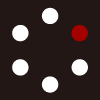
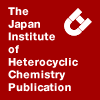
HETEROCYCLES
An International Journal for Reviews and Communications in Heterocyclic ChemistryWeb Edition ISSN: 1881-0942
Published online by The Japan Institute of Heterocyclic Chemistry
e-Journal
Full Text HTML
Received, 7th August, 2012, Accepted, 12th September, 2012, Published online, 20th September, 2012.
DOI: 10.3987/COM-12-12566
■ Novel Synthesis of Some New Fluorescent 2-Amino-3-cyanopyridines
Afsaneh Zonouzi,* Zakieh Izakian, and Seik Weng Ng
School of Chemistry, College of Science, University of Tehran, Tehran 14155-6455, Iran
Abstract
Novel synthesis of some new fluorescent 2-amino-3-cyanopyridines 2a-m in the presence of N-hydroxybenzamide or p-toluenesulfonic acid is described. Photophysical data including λAbs. and λFlu. of 2a-m in CH2Cl2, MeCN and MeOH have been measured.INTRODUCTION
Aminopyridine derivatives have attracted more attention due to their biological activities, such as cardioprotective,1 antibacterial,2 antioxidant,3 anti-inflammatory4 and anti-HIV-1.5 For example sulfapyridine which contains 2-aminopyridine moiety is an old marked antibacterial drug. Synthesis of aminopyridine derivatives with cyano substitution is rather new. In 2005 Shi and coworkers have reported the synthesis of 2-amino-3-cyanopyridine derivatives using one-pot reaction of aromatic aldehydes, ketones and malononitrile in ammonium acetate under microwave irradiation.6 In 2009 Sarda and coworkers have reported 2-amino-4,6-diphenylpyridine-3-carbonitrile through condensation of chalcones with malononitrile and ammonium acetate by ionic liquid ethylammonium nitrate as a catalyst.7 In 2011, Tang and coworkers have used ytterbium perfluoroacetate as catalyst for the synthesis of similar 2-amino- 4,6-diaryl-3-cyanopyridines in a similar manner.8 Synthesis of heteroaromatic rings contain amino substitution is still desired9 and in continuation of our quest for developing one-pot synthetic procedure for heterocyclic frameworks,10-13 herein we wish to report the one-pot condensation of 2,4-pentanedione (acetylacetone), malononitrile and some different amines in acidic media at room temperature. In this procedure non hydrated p-toluenesulfonic acid (P-TSA) and N-hydroxybenzamide (N-HB) applied as catalysts and new compounds 2a-m produced in fairly high yields (Scheme 1).
RESULTS AND DISCUSSION
In this procedure primary and secondary amines, benzyl- and phenylethylamine, cyclic amines such as pyrrolidine, piperidine and morpholine also ethanolamine can be used. These amines could reacted a little different. In the similar condition, primary amines reacted faster (5-8 h), secondary amines reacted relatively slower (8-9 h) and cyclic amines reacted even slower (9-12 h). Ethanolamine reacted more slowly (15 h) probably as a result of hydroxyl group (Table 1). It has been already reported which P-TSA and N-HB can work as Brønsted acid.14 Using N-hydroxybenzamide in CH2Cl2 catalyzed this reaction more efficient (shorter times and more yields). P-TSA in CH2Cl2 on the other hand was not as well as the other one. The results are compared in Table 1.
Structures 2a-m were assigned on the basis of their elemental analysis, IR, 1H, 13C NMR, and mass spectral data. The mass spectra of these compounds displayed molecular ion peaks at the appropriate m/z values. IR spectra of compounds 2a-m have shown a single sharp band at 2198-2215 cm-1 for C≡N group. The 1H, 13C NMR spectra of 2a-m displayed resonances in agreement with their structure. In the1H NMR of compounds 2a-i there are single N-H absorption at δ 5.03-5.58 ppm and for all of compounds there is a distinguished single peak at δ 6.34-6.60 ppm for aromatic C-H protons (Experimental Section). Single crystals of 2h were obtained from a mixture of EtOAc and n-hexane and its X-ray crystal structure was performed to confirm unambiguously the proposed structure.15 X-Ray crystal analysis have shown a monoclinic crystal structure with space group= p 21/c, R=0.635, wR2=0.1760 and Z=4 for compound 2h. Other crystallographic data can be obtained as supplementary publication No. CCDC 891215 (Figure 1).
