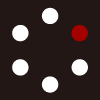
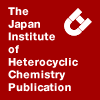
HETEROCYCLES
An International Journal for Reviews and Communications in Heterocyclic ChemistryWeb Edition ISSN: 1881-0942
Published online by The Japan Institute of Heterocyclic Chemistry
e-Journal
Full Text HTML
Received, 1st August, 2012, Accepted, 5th September, 2012, Published online, 10th September, 2012.
DOI: 10.3987/COM-12-12561
■ Structure-Activity Relationships of Biflavonoids for β-Secretase (BACE-1) Inhibitory Activity
Hiroaki Sasaki, Yuki Kitoh, Kazuhiko Miki, Kaoru Kinoshita, Kiyotaka Koyama, Miyuki Kaneda, and Kunio Takahashi*
Department of Pharmacognosy and Phytochemistry, Meiji Pharmaceutical University, 2-522-1 Noshio, Kiyose, Tokyo 204-8588, Japan
Abstract
Bioactive natural products are useful sources of new pharmaceutics. Their analogues are also important for evaluating structure-activity relationships. In this study the structure-activity relationships of 2,3-dihydro-6-methylginketin (1) for BACE-1 inhibitory activity were studied. Biflavonoids consisting of flavanone and flavone, and also the presence of a methoxy group at the C-4’ position, were found to be important for strong activity. 2,3-Dihydro-6-methylbilobetin (2) showed strong activity with an IC50 value of 0.56 μM.Biflavonoids are flavonoid dimers connected by C-C or C-O-C bonds and exhibit anti-inflammatory1 and anti-malarial2 activities. We have investigated the biological activities of biflavonoids, and found that amentoflavone-type biflavonoids showed anti-influenza3,4 and β-secretase (BACE-1: β-site APP cleaving enzyme-1) inhibitory activities.5 Amyloid β (Aβ) peptides, involved in the development of Alzheimer’s disease (AD), are generated from the cleavage of the β-amyloid precursor protein (APP) by consecutive action of BACE-1. Hence, BACE-1 has been recognized as an effective and safe therapeutic for AD. There are few compounds that function like BACE-1 inhibitors from natural resources.5-10 We found that flavanone-flavone dimeric biflavonoid, 2,3-dihydro-6-methylginkgetin (1), showed the strongest inhibitory activity among amentoflavone-type biflavonoids,5 and we later isolated new biflavonoids, 2,3-dihydro-6-methylbilobetin (2) and 2,3-dihydro-6-methylsequoiaflavone (3), from Cephalotaxus harringtonia var. fastigiata (Cephalotaxaceae).11 In this paper we report the BACE-1 inhibitory activities and structure-activity relationships of compounds 1-3 and 4 (methylation product of 1, 2,3-dihydro-6-methylamentoflavone 7,4’,7’’,4’’’-tetramethyl ether), and some flavonoids, apigenin, naringenin, and their derivatives (5-10) (Figure 1 and Scheme 1).
We have already reported strong BACE-1 inhibitory compounds, 2,3-dihydro-6-methylginkgetin (1) and 2,3-dihydroamentoflavone (1a), among amentoflavone-type biflavonoids by using the BACE-1 FRET assay kit.5 These results showed that flavanone-flavone dimeric biflavonoids (IC50, 0.35 - 0.75 µM) showed stronger BACE-1 inhibitory activities than flavone-flavone dimers (IC50, 1.54 - >10 µM). Thus, the former is more important than the latter for significant activity. Differences in the structures of 1 and 1a were the presence of 6-methyl and 7,4’-dimethoxy groups in 1 instead of 6-H and 7,4’-dihydroxy groups in 1a. The substitution of the 6-methyl group with 7 and 4’ methoxy groups on the flavanone moiety does not affect the inhibitory activity. We have also isolated new biflavonoids, 3-dihydro-6-methylbilobetin (2), 2,3-dihydro-6-sequoiaflavone (3), with 2,3-dihydro-6-methylginkgetin (1) from Cephalotaxus harringtonia var. fastigiata (Cephalotaxaceae).11 In this paper, the correlation between methoxy positions and activities were discussed among some 6-methylflavanone-flavone biflavonoids. Compound 1 was methylated by trimethylsilyldiazomethane (TMS-diazomethane) in CH2Cl2 and MeOH to afford 4 as a mixture of diastereoisomers due to the presence of a chiral