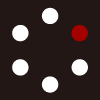
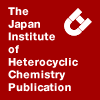
HETEROCYCLES
An International Journal for Reviews and Communications in Heterocyclic ChemistryWeb Edition ISSN: 1881-0942
Published online by The Japan Institute of Heterocyclic Chemistry
e-Journal
Full Text HTML
Received, 16th August, 2012, Accepted, 18th September, 2012, Published online, 26th September, 2012.
DOI: 10.3987/COM-12-12568
■ Oximes of 3-Hydroxyprenylflavanones and Their Cytotoxic Activities
Sanit Thongnest, Ranu Sawangsri, Korakot Navakhun, Jantana Yahuafai, Pongpun Siripong, and Somyote Sutthivaiyakit*
Department of Chemistry and Center of Excellence for Innovation in Chemistry, Faculty of Science, Ramkhamhaeng University, Ramkhamhaeng Road, Bangkapi, Bangkok 10240, Thailand
Abstract
Oximination of 3-hydroxyprenylflavanones using hydroxylamine hydrochloride yielded 4-hydroxy-3-oxime and 3-hydroxy-4-oxime derivatives. The 3β-O-acetylhydroxyprenylflavanones, under similar reaction condition, yielded corresponding 4-O-acetyl-3-oximes. The configurations at C-4 of the 4-hydroxy-3-oximes obtained from both 3α- and 3β-hydroxyprenylflavanones and of 4-O-acetyl-3-oxime derivative from 3β-O-acetylprenylflavanones were proposed. Cytotoxic activities of the oximes and their parent compounds were evaluated against a panel of human cancer cell lines.INTRODUCTION
In the course of our search for bioactive compounds from Eriosema chinense we were able to obtain a few 3-hydroxyprenylflavanones which showed strong cytotoxic activity1 and since several oximes of flavonoids showed higher potency than the parent flavonoids2 and as there has been limited report on the preparation and biological activities of oximes of 3-hydroxyflavanones,3 we herein report the formation and characterization of the oximes from the reaction between 3-hydroxyprenylflavanones (1-3) and NH2OH·HCl, in addition to their cytotoxic activity.
RESULTS AND DISCUSSION
In this study we found that reaction of 3β-hydroxyprenylflavanone 1, khonklonginol A,1 with NH2OH·HCl gave two oxime products, 4β-hydroxy-3-oxime (4, in 28% isolated yield) and 3β-hydroxy- 4-oxime (5, in 6.5% isolated yield), whereas reaction of 3α-hydroxyprenylflavanone (khonklonginol B, 2) with NH2OH·HCl gave 4 as the only product (in 62% isolated yield), as detected identical by 1H and 13C NMR spectra and specific rotations. The 1H NMR spectrum of 4 showed signals at δH 4.97 (s) and 5.27 (d) assignable to H-2 and H-4, respectively, as supported by HMBC correlations of H-2/C-3, C-4, C-1′, C-2′, C-6′, and of H-4/C-2, C-3, C-10 (Figure 2). Additionally, reaction of lupinifolinol (3) with NH2OH·HCl gave oximes 6 and 7 in 35 and 18% isolated yields, respectively.
These transformations indicated that the 3-hydroxy-4-keto function in 1, 2 and 3 could isomerize to 4-hydroxy-3-keto analogs through a ketol rearrangement under the influence of NH2OH·HCl, leading subsequently to the formation of corresponding 4-hydroxy-3-oximes (4 and 6). There are a number of reports on the rearrangement reactions of α-hydroxy-keto compounds, some contains a tertiary alcohol group4 and some with a secondary alcohol group as found in steroids5-7 and carbohydrates8 but none of the 3-hydroxyflavanone was reported previously.
Since both 3α- and 3β-hydroxyprenylflavanones (1 and 2) gave the same 4-hydroxy-3-oxime (4), we thus proposed two possible transformation pathways. Pathway a, an acid catalyzed rearrangement of 3-hydroxy-4-keto compound proceeds via an enediol intermediate 4a, which is protonated preferentially on the α-face to furnish the thermodynamically more stable 4β-hydroxy-3-keto analog (4b). The latter could then react further with NH2OH·HCl to yield the corresponding oxime 4.
Pathway b involves a stereoselective 1,2-hydride migration of the 3α-H of 1 leading to the formation of 4b as a sole intermediate which, with NH2OH·HCl, yields 4. Protonation of the carbonyl oxygen atom facilitates the hydride shift as illustrated in Figure 3. The formation of 4b from 2, the 3-epimer of 1, involves solely an enediol intermediate 4a (pathway a, Figure 3), since a stereospecific 1,2-hydride migration of the 3β-H of 2 would lead to the 4α-hydroxy-3-keto intermediate and finally to the corresponding 4α-hydroxy-3-oxime, which was not observed in this study. The use of density functional theory (DFT) calculation at the B3LYP/6-31G(d) level of theory showed that 4 is the thermodynamically most favored and is most stable when the oxygen atom of the 3-NOH group is H-bond to the hydrogen atom of the 4-OH group as indicated in Figure 4.
