dihydrodibenzo[b,f]furo[2,3-d]oxepin-3-carboxylate (4bc): Yield (8.9 mg, 15%); IR(CHCl3) ν 1775, 1744 (C=O); 1HNMR (CDCl3) δ = 7.72-7.13 (8H, m, arom H), 3.89 (1H, d, J = 9.5 Hz, CH2), 3.88 (3H, s, OMe), 3.82 (1H, d, J = 9.5 Hz, CH2), 3.23 (3H, s, OMe); 13CNMR(CDCl3) δ = 176.3, 171.0 (C=O), 167.5 (C-3a), 162.9, 162.3 (arom C-O), 131.9, 131.5, 129.5, 126.3, 123.5, 121.4, 121.1, 121.0 (arom CH), 121.2, 120.9, 117.0 (arom C and C-3), 88.5 (C-12b), 72.8 (CH2), 59.1 (OMe), 52.7 (OMe); MS m/z (rel intensity) 352 (M+, 10), 309 (70), 276 (100), 249 (35), 205 (35), 181 (50). FAB HRMS (acetone/NBA) calcd for C20H17O6 353.1025 (M+H). Found 353.1053.
Ethyl 12b-(Methoxymethyl)-2-oxo-2,12b-dihydrodibenzo[b,f]furo[2,3-d]oxepin-3-carboxylate (4bd): Yield (8.0 mg, 17%); IR(CHCl 3) ν 1771, 1720 (C=O); 1HNMR (CDCl3) δ = 7.70-7.68 (1H, arom H), 7.59-7.52 (2H, m, arom H), 7.40-7.16 (5H, arom H), 4.35 (2H, q, J = 7.0 Hz, CH2-O), 3.90 (1H, d, J = 11.5 Hz, Ha-CH-O), 3.81 (1H, d, J = 11.5 Hz, Hb-CH-O), 3.26 (3H, s, OMe), 1.31 (3H, t, J = 7.0 Hz, Me); 13CNMR (CDCl3) δ = 162.5, 161.4, (C=O), 153.3 (2 x arom C-O), 151.7 (C-3a), 133.9, 131.6, 126.4 (2C), 125.8, 123.3, 121.4, 121.1 (arom CH), 121.5 (2 x arom C), 115.0 (C-3), 88.5 (C-12b), 72.9 (CH2), 62.0 (CH2), 60.2 (OMe), 13.9 (Me); MS m/z (rel intensity) 366 (M+, 10), 323 (50), 293 (20), 276 (100), 245 (22), 221 (25). HRMS (acetone/NBA) calcd for C21H19O6 367.1182 (M+H). Found 367.1187.
12b-(Methoxymethyl)-2-oxo-2,12b-dihydrodibenzo[b,f]furo[2,3-d]oxepin-3-carboxamide (4be): Yield (102.7 mg, 66%); yellow cubes (from CHCl3/hexane); mp 223-227 °C; IR(CHCl 3) ν 3404, 3350-3000 (NH2), 1755, 1676 (C=O); 1HNMR (CDCl3) δ = 8.00-7.90 (1H, s, NH2), 7.93-7.91 (1H, arom H), 7.64-7.18 (7H, m, arom H), 5.79 (1H, s, NH), 3.79 (1H, d, J = 11.5 Hz, Ha-CH-O), 3.76 (1H, d, J = 11.5 Hz, Hb-CH-O), 3.20 (3H, s, OMe); 13CNMR (CDCl3) δ = 171.3, 166.6, 161.8 (2 x C=O and C-12a), 153.2, 151.5 (arom C-O), 134.1, 133.7, 130.8, 126.1, 125.6, 122.7, 121.1, 120.5 (arom CH), 124.9, 116.1, 115.6 (arom C and C-1), 88.6 (C-12b), 72.9 (CH2), 60.0 (OMe); MS m/z (rel intensity) 337 (M+, 8), 305 (11), 276 (100), 221 (20), 165 (12). HRMS (acetone/NBA) calcd for C19H16NO5 338.1028 (M+H). Found 338.1013.
Approach to the Synthesis of Dibenzo[b,f]thiepincarboxylate 6. A mixture of 5 (0.15 g, 0.48 mmol) and Mn(OAc)3•2H2O (0.51 g, 1.90 mmol) was heated under reflux in AcOH (16 mL) until the brown color of the solution became transparent yellow (within 15 min). After the usual work-up, the crude products were separated on silica gel TLC developing with EtOAc/hexane (2:8 v/v), not affording the desired thiepincarboxylate 6 but yielding methyl 2-acetoxy-2-(9H-thioxanthen-9-yl)acetate (7) (97.3 mg, 63%) and thioxanthone (16.5 mg, 10%). When the reaction of 5 (0.10 g, 0.32 mmol) with Mn(OAc)3•2H2O (0.34 g, 1.27 mmol) was carried out in DMF (11 mL) for 30 min at reflux temperature, only methyl 2-(9H-thioxanthen-9-ylidene)acetate (8) (9.2 mg, 10%) and thioxanthone (5.4 mg, 8%) were isolated. A similar reaction in EtOH for 26 h gave 8 (3%) and thioxanthone (13%). The oxidation of 5 (0.20 g, 0.64 mmol) with Mn(pic)3 (1.12 g, 2.54 mmol) was conducted in DMF (21 mL) at reflux temperature for 24 h. After the usual work-up, the crude products were separated on silica gel TLC developing with CHCl3/hexane (5:5 v/v), affording methyl 2-(9H-thioxanthen-9-yl)acetate (9) (2.1 mg, 2%) and thioxanthone (31.1 mg, 18%).