A possible mechanism for the formation of products 2 is shown in Scheme 2. Acetylacetone and malononitrile were initially condensed to produce adduct 3. Nucleophilic addition of amine to one of the CN groups of 3, cyclization followed by elimination of H2O in acidic media converted intermediate 3 to the final product 2 through the cyclic structure 4. Adduct 3 could be recognized as an intermediate in IR spectra of reaction mixture. Two sharp absorptions for CN groups (2054-2209 cm-1) and a sharp absorption for carbonyl group (1738-1740 cm-1) in the IR spectra of reaction mixture were evidences for 3 which were good for monitoring the progress of reactions. So, in the last stage of reactions the CO and one of the CN absorptions were highly decreased and in the IR spectra of final products only one of the CN absorptions (2198-2209 cm-1) remained. Our attempt to isolate 3 was not successful. It seems that stability gained from aromatization led to rapid transformation of intermediate 3 to final product.
Optoelectronic devices such as optical fiber switchers, tunable lasers and amplifiers, modulators with various applications need compounds emitting in blue spectral region,16-18 there are some reports on using the fluorescent compounds in biochemical and medical research.19 On the other hand 2-aminopyridine has already being used as one of the fluorescence quantum yield standards,20 so developing a procedure for the synthesis of thermally stable, highly fluorescent materials emitting in blue spectral region can be urgently interesting for technology upgrading and biochemical researches. Figure 2 shows photograph of compound 2e solutions to represent the fluorescence features of the synthesized compounds.
The photophysical data of compounds 2a-m including λAbs. (nm) and λFlu. (nm) have been measured for 2×10-5 M solution in CH2Cl2, MeCN and MeOH. The maximum absorption and emission wavelengths of 2a-m in different solvents have been in the range of 285-300 nm and 366-413 nm respectively. The results are shown in Table 2.
CONCLUSION
In summary, we have reported the novel acid catalyzed condensation of acetylacetone, malononitrile and different amines by a one-pot multi component reaction. Fluorescent 2-amino-3-cyanopyridine derivatives 2a-m are synthesized in fairly high yields. High atom economy, easy procedure and high yields are the other advantages of this method. Photophysical data of compounds 2a-m including λAbs. and λFlu. in CH2Cl2, MeCN and MeOH are measured. Further investigation of this method is currently in progress to establish its scope and utility.
EXPERIMENTAL
Chemicals and solvents were obtained from Merck (Germany) and Fluka (Switzerland). Melting points were measured on Barnstead Electrothermal melting point apparatus and are not corrected. Elemental analyses for C, H and N were performed using a Thermo Finnigan Flash EA1112 instrument. IR spectra were measured on a Bruker EQUINOX 55 spectrophotometer as ATR method. 1H NMR and 13C NMR spectra were determined on a Bruker at 500 MHz and 128 MHz. Mass spectra were recorded on a Finnigan-MAT 8430 spectrometer at an ionization potential of 70 ev. All fluorescence intensity measurements were made with a Carry Eclipse Fluorescence spectrophotometer. The photo is taken under lamp λ=366 nm TL8W/08F8T5/BLC Philips made in Holland.
General procedure for the synthesis of compounds 2
To a stirred mixture of acetylacetone (2 mmol, 0.2 mL), malononitrile (2 mmol, 0.132 g), amine 1 (2 mmol) in CH2Cl2 (5 mL) was added N-hydroxybenzamide (50 mol %, 0.137 g) or p-toluenesulfonic acid (50 mol%, 0.172 g). The solution was stirred at room temperature for 5-15 h for N-hydroxybenzamide or 12-28 h for p-toluenesulfonic acid. The reaction was monitored by IR. When one of the CN and the CO absorptions were disappeared the solvent was removed under reduced pressure and the product was purified using column chromatography (silica gel, EtOAc/n-hexane: 1/4). The colorless crystals of product (except 2i) were obtained by recrystalization from EtOAc.