We also found that both 3β-O-acetyl derivatives of 1, compounds 8 and 9, transformed to 4β-O-acetyl-3-oxime 10 (in 32 and 18% isolated yield, respectively) after reacting with NH2OH·HCl. These transformations are proposed to occur by an acid-catalyzed reaction via a 5-membered ring intermediate (8a and 9a). The ring opening step promotes the stereospecific 1,2-hydride shift of the 3α-H giving 10a which is transformed into 10 under the influence of NH2OH·HCl. Based on the proposed pathway (Figure 5), the configuration at C-4 of 10 could therefore be established as S. Under this reaction condition, the more electrophilic 5-O-acetyl group in 8 was also hydrolyzed.
Upon reacting either 4 or 10 with Ac2O/pyridine and DMAP, the triacetate 11 was obtained (Figure 6). Compound 11 which was obtained from 4 showed [α]D29 −75.6 (c 1.98, CHCl3), whereas 11 from 10 exhibited [α]D29 −76.2 (c 0.70, CHCl3). Based on this data, the configuration at C-4 of oxime 4 obtained from both the 3α- and 3β-O-hydroxyflavanones (1 and 2) could also be proposed as S.
We also found that reaction of 3β,5,4′-tri-O-acetylhydroxyflavonane 12 with NH2OH·HCl yielded 5,4′-dideacetyl- 4β-O-acetyl-3-oxime 13 with only one O-acetyl group at C-4 as a sole product, showing [α]D29 −64.9 (c 0.70, CHCl3). The reaction between 12 and NH2OH·HCl proceeds with similar rearrangement pathway (Figure 5) and led to the hydrolysis of the more electrophilic 5- and 4′-acetyl groups.
It is also worth noting that the 4-oxime derivatives (5 and 7) obtained as a minor product from the reaction of 1 and 3, respectively with NH2OH·HCl, exhibited 1H NMR chemical shifts of H-2 and H-3 as doublets at ca. δH 5.07, and 5.22, respectively. The vicinal coupling constant, J2,3 was found to be 6.9 Hz, which is noticeably less than that found (ca. 12 Hz) in the corresponding protons of the starting materials 1 and 3.1 The change of the J2,3 value in 4-oxime obtained from 3-hydroxyflavanone was documented to be due to the distortion of hetero ring with C=N bond causing a change by ca. 20-30º of the H-2-H-3 dihedral angle as compared with the ketone as found in 1 and 3.3 However, the 4-oxime derivative of the 3α-hydroxyflavanone (2), which might provide further evidence concerning stereochemistry at C-3, was unfortunately not obtained under similar condition.
Due to the stability and limit quantities of the products obtained, only compounds 1, 3-9 were evaluated for their cytotoxic activities against human breast adenocarcinoma (MCF-7), human oral epidermoid carcinoma (KB) and human cervical carcinoma (Hela) cell lines (Table 1). Results showed that both 3- and 4-oxime derivatives of khonklonginol A (compounds 4 and 5) were less active than the parent compound (1) particularly against KB cell line. The inhibitory activity of the 4-oxime of lupinifolinol (7) was less potent than lupinifolinol (3) against all cell lines tested, while the 3-oxime of lupinifolinol (6) exhibited comparable inhibitory activity against KB cell line as that of 3, however compound 6 also showed strong inhibitory activity against MCF-7 and Hela cell lines.
This is among a few report on oximination of 3-hydroxyflavanones and their 3-O-acetyl derivatives with NH2OH·HCl. Based on experimental results, the configuration at C-4 of the 3-oxime derivatives could be proposed as S. Cytotoxic activities of some synthetic products were evaluated and some exhibited broader spectrum of activities than the parent flavonoid.
EXPERIMENTAL
General experimental procedures
Solvents and reagents were dried by distillation from the usual drying agents prior to use: dichloromethane was distilled from calcium hydride, and pyridine was distilled from barium oxide. The progression of reactions was monitored by analytical thin layer chromatography using Merck precoated silica gel 60 F254 aluminum sheets and analyzed with 254 nm UV light. Flash column chromatography was performed using Merck silica gel 60. The yields referred to the isolated yields of compounds after purification. Melting points were measured on an electrothermal apparatus and are uncorrected. Optical rotations were recorded on a JASCO DIP 1020 polarimeter spectropolarimeter. The IR spectra were run on a Perkin-Elmer 1760x FT-IR spectrophotometer. The 1H, 13C, and 2D NMR data were recorded on a Bruker AVANCE 400 MHz spectrometer. The chemical shifts are in parts per million quoted relative to the residual signals of chloroform (δH 7.24 and δC 77.0 ppm) as the internal standard. HRESIMS was recorded on a Bruker Daltonics micro TOF mass spectrometer.
Computational Methods
The optimized structures of compounds were computed with the density functional theory (DFT) calculation method. DFT calculations were performed with the Becke’s three-parameter exchange functional9 with the Lee-Yang-Parr correlation functional (B3LYP).10 All optimized structures were obtained by computation at the B3LYP/6-31G(d) level of theory using the GAUSSIAN09 program.11
Method A, Oximination: Preparation of 4β-hydroxy-3-oxime flavanone (4) and 3β-hydroxy-4-oxime flavanone (5) from 1: To a stirred solution containing 97.5 mg (0.229 mmol, 1.0 equiv) of khonklonginol A1 (1) in dried pyridine (2 mL) was added 162.2 mg (2.291 mmol, 10.0 equiv) of NH2OH·HCl. The reaction mixture was stirred under N2 atmosphere at 65 °C for 60 h. After completion, the mixture was quenched with 5 mL of water and extracted with 2×10 mL of CH2Cl2. The combined organic extract was washed with 2×5 mL of an aqueous 1N HCl, followed by 10 mL of water, then dried over anhydrous Na2SO4, and the filtrate was concentrated using rotary evaporator under reduced pressure. The crude residue was purified by silica gel column chromatography eluting with CH2Cl2 to yield 23.8 mg (24%) of compound 4 together with 8.9 mg (9%) of compound 5.
