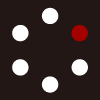
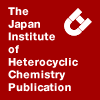
HETEROCYCLES
An International Journal for Reviews and Communications in Heterocyclic ChemistryWeb Edition ISSN: 1881-0942
Published online by The Japan Institute of Heterocyclic Chemistry
e-Journal
Full Text HTML
Received, 17th August, 2012, Accepted, 24th September, 2012, Published online, 2nd October, 2012.
DOI: 10.3987/COM-12-12570
■ Behaviour of β-Keto-δ-carbethoxyphosphonates and Phosphine Oxides in the Biginelli Multicomponent Reaction: Regioselective Synthesis of 5-Carbethoxy-6-phosphonomethyl-3,4-dihydropyrimidin-2-ones
Emna Chebil and Soufiane Touil*
Department of Chemistry, Faculty of Sciences of Bizerta, 7021-Jarzouna, Tunisia
Abstract
An efficient one-pot synthesis of the novel 5-carbethoxy-6-phosphonomethyl-3,4-dihydropyrimidin-2-ones via a three-component Biginelli-type condensation of β-keto-δ-carbethoxyphosphonates and phosphine oxides with aldehydes and urea in the presence of a catalytic amount of acetic acid, is described. The reaction proceeded efficiently at room temperature to afford regioselectively the title compounds in good to high yields. The factors governing the regioselectivity of the reaction are discussed.Multicomponent reactions (MCRs) represent one of the most important tools of organic synthesis and medicinal chemistry.1 The diversity, efficiency and rapid access to small and highly functionalized organic molecules makes this approach of central current interest in the construction of combinatorial libraries and optimization in drug discovery process. The search for new MCRs on one hand, and the full exploitation of already known multicomponent reactions on the other hand, is therefore of considerable interest.
The Biginelli reaction2 is an easy and useful three-component synthesis which involves the condensation of an aldehyde, urea, and a β-ketoester, under acid catalysis, to yield a 3,4-dihydropyrimidone derivative (Scheme 1). In this area, we have recently shown that β-ketophosphonates undergo a Biginelli-type condensation to give 5-phosphono-3,4-dihydropyrimidin-2-ones3 (Scheme 2).
Keeping in view of the above facts, we decided to investigate the behaviour of β-keto-δ-carbethoxyphosphonates and phosphine oxides 1 in the Biginelli reaction (Figure 1). Our main objective here was to compare the reactivity of carbons in the α and α’ positions with the keto function in order to identify the factors which appear to govern regioselectivity in such Biginelli reactions. The second goal of this work was the synthesis of novel 3,4-dihydropyrimidone derivatives bearing phosphoryl and ester groups. It is important to note here that dihydropyrimidone derivatives are associated with a wide range of biological properties including antimicrobial,4 antiviral,5 anti-inflammatory6 and anticancer7 activities. Most of these compounds are also medicinally important as calcium channel modulators.4 Furthermore, it is known that phosphorus substituents regulate important biological functions8 and the introduction of organophosphorus functionalities in the dihydropyrimidone core could improve the biological activity of such compounds.
The starting β-keto-δ-carbethoxyphosphonates and phosphine oxides 1 were easily prepared according to reported procedures.9 In theory, the condensation of these compounds with aldehydes and urea, performed under the Biginelli reaction conditions can lead either to the dihydropyrimidone derivative 2 or 2', or to a mixture of these compounds. The reaction pathway2 was assumed to proceed via a nucleophilic attack of urea on the aldehyde giving rise to an iminium intermediate. The interception of this last one by the keto-ester phosphonate through its enol tautomers, leads after intramolecular cyclization and dehydration to the dihydropyrimidones 2 and 2’ (Scheme 3).