4,6-Dimethyl-2-(methylamino)pyridine-3-carbonitrile (2a):
Colorless crystals; mp 126 °C; νmax (KBr) 3384 (N-H), 2204 (C≡N), 1570, 1517, 1467 (C-N) cm-1; 1H NMR (CDCl3, 500 MHz): δH 2.36, 2.40 (6H, 2s, 2CH3), 3.06 (3H, d, J=4.86 Hz, CH3), 5.15 (1H, br s, NH), 6.35 (1H, s, C-H of pyridine) ppm. 13C NMR (CDCl3, 125 MHz): δH 20.57, 25.36 (2CH3), 28.71 (CH3-N), 89.38 (C≡N), 113.51 (C-H of pyridine), 117.37, 152.62, 159.73, 162.15 (pyridine carbons) ppm; MS: m/z: 161 (M+), 131 (M+- CH3NH), 104 (C7H7N+), 90 (C6H4N+); Anal. Calcd for C9H11N3: C, 67.06; H, 6.88; N, 26.07. Found: C, 67.12; H, 6.84; N, 26.12.
2-(Ethylamino)-4,6-dimethylpyridine-3-carbonitrile (2b):
Colorless crystals; mp 99 °C; νmax (KBr) 3360 (N-H), 2207 (C≡N), 1571, 1528 (C-N) cm-1; 1H NMR (CDCl3, 500 MHz): δH 1.26 (3H, t, J=7.2 Hz, CH3), 2.35, 2.38 (6H, 2s, 2CH3), 3.51 (2H, q of d, J=14.18 Hz, 5.62 Hz, CH2), 5.03 (1H, br s, NH), 6.34 (1H, s, C-H of pyridine) ppm. 13C NMR (CDCl3, 125 MHz): δC 15.34 (CH3 of Et), 20.58, 25.37 (2CH3), 36.64 (CH2), 89.17 (C≡N), 113.46 (C-H of pyridine), 117.39, 152.60, 159.13, 162.15 (pyridine carbons) ppm; MS: m/z: 175 (M+), 160 (M+-CH3), 146 (M+-C2H5), 131 (M+-C2H5N), 57 (C3H7N+); Anal. Calcd for C10H13N3: C, 68.54; H, 7.48; N, 23.98. Found: C, 68.50; H, 7.51; N, 23.99.
4,6-Dimethyl-2-(propylamino)pyridine-3-carbonitrile (2c):
Colorless crystals; mp 69 °C; νmax (KBr) 3375 (N-H), 2209 (C≡N), 1568, 1524 (C-N), cm-1; 1H NMR (CDCl3, 500 MHz): δH 1.00 (3H, t, J=7.45 Hz, CH3), 1.63-1.71 (2H, m, CH2), 2.37, 2.40 (6H, 2s, 2CH3), 3.47-3.51 (2H, d of t, J=14.17 Hz, J=5.57 Hz, CH2-N), 5.07 (1H, br s, NH), 6.35 (1H, s, C-H of pyridine) ppm. 13C NMR (CDCl3, 125 MHz): δC 11.84 (CH3 of propyl.), 20.61, 25.40 (2CH3 of pyridine), 23.27 (CH2), 43.55 (CH2-N), 89.15 (C≡N), 113.46 (C-H of pyridine), 117.44, 152.63, 159.28, 162.18 (pyridine carbons) ppm; MS: m/z: 189 (M+), 174 (M+-CH3), 160 (M+-C2H5), 132 (M+-C3H7N), 106 (M+-C4H7N2), 91 (C6H5N+), 57; Anal. Calcd for C11H15N3: C, 69.81; H, 7.99; N, 22.20. Found: C, 69.78; H, 7.94; N, 22.25.