center on C-2 and atropisomerism induced along the C3’-C8’’ bond. Therefore, the resultant doubling of proton and carbon resonances was observed in the spectra (Table 1).11 Since the diastereoisomers of 4 could not be separated from each other, they were used in the assay without further purification. In addition, flavonoid monomers 5, 7 and 9 and their methylated compounds 6, 8 and 10 were used and compared to biflavonoids 1-4 in the assay. As shown in Table 2, 1 and 2 showed similar strong inhibitory activities, with IC50 values of 0.44, and 0.56 µM, respectively, while 3 with an IC50 value of 3.05 µM showed lower activity than both 1 and 2. From comparing 1 and 2, the substitution of a methoxy instead of a hydroxy group at C-7 does not affect the activity. Comparing 1, 2 and 3, a methoxy instead of a hydroxy group at C-4’ increases the activity. Comparing 1 to 4, methoxy instead of hydroxy groups at both C-7’’ and 4’’’ caused the activity to disappear (IC50 values of 0.44, 0.56, 3.05 and >10 µM). Compounds 4-10 showed no activities. On the basis of these results, it was revealed that the structure of a flavanone-flavone dimer with a methoxy group at the 4’ position as in 1 and 2 is important for strong BACE-1 inhibitory activities. It was concluded that structures consisting of flavanone-flavone and a 4’-methoxy on a flavanone moiety are important basic structures for significant BACE-1 inhibitory activity.
EXPERIMENTAL
Melting points were measured with a Yanaco MP-J3 micro melting point apparatus. IR spectra were measured as KBr disks with a JASCO IR Report-100 infrared spectrometer. UV spectra were measured with a HITACHI U-1800 spectrometer. MS spectra were measured with a JEOL JMS-700 spectrometer. NMR spectra were measured with a JEOL JNM-500 FT NMR spectrometer. Circular dichroism (CD) measurements were performed under dry N2 with a JASCO-820 spectropolarimeter at 25 °C.
Materials. Compounds 1-3 were isolated from Cephalotaxus harringtonia var. fastigiata.11 Apigenin (Tokyo Chemical Industry, Japan) was used as Compound 5. Naringenin (LKT Laboratories, USA) was used as Compound 7. Hesperetin (Tokyo Chemical Industry, Japan) was used as Compound 9.
2,3-Dihydro-6-methylamentoflavone 7,4’,7’’,4’’’-tetramethyl ether (4): 2,3-Dihydro-6-methyl- ginkgetin (1) (11.0 mg, 18.9 mmol) was dissolved in CH2Cl2/MeOH (6 mL, 5:1 v/v). TMS-diazomethane as a 0.6 M solution in hexanes (2 mL) was added. After stirring at rt for 12 h, acetic acid was added. The solution was evaporated and the residue was purified by column chromatography on silica gel (CHCl3-MeOH) to give 4 (4.4 mg, 34%) as a white solid; mp 85-87 °C; IR 3500 (sh), 2990, 2920, 1770, 1750, 1690, 1670, 1570, 1540, 1490, 1410, 1160 cm-1; UV λmax (Δε) 215 (4.40), 278 (4.13), 324 (3.94) nm; CD λmax (Δε) 330 (+1.9), 290 (-5.7), 250 (+0.2), 241 (-0.3), 212 (+6.1) nm; 1H NMR and 13C NMR see Table 1; EIMS m/z 610 (M+, 100), 430 (16); HREIMS 610.1842 (M+, Calcd for C35H30O10, 610.1839).
Apigenin 7,4'-dimethyl ether (6): Apigenin (5) (50.1 mg, 185 mmol) was dissolved in CH2Cl2/MeOH (6 mL; 5:1 v/v). TMS-diazomethane as a 0.6 M solution in hexanes (2 mL) was added. After stirring at rt for 12 h, acetic acid was added. The solution was evaporated and the residue was purified by column chromatography on silica gel (CHCl3-MeOH) to give 6 (44.5 mg, 81%) as a yellow solid; mp 175-176 °C; IR 3425 (sh), 1670, 1600, 1505, 1440, 1380, 1340, 1270, 1160, 1020, 835 cm-1; UV λmax (Δε) 236 (4.48), 271 (4.37), 322 (3.24) nm; 1H NMR (500 MHz, DMSO-d6): δ 3.86 (3H, s), 3.87 (3H, s), 6.37 (1H, d, J = 2.3 Hz), 6.78 (1H, d, J = 2.3 Hz), 6.93 (1H, s), 7.11 (2H, d, J = 9.2 Hz), 8.05 (2H, d, J = 9.2 Hz), 12.91 (1H, s); 13C NMR (DMSO-d6): δ 55.6, 56.0, 92.7, 98.0, 103.7, 104.7, 114.6, 122.7, 128.4, 157.2, 161.2, 162.4, 163.6, 165.2, 182.0; EIMS m/z 298 (M+, 100), 297 (10), 269 (12), 255 (10); HREIMS 298.0840 (M+, Calcd for C17H14O5, 298.0841).