The reaction was found to be completely regioselective and furnished exclusively the 2-regioisomers in good to high yields. The orientation of the reaction towards the formation of pyrimidones 2 can be attributed to the reactivity of carbons at the α and α’ positions relative to the keto function. These carbons reacting through their enol tautomer forms, we can easily realize that the α-carbon, despite it is less nucleophilic, is more reactive than the other one probably because it corresponds to the more stable enolic form. Indeed theoretical RHF/6-31G calculation, performed with Gaussian 03 program, showed that the α’-carbon is more nucleophilic than the other one (Scheme 4) and that the energies of tautomers A are much lower than those of tautomers B with a stabilization of about 9 kcal/mol in favour of the form A. These results strongly suggest that the regioselectivity of the reaction is governed, not by the nucleophilicity of α- and α’-carbons, but by the stability of the corresponding enolic forms.
Experimentally, the reaction was achieved by treatment of keto-ester phosphonates 1 with equimolar quantities of aldehyde and urea, using a catalytic amount of acetic acid, in ethanol as solvent, and stirring the mixture at 25 °C for 48–72 h. The scope of the reaction was assessed with a range of keto-ester phosphonates and aldehydes. All substrates reacted in good to high yields (Table 1).
It is important to note here that raising the temperature and using other solvents (MeCN, THF) showed no changes in regioselectivity but resulted in a decrease in yields of products 2. Furthermore, when the reaction was carried out at 0 °C, no product was observed even after a prolonged period of time.
The structures of dihydropyrimidones 2 were established through IR, NMR (1H, 31P, 13C) and mass spectral data. The IR spectra of compounds 2 showed characteristic absorption bands around 1700 and 1650 cm-1 attributable respectively to the C=O of amide and ester groups. The later is moved towards the low frequencies because of the conjugation with the pyrimidone nucleus. We observed, on the other hand, a broad band in the region 3200-3400 cm-1 corresponding to the N-H vibrators. Another band ascribable to the P=O group is present at nearly 1250 cm-1.
The 1H NMR spectra showed in particular the presence of broad singlets at 6 and 9 ppm, corresponding to the protons of NH groups. The CH2-P protons appear in the region included between 3.5 and 4.5 ppm as a doublet (2JPH = 9-15 Hz) and in some cases as two multiplets indicating that they are not magnetically equivalent. We observed also a triplet and quartet towards 1 and 4 ppm respectively, attributable to the protons of the ethoxycarbonyl group.
Other evidence of structure for compounds 2 is provided by 13C NMR. We observed in particular a doublet at 30-45 ppm, ascribable to the CH2-P carbon. Such a doublet is characteristic of the coupling with phosphorus with a 1JCP coupling constant of about 60-140 Hz. We observed, on the other hand, the characteristic signals of amide and ester carbons around 150 and 160 ppm respectively.
Structures of compounds 2 were supported additionally by the mass spectra which showed the correct molecular ion peaks.
In conclusion, we successfully developed an efficient and regioselective multicomponent synthesis of novel dihydropyrimidone derivatives bearing phosphoryl and ester groups, from the Biginelli-type condensation of β-keto-δ-carbethoxyphosphonates and phosphine oxides with aldehydes and urea in the presence of a catalytic amount of acetic acid. The regioselectivity of the reaction was found to be governed, not by the nucleophilicity of α- and α’-carbons relative to the keto function, but by the stability of the corresponding enolic forms.
The synthesized compounds might show enhanced biological activity due to the presence of both dihydropyrimidone and phosphoryl moieties. Furthermore they contain an activated phosphonomethyl group that enable them to perform Horner-Wadsworth-Emmons reaction resulting in various dihydropyrimidones with alkenyl substituents, which can be modified to give additional dihydropyrimidone derivatives. These studies are ongoing in our laboratory and will be reported in due course.
EXPERIMENTAL
1H, 31P and 13C NMR spectra were recorded with DMSO-d6 as the solvent, on a Bruker-300 spectrometer. The chemical shifts are reported in ppm relative to TMS (internal reference) for 1H and 13C NMR and relative to 85% H3PO4 (external reference) for 31P NMR. The coupling constants are reported in Hz. For the 1H NMR, the multiplicities of signals are indicated by the following abbreviations: s: singlet, d: doublet, t: triplet, q: quartet, m: multiplet. Mass spectra were determined on an Agilent 5975B spectrometer, under electronic impact (EI) conditions. IR spectra were recorded on a Nicolet IR200 spectrometer. The progress of the reactions was monitored by TLC. Purification of products was performed by column chromatography using silica gel 60 (Fluka).