2-(Isopropylamino)-4,6-dimethylpyridine-3-carbonitrile (2d):
Colorless crystals; mp 68 °C, νmax (KBr) 3356 (N-H), 2207 (C≡N), 1585, 1525 (C-N) cm-1; 1H NMR (CDCl3, 500 MHz): δH 1.23-1.32 (6H, q of d, J=22.42 Hz, J=5.57 Hz, 2CH3 of i-propyl), 2.36, 2.40 (6H, 2s, 2CH3), 4.35-4.42 (1H, m, CH), 4.85 (1H, br s, NH), 6.34 (1H, s, C-H of pyridine) ppm. 13C NMR (CDCl3, 125 MHz): δC 20.63, 25.43 (2CH3 of pyridine), 23.32 (2CH3 of i-propyl), 43.14 (CH), 89.11 (C≡N), 113.30 (C-H of pyridine), 117.48, 152.63, 158.59, 162.20 (pyridine carbons) ppm; MS: m/z: 189 (M+), 174 (M+-CH3), 132 (M+-C3H7N), 91 (C6H5N+), 57, 43; Anal. Calcd for C11H15N3: C, 69.81; H, 7.99; N, 22.20. Found: C, 69.77; H, 7.97; N, 22.26.
2-(Butylamino)-4,6-dimethylpyridine-3-carbonitrile (2e):
Colorless crystals; mp 65 °C; νmax (KBr) 3362 (N-H), 2208 (C≡N), 1570, 1525 (C-N), cm-1; 1H NMR (CDCl3, 500 MHz): δH 0.98 (3H, t, J=7.36 Hz, CH3 of butyl), 1.40-1.48 (2H, m, CH2 of butyl), 1.60-1.65 (2H, m, CH2 of butyl), 2.36, 2.43 (6H, 2s, 2CH3), 3.50-3.54 (2H, d of t, J=14.10 Hz, J=5.59 Hz, CH2-N), 5.04 (1H, br s, NH), 6.34 (1H, s, C-H of pyridine) ppm. 13C NMR (CDCl3, 125 MHz): δC 14.25 (CH3), 20.49 (CH2), 20.59, 25.39 (2CH3 of pyridine), 32.17 (CH2), 41.48 (CH2-N), 89.12 (C≡N), 113.41 (C-H of pyridine), 117.42, 152.60, 159.27, 162.16 (pyridine carbons) ppm; MS: m/z: 203 (M+), 188 (M+-CH3), 174 (M+-C2H5), 131 (M+-C4H10N), 91 (C6H5N+), 57, 43; Anal. Calcd for C12H17N3: C, 70.90; H, 8.43; N, 20.67. Found: C, 70.86; H, 8.42; N, 20.70.
2-(Isobutylamino)-4,6-dimethylpyridine-3-carbonitrile (2f):
Colorless crystals; mp 74 °C; νmax (KBr) 3369 (N-H), 2208 (C≡N), 1574, 1535 (C-N), cm-1; 1H NMR (CDCl3, 500 MHz): δH 1.00, 1.02 (6H, 2d, J=1.64 Hz, 2CH3), 1.90-1.95 (1H, m, CH), 2.36, 2.39 (6H, 2s, 2CH3), 3.34-3.37 (2H, m, CH2), 5.12 (1H, br s, NH), 6.34 (1H, s, C-H of pyridine) ppm. 13C NMR (CDCl3, 125 MHz): δC 20.59, 25.39 (2CH3 of pyridine), 20.62 (2CH3 of i-butyl), 28.84 (CH2), 49.15 (CH-N), 89.10 (C≡N), 113.43 (C-H of pyridine), 117.42, 152.60, 159.40, 162.13 (pyridine carbons) ppm; MS: m/z: 203 (M+), 188 (M+-CH3), 160 (M+-C3H7), 131 (M+-C4H10N), 105 (M+-C5H10N2), 57, 43; Anal. Calcd for C12H17N3: C, 70.90; H, 8.43; N, 20.67. Found: C, 70.93; H, 8.47; N, 20.71.