Naringenin 7,4'-dimethyl ether (8): Naringenin (7) (50.4 mg, 185 mmol) was dissolved in CH2Cl2/MeOH (6 mL; 5:1 v/v). TMS-diazomethane as a 0.6 M solution in hexanes (2 mL) was added. After stirring at rt for 12 h, acetic acid was added. The solution was evaporated and the residue was purified by column chromatography on silica gel (CHCl3-MeOH) to give 8 (42.6 mg, 77%) as a white solid; mp 117-118 °C; IR 3425 (sh), 1655, 1630, 1555, 1505, 1305, 1210, 1160, 1090, 1030, 830 cm-1; UV λmax (Δε) 237 (4.24), 290 (4.39) nm; 1H NMR (500 MHz, DMSO-d6): δ 2.76 (1H, dd, J = 17.2, 2.9 Hz), 3.34 (1H, dd, J = 17.2, 12.6 Hz), 3.77 (3H, s), 3.79 (3H, s), 5.56 (1H, dd, J = 12.6, 2.9 Hz), 6.09 (1H, d, J = 2.3 Hz), 6.12 (1H, d, J = 2.3 Hz), 6.98 (1H, d, J = 9.2 Hz), 7.45 (1H, d, J = 9.2 Hz), 12.11 (3H, s); 13C NMR (DMSO-d6): δ 42.0, 55.2, 55.9, 78.4, 93.8, 94.7, 102.6, 113.9, 128.3, 130.4, 159.5, 162.8, 163.2, 167.4, 196.8; EIMS m/z 300 (M+, 100), 299 (47), 134 (74), 121 (40); HREIMS 300.0994 (M+, Calcd for C17H16O5, 300.0998).
7,3'-Dimethoxy hesperetin (10): Hesperetin (9) (56.0 mg, 185 mmol) was dissolved in CH2Cl2/MeOH (6 mL; 5:1 v/v). TMS-diazomethane as a 0.6 M solution in hexanes (2 mL) was added. After stirring at rt for 12 h, acetic acid was added. The solution was evaporated and the residue was purified by column chromatography on silica gel (CHCl3-MeOH) to give 10 (30.5 mg, 50%) as a yellow solid; mp 136-137 °C; IR 1680, 1620, 1570, 1355, 1320, 1260, 1210, 1140, 1080, 860 cm-1; UV λmax (Δε) 238 (4.22), 288 (4.50) nm; 1H NMR (500 MHz, DMSO-d6): δ 2.76 (1H, dd, J = 17.2, 2.9 Hz), 3.39 (1H, dd, J = 17.2, 12.6 Hz), 3.77 (3H, s), 3.78 (3H, s), 3.79 (3H, s), 5.53 (1H, dd, J = 12.6, 2.9 Hz), 6.09 (1H, d, J = 1.7 Hz), 6.14 (1H, d, J = 1.7 Hz), 6.98 (1H, d, J = 8.0 Hz), 7.04 (1H, dd, J = 8.0, 1.7 Hz), 7.15 (1H, d, J = 1.7 Hz), 12.12 (1H, s); 13C NMR (DMSO-d6): δ 42.1, 55.5, 55.6, 55.9, 78.7, 93.8, 94.7, 102.6, 110.6, 111.5, 119.3, 130.8, 148.7, 149.1, 162.8, 163.2, 167.4, 196.8; EIMS m/z 330 (M+, 100), 329 (26), 164 (63), 151 (76); HREIMS 330.1096 (M+, Calcd for C18H18O6, 330.1103).