General procedure for the synthesis of 5-carbethoxy-6-phosphonomethyl-3,4-dihydropyrimidin-2-ones 2. A mixture of β-keto-δ-carbethoxyphosphonate or phosphine oxide 1 (0.01 mol), aldehyde (0.01 mol), urea (0.015 mol) and glacial acetic acid (0.1 mL), in ethanol (10 mL) was stirred at 25 °C for 48-72 h (Table 1). The reaction mixture was then concentrated under vacuum. The residue obtained was chromatographed on a silica gel column using Et2O as eluent.
2a: Clear yellow solid; mp 107-108 °C; 1H NMR (300 MHz, DMSO-d6): δ = 0.96 (t, 3H, 3JHH = 6.0 Hz, CH3-CH2-O); 3.53 (m, 1H, CH2-P); 3.86 (q, 2H, 3JHH = 6.0 Hz, CH3-CH2-O); 4.76 (m, 1H, CH2-P); 5.44 (s, 1H, CH-N); 6.59 (br s, 1H, N-H); 6.76-7.75 (m, 15H, arom-H); 8.43 (br s, 1H, N-H); 13C NMR (75.5 MHz, DMSO-d6): δ = 15.2 (s, CH3-CH2-O); 31.6 (d, 1JCP = 62.6 Hz, CH2-P); 55.4 (s, CH-N); 65.8 (s, CH3-CH2-O); 102.8 (d, 3JCP = 7.5 Hz, O=C-C=C); 142.8 (d, 2JCP = 9.0 Hz, O=C-C=C); 151.9 (s, N-C=O); 165.5 (d, 4JCP = 2.3 Hz, O-C=O); phenyl carbons: δ =125.5, 126.5, 127.5, 127.9, 128.5,128.6, 128.8, 129.4, 130.9, 131.2, 131.8, 132.3; IR (neat): νP=O = 1234 cm-1; νC=O (ester) = 1642 cm-1; νC=O (amide) = 1703 cm-1; νNH = 3248-3371 cm-1; EI-HRMS: calculated for C26H25N2O4P, 460.1552 (M+); found: 460.1554.
2b: White solid; mp 173-174 °C; 1H NMR (300 MHz, DMSO-d6): δ = 0.76 (t, 3H, 3JHH = 6.0 Hz, CH3-CH2-CH); 1.19 (t, 3H; 3JHH = 6.0 Hz, CH3-CH2-O); 2.08-2.20 (m, 2H, CH3-CH2-CH); 3.94 (q, 2H, 3JHH = 6.0 Hz, CH3-CH2-O); 4.03 (t, 1H, 3JHH = 6.0 Hz, CH-N) ; 4.06 (d, 2H, 2JPH = 15.0 Hz, CH2-P); 6.68 (br s, 1H, N-H); 7.37-7.85 (m, 10H, arom-H); 9.13 (br s, 1H, N-H); 13C NMR (75.5 MHz, DMSO-d6): δ = 13.8 (s, CH3-CH2-CH); 15.8 (s, CH3-CH2-O); 23.8 (s, CH3-CH2-CH); 45.3 (d, 1JCP = 58.9 Hz, CH2-P); 51.0 (s, CH-N); 60.5 (s, CH3-CH2-O); 106.2 (d, 3JCP = 7.3 Hz, O=C-C=C); 135.9 (d, 2JCP = 9.7 Hz, O=C-C=C); 154.1 (s, N-C=O); 160.6 (s, O-C=O); phenyl carbons: δ = 120.6, 128.1, 128.3, 130.7, 130.9, 131.7, 131.8, 132.0; IR (neat): νP=O = 1261 cm-1; νC=O(ester) = 1643 cm-1; νC=O (amide) = 1691 cm-1; νNH = 3328-3350 cm-1; EI-HRMS: calculated for C22H25N2O4P, 412.1552 (M+); found: 412.1556.