2-(2-Hydroxyethylamino)-4,6-dimethylpyridine-3-carbonitrile (2g):
Colorless crystals; mp 75 °C; νmax (KBr) 3356 (N-H), 3465-3265 (O-H), 2209 (C≡N), 1568, 1536 (C-N), 1243 (C-O) cm-1; 1H NMR (CDCl3, 500 MHz): δH 2.41-2.45 (6H, 2s, 2CH3), 3.68-3.71 (2H, d of t, J=9.38 Hz, J=5.42 Hz, CH2-NH), 3.84-3.86 (2H, t, J=4.69 Hz, CH2-OH), 4.75 (1H, br s, OH), 5.58 (1H, br s, NH), 6.44 (1H, s, C-H of pyridine) ppm. 13C NMR (CDCl3, 125 MHz): δC 20.74, 24.92 (2CH3), 45.84 (CH2-NH), 64.62 (CH2-OH), 90.18 (C≡N), 114.40 (C-H of pyridine), 116.82, 153.70, 159.58, 161.49 (pyridine carbons) ppm; MS: m/z: 190 (M+-1), 173 (M+-H2O), 141 (M+-H2O, C2H4N+), 131 (M+-C2H6NO), 103 (M+-C3H6N2O), 91 (C6H5N+); Anal. Calcd for C10H13N3O: C, 62.81; H, 6.85; N, 21.97. Found: C, 62.84; H, 6.89; N, 22.04.
2-(Benzylamino)-4,6-dimethylpyridine-3-carbonitrile (2h):
Colorless crystals; mp 138 °C; νmax (KBr) 3392 (N-H), 2198 (C≡N), 1574, 1522 (C-N) cm-1; 1H NMR (CDCl3, 500 MHz): δH 2.40, 2.43 (6H, 2s, 2CH3), 4.75 (2H, d, J=5.56 Hz, CH2), 5.42 (1H, br s, NH), 6.42 (1H, s, C-H of pyridine), 7.29-7.33, 7.37-7.41 (5H, 2m, phenyl protons) ppm. 13C NMR (CDCl3, 125 MHz): δC 20.65, 25.39 (2CH3), 45.71(CH2), 89.48 (C≡N), 114.08 (C-H of pyridine), 127.82, 128.30, 129.05, 139.42 (phenyl carbons), 117.23, 152.84, 158.90, 162.22 (pyridine carbons) ppm; MS: m/z: 237 (M+), 222 (M+-CH3), 146 (M+-C7H7), 131 (M+-PhCH2N), 107 (PhCH2N+), 91, 77; Anal. Calcd for C15H15N3: C, 75.92; H, 6.37; N, 17.71. Found: C, 75.90; H, 6.40; N, 17.74.
4,6-Dimethyl-2-(phenethylamino)pyridine-3-carbonitrile (2i):
Colorless crystals; mp 106 °C; νmax (KBr) 3368 (N-H), 2208 (C≡N), 1566, 1518 (C-N) cm-1; 1H NMR (CDCl3, 500 MHz): δH 2.38, 2.42 (6H, 2s, 2CH3), 2.94-2.97 (2H, t, J=7.13 Hz, CH2), 3.77-3.81 (2H, d of t, J=13.59 Hz, J=5.97 Hz, CH2-N), 5.15 (1H, br s, NH), 6.38 (1H, s, C-H of pyridine), 7.26-7.30, 7.35-7.38 (5H, 2m, phenyl protons) ppm. 13C NMR (CDCl3, 125 MHz): δC 20.63, 25.42 (2CH3 of pyridine), 36.28 (Ph-CH2), 43.18 (CH2-NH), 89.48 (C≡N), 113.74 (C-H of pyridine), 126.90, 129.05, 129.24, 139.58 (phenyl carbons), 117.18, 152.70, 159.02, 162.17 (pyridine carbons) ppm; MS: m/z: 251 (M+), 160 (M+-PhCH2), 131 (M+-C8H10N), 120 (C8H10N+), 91 (C6H5N+), 57, 43; Anal. Calcd for C16H17N3: C, 76.46; H, 6.82; N, 16.72. Found: C, 76.49; H, 6.84; N, 16.77.