3-Oxime of khonklonginol A (4): a yellow amorphous solid; [α]D29−28.6 (c 0.44, MeOH); IR (KBr) νmax 3379, 2922, 2851, 1640, 1614, 1516, 1463, 1376, 1304, 1249, 1198, 1173, 1127, 1097, 1031 cm-1; 1H NMR (400 MHz, CDCl3) δH: 7.47 (2H, d, J = 8.6 Hz, H-2′, H-6′), 6.96 (2H, d, J = 8.6 Hz, H-3′, H-5′), 6.65 (1H, d, J = 9.9 Hz, H-4′′), 5.48 (1H, d, J = 9.90 Hz, H-5′′), 5.27 (1H, d, J = 1.6 Hz, H-4), 5.18 (1H, t, J = 7.3 Hz, H-2′′′), 4.97 (1H, s, H-2), 3.82 (3H, s, OCH3-4′), 3.30 (1H, dd, J = 13.9, 7.3 Hz, H-1′′′), 3.25 (1H, dd, J = 13.9, 7.3 Hz, H-1′′′), 1.67 (3H, s, CH3-3′′′), 1.62 (3H, s, CH3-3′′′), 1.41 (3H, s, CH3-6′′), 1.40 (3H, s, CH3-6′′); 13C NMR (100 MHz, CDCl3) δC: 159.6 (C, C-4′), 156.0 (C, C-3), 154.6 (C, C-7), 154.4 (C, C-9), 152.4 (C, C-5), 130.6 (C, C-3′′′), 128.1 (C, C-1′), 127.9 (CH, C-2′, C-6′), 126.5 (CH, C-5′′), 123.1 (CH, C-2′′′), 116.5 (CH, C-4′′), 113.9 (CH, C-3′, C-5′), 109.6 (C, C-8), 104.1 (C, C-6), 95.9 (C, C-10), 79.3 (CH, C-2), 76.8 (C, C-6′′), 61.3 (CH, C-4), 55.3 (CH3, OCH3-4′), 28.1 (CH3, CH3-6′′), 28.0 (CH3, CH3-6′′), 25.8 (CH3, CH3-3′′′), 21.7 (CH2, C-1′′′), 17.9 (CH3, CH3-3′′′); HR-ESI-MS m/z: 452.2073 [M+H]+ (calcd for C26H30NO6, 452.2073).
4-Oxime of khonklonginol A (5): a yellow amorphous solid, [α]D29−24.9 (c 0.77, CHCl3); IR (KBr) νmax 3367, 2975, 2924, 2855, 1639, 1614, 1518, 1450, 1375, 1240, 1200, 1169, 1126, 1102, 1027, 1013 cm-1 ;1H NMR (400MHz, CDCl3) δH 10.50 (br s, OH), 7.93 (br s, OH), 7.31 (2H, d, J = 8.6 Hz, H-2′, H-6′), 6.86 (2H, d, J = 8.6 Hz, H-3′, H-5′), 6.62 (1H, d, J = 9.9 Hz, H-4′′), 5.46 (1H, d, J = 9.9 Hz, H-5′′), 5.23 (1H, d, J = 6.9 Hz, H-3), 5.12 (1H, t, J = 7.2 Hz, H-2′′′), 5.08 (1H, d, J = 6.9 Hz, H-2), 3.78 (3H, s, OCH3-4′), 3.21 (2H, d, J = 6.5 Hz, H-1′′′), 1.63 (3H, s, CH3-3′′′), 1.61 (3H, s, CH3-3′′′), 1.41 (3H, s, CH3-6′′), 1.38 (3H, s, CH3-6′′); 13C NMR (100 MHz, CDCl3) δC 159.6 (C, C-4′), 156.4 (C, C-4), 154.6 (C, C-7), 153.9 (C, C-9), 152.1 (C, C-5), 130.7 (C, C-3′′′), 129.2 (C, C-1′), 128.2 (C, C-2′, C-6′), 126.4 (CH, C-5′′), 122.9 (CH, C-2′′′), 116.5 (CH, C-4′′), 113.9 (C, C-3′, C-5′), 109.5 (C, C-8), 104.1 (C, C-6), 96.9 (C, C-10), 80.2 (CH, C-2), 76.9 (C, C-6′′), 66.1 (CH, C-3), 55.3 (CH3, OCH3-4′), 28.2 (CH3, CH3-6′′), 27.9 (CH3, CH3-6′′), 25.8 (CH3, CH3-3′′′), 21.6 (CH2, C-1′′′), 17.8 (CH3, CH3-3′′′); HR-ESI-MS m/z: 452.2075 [M+H]+ (calcd for C26H30NO6, 452.2073).
3-Oxime of khonklonginol B (4) from 2: a yellow amorphous solid, [α]D29−19.7 (c 1.23, MeOH); HR-ESI-MS m/z: 452.2081 [M+H]+ (calcd for C26H30NO6, 452.2073).