2c: Clear yellow solid; mp 135-136 °C; 1H NMR (300 MHz, DMSO-d6): δ = 0.83 (t, 3H, 3JHH = 6.0 Hz, CH3-CH2-O); 1.30 (d, 6H, 3JHH = 6.0 Hz, (CH3)2CH); 3.76 (m, 3H, CH3-CH2-O and (CH3)2CH); 3.85 (d, 2H, 2JPH = 15.0 Hz, CH2-P); 5.73 (br s, 1H, N-H); 6.05 (d, 1H, 3JHH = 6.0 Hz, CH-N); 6.29 (br s, 1H, N-H); 7.26-7.61 (m, 10H, arom-H); 13C NMR (75.5 MHz, DMSO-d6): δ = 16.1 (s, CH3-CH2-O); 20.8 (s, (CH3)2CH); 22.1 (s, (CH3)2CH); 32.3 (s, (CH3)2-CH); 32.7 (d, 1JCP = 75.5 Hz, CH2-P); 56.2 (s, CH-N); 60.5 (s, CH3-CH2-O); 107.8 (d, 3JCP = 7.8 Hz, O=C-C=C); 132.0 (d, 2JCP = 8.3 Hz, O=C-C=C); 156.1 (s, N-C=O); 166.5 (s, O-C=O); phenyl carbons: δ = 118.8, 128.5, 128.7, 130.5, 130.4, 131.6, 131.9, 132.0; IR (neat): νP=O = 1267 cm-1; νC=O (ester ) = 1658 cm-1; νC=O (amide) = 1684 cm-1; νNH = 3240-3359 cm-1; EI-HRMS: calculated for C23H27N2O4P, 426.1708 (M+); found: 426.1709.
2d: Yellow oil; 1H NMR (300 MHz, DMSO-d6): δ = 0.75 (d, 6H, 3JHH = 6.0 Hz, (CH3)2CH); 1.08 (t, 3H, 3JHH = 6.0 Hz, CH3-CH2-O); 1.98-2.03 (m, 2H, (CH3)2CH-CH2); 2.18-2.25 (m, 1H, (CH3)2CH-CH2); 3.80-4.10 (m, 4H, CH2-P and CH3-CH2-O); 5.78 (t, 1H, 3JHH = 6.0 Hz, CH-N); 6.53 (br s, 1H, N-H); 7.36-7.79 (m, 10H, arom-H); 8.67 (br s, 1H, N-H); 13C NMR (75.5 MHz, DMSO-d6): δ = 13.5 (s, CH3-CH2-O); 20.1 (s, (CH3)2CH-CH2); 22.8 (s, (CH3)2CH-CH2); 26.8 (s, (CH3)2CH-CH2); 30.0 (d, 1JCP = 81.5 Hz, CH2-P); 31.4 (s, (CH3)2CH-CH2); 47.2 (s, CH-N); 59.8 (s, CH3-CH2-O); 101.9 (d, 3JCP = 6.8 Hz, O=C-C=C); 141.9 (d, 2JCP = 9.8 Hz, O=C-C=C); 151.4 (s, N-C=O); 164.1 (s, O-C=O); phenyl carbons: δ = 126.8, 127.0, 127.4, 129.4, 130.8, 130.6, 131.2, 132.5; IR (neat): νP=O = 1228 cm-1; νC=O (ester) = 1670 cm-1; νC=O (amide) = 1722 cm-1; νNH = 3229-3375 cm-1; EI-HRMS: calculated for C24H29N2O4P, 440.1865 (M+); found: 440.1862.