BACE-1 Assay Method. BACE-1 assays were performed on black 384-well plates using a BACE-1 FRET Assay kit (Invitrogen Co., USA). The assay was carried out according to the supplied manual with modifications.5 Samples were dissolved in the assay buffer (50 mM sodium acetate, pH 4.5) with DMSO (final concentrations were 10%). 10 µL of test samples, 10 µL of BACE-1 substrate (750 nM Rh-EVNLDAEFK-Quencher, in 50 mM ammonium bicarbonate), and 10 µL of BACE-1 enzyme (1.0 U/mL) were mixed in the wells, and incubated 60 min in the dark at 25 °C. The fluorescence intensities of the mixtures were measured by Fluoroskan Ascent (Thermo Scientific) for excitation at 544 nm and emission at 590 nm. The inhibition ratio was calculated by the following equation: inhibition (%) = [1-{(S-S0)-(B-B0)/(C-C0)-(B-B0)}]×100, where C was the fluorescence of a control [enzyme, substrate, and assay buffer concentration with DMSO (final concentrations were 10%)] after 60 min of incubation, C0 was the initial fluorescence of a control [enzyme, substrate, and assay buffer concentration with DMSO (final concentrations were 10%)], B was the fluorescence of a control [substrate and assay buffer concentration with DMSO (final concentrations were 10%)] after 60 min of incubation, B0 was the initial fluorescence of a control [substrate and assay buffer concentration with DMSO (final concentrations were 10%)], S was the fluorescence of the tested samples (enzyme, sample solution, and substrate) after 60 min of incubation, and S0 was the initial fluorescence of the tested samples (enzyme, sample solution, and substrate). To check the quenching effect of the tested samples, the sample solution was added to reaction mixture C, and any reduction in fluorescence by the sample was investigated. β-Secretase Inhibitor (Wako, Japan) was used as a positive control.
ACKNOWLEDGEMENT
This work was supported in part by a grant from the High-Tech Research Center Project, the Ministry of Education, Culture, Sports, Science and Technology (MEXT), Japan (No. S0801043).
References
1. W.-J. Kwak, C. K. Han, K. H. Son, H. W. Chang, S. S. Kang, B. K. Park, and H. P. Kim, Planta Med., 2002, 68, 316. CrossRef
2. C. Ichino, H. Kiyohara, N. Soonthornchareonnon, W. Chuakul, A. Ishiyama, H. Sekiguchi, M. Namatame, K. Otoguro, S. Omura, and H. Yamada, Planta Med., 2006, 72, 611. CrossRef
3. K. Miki, T. Nagai, K. Suzuki, R. Tsujimura, K. Koyama, K. Kinoshita, K. Furuhata, H. Yamada, and K. Takahashi, Bioorg. Med. Chem. Lett., 2007, 17, 772. CrossRef
4. K. Miki, T. Nagai, T. Nakamura, M. Tuji, K. Koyama, K. Kinoshita, K. Furuhata, H. Yamada, and K. Takahashi, Heterocycles, 2008, 75, 879. CrossRef
5. H. Sasaki, K. Miki, K. Kinoshita, K. Koyama, L. D. Juliawaty, S. A. Achmad, E. H. Hakim, M. Kaneda, and K. Takahashi, Bioorg. Med. Chem. Lett., 2010, 20, 4558. CrossRef
6. S. Y. Jeon, K. H. Bae, Y. H. Seong, and K. S. Song, Bioorg. Med. Chem. Lett., 2003, 13, 3905. CrossRef
7. E. M. Hwang, Y. B. Ryu, H. Y. Kim, D.-G. Kim, S.-G. Hong, J. H. Lee, M. J. Curtis-Long, S. H. Jeong, J.-Y. Park, and K. H. Park, Bioorg. Med. Chem., 2008, 16, 6669. CrossRef
8. S. Marumoto and M. Miyazawa, Phytother. Res., 2010, 24, 510.
9. J. K. Cho, Y. B. Ryu, M. J. Curtis-Long, J. Y. Kim, D. Kim, S. Lee, W. S. Lee, and K. H. Park, Bioorg. Med. Chem. Lett., 2011, 21, 2945. CrossRef
10. S. Marumoto and M. Miyazawa, Bioorg. Med. Chem., 2012, 20, 784. CrossRef
11. H. Sasaki, K. Miki, K. Koyama, K. Kinoshita, and K. Takahashi, Heterocycles, 2008, 75, 939. CrossRef