Compounds 6 and 7 were prepared according to method A using 61.7 mg (0.146 mmol, 1.0 equiv) of lupinifolinol (3) in dried pyridine (1 mL) with 103.0 mg (1.420 mmol, 9.7 equiv) of NH2OH·HCl (30 h) to yield 22.2 mg (35%) of 6 and 11.7 mg (18%) of compound 7.
3-Oxime of lupinifolinol (6): a sticky solid, [α]D29 –24.6 (c 2.94, MeOH); IR (KBr) νmax 3400, 2974, 2925, 2855, 1637, 1614, 1518, 1384, 1236, 1169, 1126, 1105 cm-1; 1H NMR (400 MHz, CDCl3) δH: 7.39 (2H, d, J = 8.4 Hz, H-2′, H-6′), 6.85 (2H, d, J = 8.4 Hz, H-3′, H-5′), 6.63 (1H, d, J = 9.9 Hz, H-4′′), 5.47 (1H, d, J = 9.9 Hz, H-5′′), 5.25 (1H, br s, H-4), 5.17 (1H, t, J = 7.2 Hz, H-2′′′), 4.94 (1H, br s, H-2), 3.27 (2H, m, H-1′′′), 1.66 (3H, s, CH3-3′′′), 1.61 (3H, s, CH3-3′′′), 1.39 (2H, s, 2×CH3-6′′); 13C NMR (100 MHz, CDCl3) δC: 155.5 (C, C-3, C-4′), 154.5 (C, C-7), 154.4 (C, C-9), 152.2 (C, C-5), 130.8 (C, C-3′′′),128.0 (CH, C-2′, C-6′), 126.6 (C, C-5′′), 123.1 (CH, C-2′′′), 116.4 (CH, C-4′′), 115.4 (CH, C-3′, C-5′), 109.3 (C, C-8), 104.1 (C, C-10), 96.0 (C, C-10), 79.2 (CH, C-2), 76.8 (C, C-6′′), 61.3 (CH, C-4), 28.1 (CH3, C-6′′), 27.9 (CH3, C-6′′), 25.8 (CH3, C-3′′′), 21.7 (CH2, C-1′′′), 17.9 (CH3, C-3′′′); HR-ESI-MS m/z: 438.1914 [M+H]+ (calcd for C25H28NO6 438.1917).
4-Oxime of lupinifolinol (7): a yellow amorphous solid, [α]D29 –119.5 (c 0.88, MeOH); IR (KBr) νmax 3367, 2924, 2855, 1639, 1614, 1518, 1375, 1240, 1200, 1169, 1126, 1102 cm-1; 1H NMR (400 MHz, CDCl3) δH 10.50 (1H, br s, OH), 7.93 (1, br s, OH), 7.25 (2H, d, J = 8.4 Hz, H-2′, H-6′), 6.77 (2H, d, J = 8.4 Hz, H-3′, H-5′), 6.61 (1H, d, J = 10.0 Hz, H-4′′), 5.47 (1H, d, J = 10.0 Hz, H-5′′), 5.21 (1H, d, J = 6.9 Hz, H-3), 5.06 (1H, d, J = 6.9 Hz, H-2), 5.11 (1H, t, J = 7.3 Hz, H-2′′′), 3.21 (2H, d, J = 6.0 Hz, H-1′′′), 1.62 (3H, s, H-3′′′), 1.61 (3H, s, H-3′′′), 1.41 (3H, s, H-6′′), 1.38 (3H, s, H-6′′); 13C NMR (100 MHz, CDCl3) δC 156.6 (C, C=N), 154.8 (C, C-4′), 153.8 (C, C-7), 153.6 (C, C-9), 151.6 (C, C-5), 130.5 (C, C-3′′′), 128.3 (C, C-1′), 128.1 (CH, C-2′, C-6′), 126.2 (CH, C-5′′), 123.0 (CH, C-2′′′), 116.4 (C-4′′), 115.1 (CH, C-3′, C-5′), 109.1 (C, C-8), 103.7 (C, C-6) , 97.2 (C, C-10), 80.3 (CH, C-2), 76.5 (C, C-6′′), 65.1 (CH, C-3), 27.9 (CH3, C-6′′), 27.6 (CH3, C-6′′), 25.5 (CH3, C-3′′′), 21.4 (CH2, C-1′′′), 17.6 (CH3, C-3′′′); HR-ESI-MS m/z: 438.1916 [M+H]+ (calcd for C25H28NO6, 438.1917).
Method B, Acetylation reaction: Preparation of 3,5-di-O-acetylkhonklonginol A (8) and 3-O-acetylkhonklonginol A (9): To a stirred solution containing 52.0 mg (0.119 mmol, 1.0 equiv) of khonklonginol A (1) in 0.1 mL of dried pyridine and 0.1 mL of acetic anhydride, was added catalytic amount of DMAP. The reaction mixture was stirred at room temperature under N2 atmosphere and monitored the progression of the reaction by TLC. Upon completion (5 h), the mixture of EtOAc (10 mL) and water (10 mL) was added, the layers were separated and the aqueous layer was further extracted with EtOAc (10 mL). The combined organic layer was then dried over anhydrous Na2SO4 and the filtrate concentrated using rotary evaporator. The crude residue was purified by silica gel column chromatography using hexane-EtOAc (94:6 to 85:15) to afford 48.7 mg (82%) of compound 8 together with 13.2 mg (24%) of compound 9.