2e: Yellow oil; 1H NMR (300 MHz, DMSO-d6): δ = 1.07-130 (m, 9H, 3 CH3-CH2-O); 3.59 (d, 2H, 2JPH = 12 Hz, CH2-P ); 3.90-4.15 (m, 6H, 3 CH3-CH2-O); 5.72 (s, 1H, CH-N); 5.79 (br s, 1H, N-H); 7.15-7.63 (m, 5H, arom-H); 9.32 (br s, 1H, N-H); 13C NMR (75.5 MHz, DMSO-d6): δ = 13.4 (s, CH3-CH2-O); 15.9 (d, 3JCP = 4.5 Hz, CH3-CH2-O-P); 28.2 (d, 1JCP = 144.2 Hz, CH2-P); 53.9 (s, CH-N); 61.9 (s, CH3-CH2-O); 62.0 (d, 2JCP = 6.0 Hz, CH3-CH2-O-P); 101.3 (d, 3JCP = 8.9 Hz, O=C-C=C); 144.6 (d, 2JCP = 9.8 Hz, O=C-C=C); 152.4 (s, N-C=O); 165.3 (s, O-C=O); phenyl carbons: δ = 126.0, 128.1, 128.3, 128.8; IR (neat): νP=O = 1237 cm-1; νC=O (ester) = 1655 cm-1; νC=O (amide) = 1698 cm-1; νNH = 3248-3359 cm-1; EI-HRMS: calculated for C18H25N2O6P, 396.1450 (M+); found: 396.1457.
2f: Yellow solid; mp 214-216 °C; 1H NMR (300 MHz, DMSO-d6): δ = 0.78 (d, 6H, 3JHH = 6.0 Hz, (CH3)2CH); 1.00-1.21 (m, 10H, 3 CH3-CH2-O and (CH3)2CH); 3.80-4.10 (m, 8H, 3 CH3-CH2-O and CH2-P ); 4.57 (d, 1H, 3JHH = 9.0 Hz, CH-N); 7.09 (br s, 1H, N-H); 9.02 (br s, 1H, N-H); 13C NMR (75.5 MHz, DMSO-d6): δ = 13.8 (s, CH3-CH2-O); 15.7 (s, (CH3)2CH); 16.0 (s, (CH3)2CH); 17.4 (d, 3JCP = 4.2 Hz, CH3-CH2-O-P); 32.2 (d, 1JCP = 122.3 Hz, CH2-P); 34.4 (s, (CH3)2CH); 55.5 (s, CH-N); 61.8 (d, 2JCP = 6.0 Hz, CH3-CH2-O-P); 63.2 (s, CH3-CH2-O); 98.5 (s, O=C-C=C); 147.9 (s, O=C-C=C); 153.9 (s, N-C=O); 161.5 (s, O-C=O); IR (neat): νP=O = 1246 cm-1; νC=O (ester) = 1663 cm-1; νC=O (amide) = 1716 cm-1; νNH = 3219-3362 cm-1; EI-HRMS: calculated for C15H27N2O6P, 362.1607 (M+); found: 362.1602.
2g: Brown oil; 1H NMR (300 MHz, DMSO-d6): δ = 0.86 (d, 6H, 3JHH = 6.0 Hz, (CH3)2CH); 1.18-1.31 (m, 11H, 3 CH3-CH2-O and (CH3)2CH-CH2); 1.95-2.24 (m, 1H, (CH3)2CH-CH2); 3.95-4.12 (m, 9H, 3 CH3-CH2-O, CH2-P and CH-N); 7.01 (br s, 1H, N-H); 7.91 (br s, 1H, N-H); 13C NMR (75.5 MHz, DMSO-d6): δ = 13.7 (s, CH3-CH2-O); 14.0 (d, 3JCP = 4.5 Hz, CH3-CH2-O-P); 20.4 (s, (CH3)2CH-CH2); 21.4 (s, (CH3)2CH-CH2); 23.7 (s, (CH3)2CH-CH2); 28.1 (s, (CH3)2CH-CH2); 43.7 (d, 1JCP = 129.8 Hz, CH2-P); 49.6 (s, CH-N); 60.5 (s, CH3-CH2-O); 61.9 (d, 2JCP = 6.0 Hz, CH3-CH2-O-P); 101.9 (s, O=C-C=C); 147.0 (s, O=C-C=C); 154.9 (s, N-C=O); 162.2 (s, O-C=O); IR (neat): νP=O = 1239 cm-1; νC=O (ester) = 1665 cm-1; νC=O (amide) = 1712 cm-1; νNH = 3224-3372 cm-1; EI-HRMS: calculated for C16H29N2O6P, 376.1763 (M+); found: 376.1759.