Methyl 2-Acetoxy-2-(9H-thioxanthen-9-yl)acetate (7): Colorless oil; IR (CHCl3) ν 1746 (C=O); 1HNMR (CDCl3) δ = 7.44-7.20 (8H, m, arom H), 5.26 (1H, d, J = 9.0 Hz, CH), 4.50 (1H, d, J = 9.0 Hz, CH), 3.38 (3H, s, OMe), 1.96 (3H, s, AcO); 13CNMR (CDCl3) δ = 169.8, 169.7 (C=O), 133.6, 132.79, 132.76, 131.8 (arom C), 130.6, 129.3, 127.7, 127.5, 127.0, 126.9, 126.4, 126.2 (arom CH), 71.1 (CH), 51.8 (CH), 50.6 (OMe), 20.4 (Ac); MS m/z (rel intensity) 328 (M+, 15), 197 (100), 165 (45). FAB HRMS (acetone/NBA/NaI) calcd for C18H16O4SNa 351.0667 (M+Na). Found 351.0678.
Methyl 2-(9H-Thioxanthen-9-ylidene)acetate (8): Yellow oil; IR(CHCl3) ν 1717 (C=O), 1612 (C=C); 1HNMR (CDCl3) δ = 7.65-7.23 (8H, m, arom H), 6.15 (1H, s, HC=C), 3.69 (3H, s, OMe); 13CNMR (CDCl3) δ = 166.7 (C=O), 147.8 (=C<), 135.3, 133.0, 132.3, 130.7 (arom C), 130.1, 128.6, 128.2, 126.9, 126.4, 125.9, 125.6, 125.5 (arom CH), 117.7 (=CH-), 51.4 (OMe); MS m/z (rel intensity) 268 (M+, 100), 253 (45), 237 (85), 210 (60). FAB HRMS (acetone/NBA) calcd for C16H12O2S 268.0558 (M+). Found 268.0568.
Methyl 2-(9H-Thioxanthen-9-yl)acetate (9): yellow oil; IR(CHCl3) ν 1732 (C=O); 1HNMR (CDCl3) δ = 7.42-7.19 (8H, m, arom H), 4.61 (1H, t, J = 8.0 Hz), 3.54 (3H, s, OMe), 2.75 (2H, d, J = 8.0 Hz, CH2); 13CNMR (CDCl3) δ = 172.0 (C=O), 136.7, 132.6 (arom C), 128.8, 127.0, 126.9, 126.7 (arom CH), 51.5 (OMe), 45.3 (CH), 36.9 (CH2); MS m/z(rel intensity), 270 (M+, 25), 197 (100). FAB HRMS (acetone/NBA) calcd for C16H14O2S 270.0715 (M+). Found 270.0717.
ACKNOWLEDGEMENTS
This research was supported by a Grant-in-Aid for Scientific Research (C), No. 22550041, from the Japan Society for the Promotion of Science.
References
1. a) S. von Angerer, Sci. Synth., 2004, 17, 653; b) R. Olivera, R. SanMartin, F. Churruca, and E. Domínguez, Org. Prep. Proced. Int., 2004, 36, 297; CrossRef c) D. Pesic, I. O. Landek, R. Rupcic, M. Modric, I. Dzapo, R. Trojko, M. Mercep, and M. Mesic, J. Heterocycl. Chem., 2012, 49, 243; CrossRef d) T. P. Majhi, B. Achari, and P. Chattopadhyay, Heterocycles, 2007, 71, 1011. CrossRef
2. a) A. S. R. Anjaneyulu, A. V. R. Reddy, D. S. K. Reddy, R. S. Ward, D. Adhikesavalu, and T. S. Cameron, Tetrahedron, 1984, 40, 4245; CrossRef b) M. F. Comber and M. V. Sargent, J. Chem. Soc., Perkin Trans. 1, 1990, 1371. CrossRef
3. T.-X. Qian and L.-N. Li, Phytochemistry, 1992, 31, 1068. CrossRef
4. a) M.-I. Chung, H.-H. Ko, M.-H. Yen, C.-N. Lin, S.-Z. Yang, L.-T. Tsao, and J.-P. Wang, Helv. Chim. Acta, 2000, 83, 1200; CrossRef b) H.-H. Ko and C.-N. Lin, Helv. Chim. Acta, 2000, 83, 3000; CrossRef c) Y.-H. Lu, C.-N. Lin, H.-H. Ko, S.-Z. Yang, L.-T. Tsao, and J.-P. Wang, Helv. Chim. Acta, 2002, 85, 1626. CrossRef
5. P. Kittakoop, S. Nopichai, N. Thongon, P. Charoenchai, and Y. Thebtaranonth, Helv. Chem. Acta, 2004, 87, 175. CrossRef
6. G. R. Pettit, A. Numata, C. Iwamoto, Y. Usami, T. Yamada, H. Ohishi, and G. M. Cragg, J. Nat. Prod., 2006, 69, 323. CrossRef