3,5-Di-O-acetylkhonklonginol A (8): an amorphous solid, [α]D29 +43.0 (c 0.87, MeOH); IR (KBr) νmax 2976, 2922, 1761, 1605, 1517, 1465, 1187, 1123 cm-1; 1H NMR (400 MHz, CDCl3) δH: 7.35 (2H, d, J = 8.8 Hz, H-2′, H-6′), 6.90 (2H, d, J = 8.0 Hz, H-3′, H-5′), 6.35 (1H, d, J = 8.0 Hz, H-4′′), 5.65 (1H, d, J = 12.0 Hz, H-2), 5.63 (1H, d, J = 8.0 Hz, H-4′′), 5.27 (1H, d, J = 12.0 Hz, H-3), 5.10 (1H, tt, J = 7.6, 1.2 Hz, H-2′′′), 3.81 (3H, s, OCH3-4′), 3.24 (1H, dd, J = 13.8, 7.5 Hz, H-1′′′), 3.19 (1H, dd, J = 14.2, 8.0 Hz, H-1′′′), 2.38 (3H, s, OCOCH3-5), 1.98 (3H, s, OCOCH3-3), 1.62 (3H, s, CH3-3′′′), 1.55 (3H, s, CH3-3′′′), 1.44 (3H, s, CH3-6′′), 1.42 (3H, s, CH3-6′′); 13C NMR (100 MHz, CDCl3) δC: 185.6 (C, C-4), 169.3 (C, 2 × OCOCH3), 160.2 (C, C-4′, C-7), 157.7 (C, C-9), 144.1 (C, C-5), 131.8 (C, C-3′′′), 129.9 CH, C-5′′), 128.7 (CH, C-2′, C-6′), 127.8 (C, C-1′), 121.4 (CH, C-2′′′), 115.3 (CH, C-4′′), 113.9 (CH, C-3′, C-5′), 109.9 (C, C-8), 106.9 (C, C-6), 80.8 (CH, C-2), 78.1 (C, C-6′′), 73.4 (CH, C-3), 55.3 (CH3, OCH3-4′), 28.4 (2×CH3, CH3-6′′), 25.8 (CH3, CH3-3′′′), 21.8 (CH2, C-1′′′), 20.9 (CH3, OCOCH3-5), 20.4 (CH3, OCOCH3-3), 17.8 (CH3, CH3-3′′′); HR-ESI-MS m/z: 543.1994 [M+Na]+ (calcd for C30H32O8Na, 543.1995).
3-O-Acetylkhonklonginol A (9): a yellow amorphous solid, IR (KBr) νmax: 2922, 1756, 1586, 1463, 1190, 1123 cm-1; 1H NMR (400 MHz, CDCl3) δH 11.68 (br s, OH-5), 7.36 (2H, d, J = 8.7 Hz, H-2′, H-6′), 6.91 (2H, d, J = 8.7 Hz, H-3′, H-5′), 6.61 (1H, d, J = 10.0 Hz, H-4′′), 5.75 (1H, d, J = 11.8 Hz, H-2), 5.50 (1H, d, J = 10.0 Hz, H-5′′), 5.25 (1H, d, J = 11.8 Hz, H-3), 5.09 (1H, tt, J = 7.4, 1.2 Hz, H-2′′′), 3.82 (3H, s, OCH3-4′), 2.01 (3H, s, OCOCH3), 3.14 (2H, dd, J = 7.4, 2.7 Hz, H-1′′′), 1.62 (3H, s, CH3-3′′′), 1.56 (3H, s, CH3-3′′′), 1.43 (3H, s, CH3-6′′), 1.42 (3H, s, CH3-6′′); 13C NMR (100 MHz, CDCl3) δC 192.2 (C, C-4), 169.3 (C, OCOCH3), 160.4 (C, C-4′), 160.3 (C, C-7), 158.8 (C, C-9), 156.5 (C, C-5), 131.3 (C, C-3′′′), 128.7 (CH, C-2′, C-6′), 127.7 (C, C-1′), 126.2 (CH, C-5′′), 122.2 (CH, C-2′′′), 115.5 (CH, C-4′′), 114.0 (CH, C-3′, C-5′), 109.1 (C, C-8), 103.3 (C, C-6), 101.4 (C, C-10), 80.8 (CH, C-2), 78.4 (C, C-6′′), 72.6 (CH, C-3), 55.3 (CH3, OCH3-4′), 28.4 (2×CH3, CH3-6′′), 25.8 (CH3, CH3-3′′′), 21.3 (CH2, C-1′′′), 20.4 (CH3, OCOCH3), 17.8 (CH3, CH3-3′′′); HR-ESI-MS m/z: 501.1889 [M+Na]+ (calcd for C28H30O7Na, 501.1889).
Preparation of 4-O-acetyl-3-oxime flavanone 10 from 8: compound 10 was prepared according to method A using 13.0 mg (0.025 mmol, 1.0 equiv) of 8 in 0.5 mL of dried pyridine with 17.1 mg (0.250 mmol, 10.0 equiv) of NH2OH·HCl (30 h) to obtain 4.0 mg (32%) of 10.