2h: Clear brown solid; mp 165-166 °C; 1H NMR (300 MHz, DMSO-d6): δ = 1.07 (t, 3H, 3JHH = 6.0 Hz, CH3-CH2-O); 3.65 (d, 2H, 2JPH = 12.0 Hz, CH2-P); 3.94 (d, 6H, 3JPH = 3.0 Hz, 2 CH3-O); 4.02 (q, 2H, 3JHH= 6.0 Hz, CH3-CH2-O); 5.13 (s, 1H, CH-N); 6.96 (br s, 1H, N-H); 7.17-7.33 (m; 5H; arom-H); 9.13 (br s, 1H, N-H); 13C NMR (75.5 MHz, DMSO-d6): δ = 15.1 (s, CH3-CH2-O); 43.1 (d, 1JCP = 113.2 Hz, CH2-P); 55.1 (d, 2JCP = 6.1 Hz, CH3-O-P); 59.7 (s, CH-N); 65.7 (s, CH3-CH2-O); 102.6 (d, 3JCP = 8.6 Hz, O=C-C=C); 145.4 (s, O=C-C=C); 153.2 (s, N-C=O); 164.5 (s, O-C=O); phenyl carbons: δ= 126.5, 126.7, 127.8, 128.5; IR (neat): νP=O = 1234 cm-1; νC=O (ester) = 1659 cm-1; νC=O (amide)= 1695 cm-1; νNH = 3219-3281 cm-1; EI-HRMS: calculated for C16H21N2O6P, 368.1137 (M+); found: 368.1134.
2i: Yellow oil; 1H NMR (300 MHz, DMSO-d6): δ = 1.10 (t, 3H, 3JHH = 6.0 Hz, CH3-CH2-CH); 1.13 (t, 3H, 3JHH = 6.0 Hz, CH3-CH2-O); 1.48-1.58 (m, 2H, CH3-CH2-CH); 3.54 (d, 2H, 2JPH = 9.0 Hz, CH2-P); 3.89-4.09 (m, 8H, CH3-CH2-O and 2 CH3-O); 5.30 (t, 1H, 3JHH = 9.0 Hz, CH-N); 6.48 (br s, 1H, N-H); 9.99 (br s, 1H, N-H); 13C NMR (75.5 MHz, DMSO-d6): δ = 13.7 (s, CH3-CH2-CH); 18.2 (s, CH3-CH2-O); 26.0 (s, CH3-CH2-CH); 43.6 (d, 1JCP = 99.6 Hz, CH2-P); 51.9 (d, 2JCP = 6.8 Hz, CH3-O-P); 56.0 (s, CH-N); 61.0 (s, CH3-CH2-O); 101.7 (d, 3JCP = 8.2 Hz, O=C-C=C); 131.8 (s, O=C-C=C); 153.9 (s, N-C=O); 161.4 (s, O-C=O); IR (neat): νP=O = 1273 cm-1; νC=O (ester) = 1659 cm-1; νC=O (amide) = 1728 cm-1; νNH = 3364-3372 cm-1; EI-HRMS: calculated for C12H21N2O6P, 320.1137 (M+); found: 320.1131.