7. L.-H. Mu, J.-B. Li, J.-Z. Yang, and D.-M. Zhang, J. Asian Nat. Prod. Res., 2007, 9, 649. CrossRef
8. a) Z. Yang, H. N. C. Wong, P. M. Hon, H. M. Chang, and C. M. Lee, J. Org. Chem., 1992, 57, 4033; CrossRef b) M. P. Paduraru and P. D. Wilson, Org. Lett., 2003, 5, 4911; CrossRef c) A. A. Trabanco, J. M. Alonso, J. I. Andrés, J. M. Cid, J. Fernández, L. Iturrino, and A. Megens, Chem. Pharm. Bull., 2004, 52, 262; CrossRef d) S. Kumar, H. Ila, and H. Junjappa, Tetrahedron, 2007, 63, 10067; CrossRef e) T. H. Jepsen, M. Larsen, M. Joergensen, and M. B. Nielsen, Synlett, 2012, 418. CrossRef
9. a) Z. Cong, T. Miki, O. Urakawa, and H. Nishino, J. Org. Chem., 2009, 74, 3978; CrossRef b) H. Nishino, H. Kamachi, H. Baba, and K. Kurosawa, J. Org. Chem., 1992, 57, 3551. CrossRef
10. a) W. E. Fristad and J. R. Peterson, J. Org. Chem., 1985, 50, 10; CrossRef b) W. E. Fristad, J. R. Peterson, and J. R. Ernst, J. Org. Chem., 1985, 50, 3143; CrossRef c) E. J. Corey and A. W. Gross, Tetrahedron Lett., 1985, 26, 4291; CrossRef d) W. E. Fristad, J. R. Peterson, J. R. Ernst, and G. B. Urbi, Tetrahedron, 1986, 42, 3429. CrossRef
11. J. B. Bush, Jr. and H. Finkbeiner, J. Am. Chem. Soc., 1968, 90, 5903. CrossRef
12. a) H. Nishino, V.-H. Nguyen, S. Yoshinaga, and K. Kurosawa, J. Org. Chem., 1996, 61, 8264; CrossRef b) Y. Ito, T. Yoshinaga, and H. Nishino, Tetrahedron, 2010, 66, 2683. CrossRef
13. Ionization potential (IP) was calculated by Spartan ’08 according to the ground state with Hartree-Fock 3-21G in vacuum.
14. a) N. Ito, H. Nishino, and K. Kurosawa, Bull. Chem. Soc. Jpn., 1983, 56, 3527; CrossRef b) N. Fujimoto, H. Nishino, and K. Kurosawa, Bull. Chem. Soc. Jpn., 1986, 59, 3161; CrossRef c) W. E. Fristad and S. S Hershberger, J. Org. Chem., 1985, 50, 1026; CrossRef d) W. E. Fristad, J. R. Peterson, and A. B. Ernst, J. Org. Chem., 1985, 50, 3143. CrossRef
15. a) E. I. Heiba and R. M. Dessau, J. Org. Chem., 1974, 39, 3456; CrossRef b) H. Nishino, Bull. Chem. Soc. Jpn., 1985, 58, 1922. CrossRef
16. H. Sato, H. Nishino, and K. Kurosawa, Bull. Chem. Soc. Jpn., 1987, 60, 1753. CrossRef
17. a) H. Nishino, Bull. Chem. Soc. Jpn., 1985, 58, 217; CrossRef b) H. Nishino, H. Hashimoto, J. D. Korp, and K. Kurosawa, Bull. Chem. Soc. Jpn., 1995, 68, 1999; CrossRef c) H. Nishino, K. Ishida, H. Hashimoto, and K. Kurosawa, Synthesis, 1996, 888. CrossRef
18. D. Acton, G. Hill, and B. S. Tait, J. Med. Chem., 1983, 26, 1131. CrossRef
19. a) Y. Tamura, C. Mukai, and M. Ikeda, Heterocycles, 1979, 12, 1179; CrossRef b) Y. Tamura, C. Mukai, N. Nakajima, and M. Ikeda, J. Chem. Soc., Perkin Trans. 1, 1981, 212. CrossRef
20. Y. Yamaguchi and D. T. Sawyer, Inorg. Chem., 1985, 24, 971. CrossRef
21. M. Tamano and J. Koketsu, Bull. Chem. Soc. Jpn., 1985, 58, 2577. CrossRef
22. Y. Tamura, C. Mukai, N. Nakajima, M. Ileda, and M. Kido, J. Chem. Soc., Perkin Trans. 1, 1981, 212. CrossRef