Preparation of 4-O-acetyl-3-oxime flavanone 10 from 9: compound 10 was prepared according to method A using 21.0 mg (0.044 mmol, 1.0 equiv) of 9 in 0.5 mL of dried pyridine with 30.5 mg (0.440 mmol, 10.0 equiv) of NH2OH·HCl (32 h) to obtain 3.8 mg (18%) of 10.
4-O-Acetyl-3-oxime flavanone (10): a yellow amorphous solid, [α]D29−74.7 (c 1.12, MeOH); IR (KBr) νmax 3401, 2973, 2925, 2855, 1755, 1733, 1641, 1615, 1587, 1516, 1462, 1374, 1303, 1250, 1219, 1203, 1173, 1128, 1101, 1031 cm-1; 1H NMR (400 MHz, CDCl3) δH: 7.37 (2H, d, J = 8.7 Hz, H-2′, H-6′), 6.89 (2H, d, J = 8.7 Hz, H-3′, H-5′), 6.67 (1H, d, J = 9.9 Hz, H-4′′), 6.53 (1H, d, J = 1.9 Hz, H-4), 5.49 (1H, d, J = 9.9 Hz, H-5′′), 5.18 (1H, t, J = 7.3 Hz, H-2′′′), 5.07 (1H, d, J = 1.9 Hz, H-2), 3.81 (3H, s, OCH3-4′´), 3.30 (1H, dd, J = 14.0, 7.2 Hz, H-1′′′), 3.22 (1H, dd, J = 14.0, 7.2 Hz, H-1′′′), 1.85 (3H, s, OCOCH3-4), 1.64 (3H, s, CH3-3′′′), 1.62 (3H, s, CH3-3′′′), 1.42 (3H, s, CH3-6′′), 1.40 (3H, s, CH3-6′′); 13C NMR (100 MHz, CDCl3) δC: 168.6 (C, OCOCH3), 159.6 (C, C-4′), 154.6 (C, C-7, C-9), 152.4 (C, C-3), 152.2 (C, C-5), 130.7 (C, C-3′′′), 127.8 (CH, C-2′, C-6′), 127.5 (C, C-1′), 126.5 (CH, C-5′′), 123.0 (CH, C-2′′′), 116.5 (CH, C-4′′), 113.7 (CH, C-3′, C-5′), 109.3 (C, C-8), 104.1 (C, C-6), 96.3 (C, C-10), 78.5 (CH, C-2), 76.9 (C, C-6′′), 61.1 (CH, C-4), 55.2 (CH3, OCH3-4′), 28.1 (CH3, CH3-6′′), 28.0 (CH3, CH3-6′′), 25.8 (CH3, CH3-3′′′), 21.7 (CH2, C-1′′′), 20.4 (CH3, OCOCH3), 17.9 (CH3, CH3-3′′′); HR-ESI-MS m/z: 516.2009 [M+Na]+ (calcd for C28H31NO7Na, 516.1998).
Preparation of triacetate derivative 11 from 4β-hydroxy-3-oxime flavanone 4: compound 11 was prepared according to method B using 9.3 mg (0.021 mmol, 1.0 equiv) of 4 and catalytic amount of DMAP in 0.5 mL of acetic anhydride and 0.5 mL of dried pyridine (20 h) to afford 4.7 mg (40%) of 11.
Preparation of triacetate derivative 11 from 4β-O-acetyl-3-oxime flavanone 10: compound 11 was prepared according to method B using 11.2 mg (0.023 mmol, 1.0 equiv) of 10 and catalytic amounts of DMAP in 0.05 mL of acetic anhydride and 0.05 mL of pyridine (25 h) to afford 7.0 mg (54%) of 11.
Compound 11 from 4: a yellow amorphous solid, [α]D29−75.6 (c 1.98, CHCl3); IR (KBr) νmax 2926, 2852, 1755, 1634, 1612, 1516, 1464, 1371, 1251, 1216, 1127, 1030 cm-1; 1H NMR (400 MHz, CDCl3) δH 7.34 (2H, d, J = 8.7 Hz, H-2′, H-6′), 6.90 (2H, d, J = 8.7 Hz, H-3′, H-5′), 6.45 (1H, br s, H-4), 6.35 (1H, br s, H-4′′), 5.63 (1H, d, J = 10.0 Hz, H-5′′), 5.16 (1H, t, J = 7.2 Hz, H-2′′′), 5.14 (1H, br s, H-2), 3.80 (3H, s, OCH3-4′), 3.35 (1H, dd, J = 13.7, 7.3 Hz, H-1′′′), 3.28 (1H, dd, J = 13.9, 7.4 Hz, H-1′′′), 2.45 (3H, s, N-O-COCH3-3), 2.08 (3H, s, OCOCH3-5), 1.82 (3H, s, OCOCH3-4), 1.63 (6H, s, CH3-3′′′), 1.44 (3H, s, CH3-6′′), 1.41 (3H, s, CH3-6′′); 13C NMR (100 MHz, CDCl3) δC 168.1 (C, OCOCH3), 167.0 (C, OCOCH3), 166.98 (C, OCOCH3), 159.8 (C, C-4′), 156.6 (C, C=N), 155.0 (C, C-9), 153.4 (C, C-7), 142.7 (C, C-5), 131.5 (C, C-1′), 130.0 (CH, C-5′′), 127.8 (CH, C-2′, C-6′), 127.1 (C, C-3′′′), 121.9 (CH, C-2′′′), 115.9 (CH, C-4′′), 115.7 (C, C-10), 113.7 (CH, C-3′, C-5′), 110.1 (C, C-8), 106.3 (C, C-6), 78.5 (CH, C-2), 77.5 (C, C-6′′), 63.3 (CH, C-4), 55.2 (CH3, OCH3-4′), 28.3 (CH3, CH3-6′′), 28.2 (CH3, CH3-6′′), 25.8 (CH3, CH3-3′′′), 22.1 (CH2, C-1′′′), 21.2 (CH3, OCOCH3), 20.3 (CH3, OCOCH3), 19.5 (CH3, OCOCH3), 17.9 (CH3, CH3-3′′′); HR-ESI-MS m/z: 600.2210 [M+Na]+ (calcd for C32H35NO9Na, 600.2210).