2-(Dimethylamino)-4,6-dimethylpyridine-3-carbonitrile (2j):
Colorless crystals; mp 48 °C; νmax (KBr) 2198 (C≡N), 1581, 1557, 1513 (C-N) cm-1; 1H NMR (CDCl3, 500 MHz): δH 2.40, 2.42 (6H, 2s, 2CH3), 3.27 (6H, s, 2CH3-N), 6.44 (1H, s, C-H of pyridine) ppm. 13C NMR (CDCl3, 125 MHz): δC 21.13, 25.20 (2CH3 of pyridine), 40.97 (2CH3-N), 89.75 (C≡N), 114.33 (C-H of pyridine), 118.82, 154.85, 160.92, 161.10 (pyridine carbons) ppm; MS: m/z: 175 (M+), 160 (M+-CH3), 131 (M+-C2H6N), 105 (C7H7N+), 91 (C6H5N+); Anal. Calcd for C10H13N3: C, 68.54; H, 7.48; N, 23.98. Found: C, 68.56; H, 7.44; N, 24.03.
4,6-Dimethyl-2-(pyrrolidin-1-yl)pyridine-3-carbonitrile (2k):
Colorless crystals; mp 46 °C; νmax (KBr) 2201 (C≡N), 1555, 1554 (C-N) cm-1; 1H NMR (CDCl3, 500 MHz): δH 1.96-1.99 (4H, m, 2CH2 of pyrrolidine), 2.36, 2.39 (6H, 2s, 2CH3), 3.77-3.80 (4H, t, J=4.44 Hz, 2CH2-N of pyrrolidine), 6.35 (1H, s, C-H of pyridine) ppm. 13C NMR (CDCl3, 125 MHz): δC 21.15, 25.25 (2CH3 of pyridine), 25.99 (2CH3), 49.39 (CH2 of pyrrolidine), 88.10 (C≡N), 113.29 (C-H of pyridine), 119.34, 154.40, 157.95, 161.46 (pyridine carbons) ppm; MS: m/z: 203 (M+), 188 (M+-CH3), 131 (M+-C4H10N), 116 (M+-C4H10N, CH3), 105 (C7H7N+), 43; Anal. Calcd for C12H17N3: C, 70.90; H, 8.43; N, 20.67. Found: C, 70.94; H, 8.46; N, 20.71.
4,6-Dimethyl-2-(piperidin-1-yl)pyridine-3-carbonitrile (2l):
Yellow liquid; IR (KBr) 2209 (C≡N), 1579, 1556 (C-N) cm-1; 1H NMR (CDCl3, 500 MHz): δH 1.65-1.74 (6H, m, 3CH2), 2.39, 2.41 (6H, 2s, 2CH3), 3.61-3.63 (4H, t, J=5.59 Hz, 2CH2-N), 6.49 (1H, s, C-H of pyridine) ppm. 13C NMR (CDCl3, 125 MHz): δC 20.97, 25.05, 25.20, 26.38 (2CH3, 2CH2 of piperidine, 2CH2-N of piperidine) 50.24 (2CH2-N), 93.20 (C≡N), 115.49 (C-H of pyridine), 118.11, 152.30, 160.97, 162.54 (pyridine carbons) ppm; MS: m/z: 215 (M+), 200 (M+-CH3), 131 (M+-C5H10N), 105 (C7H7N+), 84 (C5H10N+), 91 (C6H5N+); Anal. Calcd for C13H17N3: C, 72.52; H, 7.96; N, 19.52. Found: C, 72.48; H, 7.91; N, 19.56.
4,6-Dimethyl-2-morpholinopyridine-3-carbonitrile (2m):
Colorless crystals; mp 100 °C; νmax (KBr) 2209 (C≡N), 1557, 1580 (C-N), 1148, 1066 (C-O) cm-1; 1H NMR (CDCl3, 500 MHz): δH 2.44, 2.46 (6H, 2s, 2CH3), 3.67-3.69, (4H, 2t, J=4.59 Hz, CH2 of morpholine), 3.86-3.88 (4H, 2t, J=4.89 Hz, CH2 of morpholine), 6.60 (1H, s, C-H of pyridine) ppm. 13C NMR (CDCl3, 125 MHz): δC 20.94, 25.15 (2CH3 of pyridine), 49.36, 67.26 (CH2 of morpholine), 93.94 (C≡N), 116.62 (C-H of pyridine), 117.76, 154.52, 161.21, 162.10 (pyridine carbons) ppm; MS: m/z: 217 (M+), 202 (M+-CH3), 131 (M+-C4H8NO), 105 (C7H7N+), 91 (C6H5N+), 30; Anal. Calcd for C12H15N3O: C, 66.34; H, 6.98; N, 19.34. Found: C, 66.39; H, 7.01; N, 19.39.