Compound 11 from 10: a yellow amorphous solid, [α]D29 −76.2 (c 0.70, CHCl3).
Preparation of 12: Compound 12 was prepared according to method B using 66.0 mg (0.156 mmol, 1.0 equiv) of lupinifolinol (3) and catalytic amount of DMAP in 0.5 mL of acetic anhydride and 0.5 mL of pyridine (12 h) to obtain 89.6 mg (100%) of 12.
3,5,4′-Tri-O-acetyllupinifolinol (12): a yellow amorphous solid, [α]D29 +36.2 (c 0.82, MeOH); IR (KBr) νmax 3503, 2919, 1771, 1760, 1640, 1511, 1466, 1371, 1216, 1166 cm-1; 1H NMR (400 MHz, CDCl3) δH 7.45 (2H, d, J = 8.5 Hz, H-2′, H-6′), 7.13 (2H, d, J = 8.5 Hz, H-3′, H-5′), 6.35 (1H, d, J = 10.1 Hz, H-4′′), 5.63 (1H, d, J = 10.1 Hz, H-5′′), 5.62 (1H, d, J = 12.1 Hz, H-2), 5.34 (1H, d, J = 12.1 Hz, H-3), 5.09 (1H, t, J = 7.3 Hz, H-2′′′), 3.26 (1H, dd, J = 13.8, 7.5 Hz, H-1′′′), 3.19 (1H, dd, J = 13.8, 7.5 Hz, H-1′′′), 2.38 (3H, s, OCOCH3), 2.29 (3H, s, OCOCH3), 1.99 (3H, s, OCOCH3), 1.62 (3H, s, CH3-3′′′), 1.57 (3H, s, CH3-3′′′), 1.44 (3H, s, CH3-6′′), 1.42 (3H, s, CH3-6′′); 13C NMR (100 MHz, CDCl3) δC 185.2 (C, C-4), 169.2 (C, OCOCH3), 169.1 (C, OCOCH3), 160.0 (C, C-9), 157.8 (C, C-7), 151.3 (C, C-4′), 144.1 (C, C-5), 133.2 (C, C-1′), 131.8 (C, C-3′′′), 130.0 (CH, C-5′′), 128.4 (CH, C-2′, C-6′), 121.7 (CH, C-3′, C-5′), 121.4 (CH, C-2′′′), 115.4 (C, C-10), 115.3 (CH, C-4′′), 110.1 (C, C-8), 106.2 (C, C-6), 80.4 (CH, C-2), 78.2 (C, C-6′′), 73.4 (CH, C-3), 28.4 (2×CH3, CH3-6′′), 25.7 (CH3, CH3-3′′′), 21.8 (CH2, C-1′′′), 21.1 (CH3, OCOCH3), 20.9 (CH3, OCOCH3), 20.4 (CH3, OCOCH3), 17.8 (CH3, CH3-3′′′); HR-ESI-MS m/z: 549.2114 [M+H]+ (calcd for C31H33O9, 549.2125).
Preparation of 4β-O-acetyl-3-oxime flavanone 13: compound 13 was prepared according to method A using 20.0 mg (0.037 mmol, 1 .0 equiv) of 12 in 0.5 mL of dried pyridine with 27.0 mg (0.365 mmol, 10.0 equiv) of NH2OH·HCl (for 42 h) to yield 7.0 mg (40%) of 13.
4β-O-Acetyl-3-oxime flavanone 13: a yellow amorphous solid, [α]D29−64.9 (c 0.70, CHCl3); IR (KBr) νmax 3368, 2922, 2853, 1730, 1615, 1519, 1240, 1169, 1101 cm-1; 1H NMR (400 MHz, CDCl3) δH 7.31 (2H, d, J = 8.5 Hz, H-2′, H-6′) , 6.81 (2H, d, J = 8.5 Hz, H-3′, H-5′), 6.65 (1H, d, J = 9.9 Hz, H-4′′), 5.49 (1H, d, J = 9.9 Hz, H-5′′), 5.18 (1H, t, J = 7.4 Hz, H-2′′′), 5.06 (1H, d, J = 1.5 Hz, H-2), 3.30 (1H, dd, J = 13.9, 7.6 Hz, H-1′′′), 3.22 (1H, dd, J = 13.6 , 7.5 Hz, H-1′′′), 1.87 (3H, s, OCOCH3), 1.64 (3H, s, CH3-3′′′), 1.62 (3H, s, CH3-3′′′), 1.42 (3H, s, CH3-6′′′), 1.40 (3H, s, CH3-6′′); 13C NMR (100 MHz, CDCl3) δC 169.3 (C, OCOCH3), 155.9 (C, C-4), 154.6 (C, C-7), 154.5 (C, C-9), 152.3 (C, C-3), 152.1 (C, C-5), 130.7 (C, C-3′′′), 127.9 (CH, C-2′, C-6′), 127.4 (C, C-1′), 126.6 (CH, C-5′′), 123.0 (CH, C-2′′′), 116.4 (CH, C-4′′), 115.3 (CH, C-3′, C-5′), 109.3 (C, C-8), 104.1 (C, C-6), 96.3 (C, C-10), 78.5 (CH, C-2), 76.9 (C, C-6′′), 61.4 (CH, C-4), 28.1 (CH3, C-6′′), 28.0 (CH3, C-6′′), 25.8 (CH3, C-3′′′), 21.7(CH3, C-1′′′), 20.4 (CH3, OCOCH3), 17.9 (CH3, C-3′′′); HR-ESI-MS m/z: 480.2021 [M+H]+ (calcd for C27H31NO7, 480.2022).
Bioassay: cytotoxicity assays were performed using MTT colorimetric assay as previously reported.12
ACKNOWLEDGEMENTS
We are grateful to Ramkhamhaeng University for financial support. S.T. and R.S. acknowledge the Center of Excellence for Innovation in Chemistry (PERCH-CIC), the Office of Higher Education, Ministry of Education for a scholarship. We thank Professor R. Grigg, the School of Chemistry, University of Leeds, for his valuable comment. We acknowledge the Chemistry Department of Chiangmai University, and Chulabhorn Research Institute for HRMS measurements.
References
1. S. Sutthivaiyakit, O. Thongnak, T. Lhinhatrakool, O. Yodchun, R. Srimark, P. Dowtaisong, and M. Chuankamnerdkarn, J. Nat. Prod., 2009, 72, 1092. CrossRef
2. C. Yenjai and S. Wanich, Bioorg. Med. Chem. Lett., 2010, 20, 2821. CrossRef
3. G. Janzsó, F. Kállay, I. Koczor, and L. Radics, Tetrahedron, 1967, 23, 3699. CrossRef
4. L. A. Paquette and J. E. Hofferberth, Chapter 3. The α-hydroxyl ketone (α-ketol) and related rearrangements. In L. E. Overman, et al., (Eds.), Org. Reactions, Vol. 62, John Wiley, 2003, pp. 477−567.
5. M. Numazawa and M. Nagaoka, J. Org. Chem., 1982, 47, 4024. CrossRef
6. M. Numazawa, M. Nagaoka, and A. Mutsumi, Chem. Pharm. Bull., 1987, 35, 4763. CrossRef
7. A. C. Bruttomesso, D. Doller, and E. G. Gros, Bioorg. Med. Chem., 1999, 7, 943. CrossRef
8. D. W. Harris and M. S. Feather, J. Amer. Chem. Soc., 1975, 97, 178. CrossRef
9. A. D. Becke, J. Chem. Phys., 1993, 98, 5648. CrossRef
10. C. Lee, W. Yang, and R. G. Parr, Phys. Rev., 1988, B37, 785.
11. M. J. Frisch, G. W. Trucks, H. B. Schlegel, G. E. Scuseria, M. A. Robb, J. R. Cheeseman, G. Scalmani, V. Barone, B. Mennucci, G. A. Petersson, H. Nakatsuji, M. Caricato, X. Li, H. P. Hratchian, A. F. Izmaylov, J. Bloino, G. Zheng, J. L. Sonnenberg, M. Hada, M. Ehara, K. Toyota, R. Fukuda, J. Hasegawa, M. Ishida, T. Nakajima, Y. Honda, O. Kitao, H. Nakai, T. Vreven, Jr., J. A. Montgomery, J. E. Peralta, F. Ogliaro, M. Bearpark, J. J. Heyd, E. Brothers, K. N. Kudin, V. N. Staroverov, R. Kobayashi, J. Normand, K. Raghavachari, A. Rendell, J. C. Burant, S. S. Iyengar, J. Tomasi, M. Cossi, N. Rega, N. J. Millam, M. Klene, J. E. Knox, J. B. Cross, V. Bakken, C. Adamo, J. Jaramillo, R. Gomperts, R. E. Stratmann, O. Yazyev, A. J. Austin, R. Cammi, C. Pomelli, J. W. Ochterski, R. L .Martin, K. Morokuma, V. G. Zakrzewski, G. A. Voth, P. Salvador, J. J. Dannenberg, S. Dapprich, A. D. Daniels, Ӧ. Farkas, J. B. Foresman, J. V. Ortiz, J. Cioslowski, and D. J. Fox, Gaussian09, Revision B.01, Gaussian, Inc., Wallingford CT, 2009.
12. P. Siripong, C. Hahnvajanawong, J. Yahuafai, S. Piyaviriyakul, K. Kanokmedhakul, N. Kongkathip, S. Ruchirawat, and N. Oku, Biol. Pharm. Bull., 2009, 32, 1251. CrossRef