ACKNOWLEDGEMENTS
The support of this study by the Research Council at the University of Tehran is gratefully acknowledged.
References
1. J. Stoltefuss, S. Goldmann, A. Straub, H. Boshagen, M. Bechem, R. Gross, S. Hebisch, J. Hutter, and H. P. Rounding, 1995, US Patent 5432282 (Chem. Abstr., 1975, 122, p31344g).
2. G. D. Henry, Tetrahedron, 2004, 60, 6043. CrossRef
3. M. R. P. Queiroz, I. C. F. R. Ferreira, R. C. Calhelha, and L. M. Estevinho, Bioorg. Med. Chem., 2007, 15, 1788. CrossRef
4. F. Manna, F. Chimenti, A. Bolasco, B. Bizzarri, W. Filippelli, A. Filippelli, and L. Gagliardi, Eur. J. Med. Chem., 1999, 34, 245. CrossRef
5. J. Deng, T. Sanchez, L. Q. Al-Mawsawi, R. Dayam, R. A. Yunes, A. Garofalo, M. B. Bolger, and N. Neamati, Bioorg. Med. Chem., 2007, 15, 4985. CrossRef
6. F. Shi, S. Tu, F. Fang, and T. Li, ARKIVOC, 2005, i, 137.
7. S. R. Sarda, J. D. Kale, S. K. Wasmatkar, V. S. Kadam, P. G. Ingole, W. N. Jadhav, and R. P. Pawar, Mol. Divers., 2009, 13, 545. CrossRef
8. J. Tang, L. Wang, Y. Yao, L. Zhang, and W. Wang, Tetrahedron Lett., 2011, 52, 509. CrossRef
9. Q.-Z. Chen, Z.-C. Ding, Y.-L. Ma, Z.-D. Wang, and Z.-P. Zhan, Heterocycles, 2012, 85, 1891. CrossRef
10. A. Zonouzi, R. Mirzazadeh, A. Peivandi, and S. Dehdari, Heterocycles, 2012, 85, 1447. CrossRef
11. A. Zonouzi, H. Rahmani, A. Kamali, and M. Biniaz, Org. Prep. Proced. Int., 2011, 43, 276. CrossRef
12. A. Zonouzi, R. Mirzazadeh, M. Talebi, R. Jafarypoor, A. Peivandi, and S. W. Ng, Heterocycles, 2010, 81, 2131. CrossRef
13. A. Zonouzi, M. Biniaz, R. Mirzazadeh, M. Talebi, and S. W. Ng, Heterocycles, 2010, 81, 1271. CrossRef
14. E. Bartmess, Patai's Chemistry of Functional Groups, The Brønsted Acid/Base Character of Hydroxylamines, Oximes and Hydroxamic Acids, John Wiley & Sons. Inc., 2010, pp. 20-22.
15. Crystallographic data for the structure of compound 2h reported in this paper have been deposited with the Cambridge Crystallographie Data Center as supplementary publication No. CCDC 891215. These data can be obtained free of charge via www.ccdc.com.ac.uk/ data_request/cif.
16. H. Kanbara, M. Asobe, K. Kubodera, T. Kaino, and T. Kurihara, Appl. Phys. Lett., 1992, 61, 2290. CrossRef
17. F. J. Duarte, Appl. Opt., 1994, 33, 3857. CrossRef
18. C. W. Tang and S. A. VanSlyke, Appl .Phys. Lett., 1987, 51, 913. CrossRef
19. J. R. Lakowicz, Principles of Fluorescence Spectroscopy. Springer, New York, 2006, p. 63. CrossRef
20. D. F. Eaton, Pure Appl. Chem., 1988, 60, 1107. CrossRef