2j: Brown oil; 1H NMR (300 MHz, DMSO-d6): δ = 0.90 (d, 6H, 3JHH = 6.0 Hz, (CH3)2CH); 1.14 (t, 3H, 3JHH = 6.0 Hz, CH3-CH2-O ); 1.12-1.19 (m, 1H, (CH3)2CH); 3.59 (d, 2H, 2JPH = 12.0 Hz, CH2-P); 4.01 (d, 6H, 3JPH = 3.0 Hz, 2 CH3-O); 4.05 (q, 2H, 3JHH = 6.0 Hz, CH3-CH2-O); 4.10 (d, 1H, 3JHH = 6.0 Hz, CH-N); 6.51 (br s, 1H, N-H); 10.02 (br s, 1H, N-H); 13C NMR (75.5 MHz, DMSO-d6): δ = 13.7 (s, CH3-CH2-O); 19.0 (s, (CH3)2CH); 20.8 (s, (CH3)2CH); 28.9 (s, (CH3)2CH); 43.6 (d, 1JCP = 99.6 Hz, CH2-P); 49.4 (s, CH-N); 51.9 (d, 2JCP = 6.0 Hz, CH3-O-P); 61.0 (s, CH3-CH2-O); 103.4 (d, 3JCP = 8.7 Hz, O=C-C=C); 140.6 (s, O=C-C=C); 154.0 (s, N-C=O); 161.3 (s, O-C=O); IR (neat): νP=O = 1239 cm-1; νC=O (ester) = 1663 cm-1; νC=O (amide) = 1716 cm-1; νNH = 3119-3199 cm-1; EI-HRMS: calculated for C13H23N2O6P, 334.1294 (M+); found: 334.1299.
2k: Brown oil; 1H NMR (300 MHz, DMSO-d6): δ = 0.85 (d, 6H, 3JHH = 6.0 Hz, (CH3)2CH); 1.15 (t, 3H, 3JHH = 6.0 Hz, CH3-CH2-O); 2.22-2.24 (m, 2H, (CH3)2CH-CH2); 3.33-3.40 (m, 1H, (CH3)2CH-CH2); 3.35 (d, 2H, 2JPH = 12.0 Hz, CH2-P); 3.39 (d, 6H, 3JPH = 3.0 Hz, 2 CH3-O); 3.79 (q, 2H, 3JHH = 6.0 Hz, CH3-CH2-O); 4.50 (t, 1H, 3JHH = 6.0 Hz, CH-N); 6.32 (br s, 1H, N-H); 9.22 (br s, 1H, N-H); 13C NMR (75.5 MHz, DMSO-d6): δ = 13.7 (s, CH3-CH2-O); 18.1 (s, (CH3)2CH-CH2); 20.8 (s, (CH3)2CH-CH2); 24.4 (s, (CH3)2CH-CH2); 36.4 (s, (CH3)2CH-CH2); 42.3 (s, CH-N); 47.0 (d, 1JCP = 118.5 Hz, CH2-P); 51.9 (d, 2JCP = 9.7 Hz, CH3-O-P); 60.7 (s, CH3-CH2-O); 102.6 (s, O=C-C=C); 133.3 (s, O=C-C=C); 154.0 (s, N-C=O); 166.5 (s, O-C=O); IR (neat): νP=O = 1273 cm-1; νC=O (ester) = 1659 cm-1; νC=O (amide) = 1724 cm-1; νNH = 3294-3389 cm-1; EI-HRMS: calculated for C14H25N2O6P, 348.1450 (M+); found: 348.1453.
ACKNOWLEDGEMENTS
We thank the Tunisian Ministry of Higher Education and Scientific Research for financial support.
References
1. For reviews concerning multicomponent reactions, see: A. Dömling and I. Ugi, Angew. Chem. Int. Ed., 2000, 39, 3168; CrossRef V. Nair, C. Rajesh, A. U. Vinod, S. Bindu, A. R. Sreekenth, and L. Balagopal, Acc. Chem. Res., 2003, 36, 899; CrossRef J. P. Zhu and H. Bienayme, ‘Multicomponent Reactions’, Eds. Wiley-VCH: Weinheim, Germany, 2005; A. Dömling, Chem. Rev., 2006, 106, 17; CrossRef D. Tejedor and F. Garcia-Tellado, Chem. Soc. Rev., 2007, 36, 484; CrossRef J. D. Sunderhaus and S. F. Martin, Chem. Eur. J., 2009, 15, 1300. CrossRef
2. For reviews on the Biginelli reaction, see: C. O. Kappe, Tetrahedron, 1993, 49, 6937; CrossRef C. O. Kappe, Acc. Chem. Res., 2000, 33, 879; CrossRef C. O. Kappe and A. Stadler, Org. Reactions, 2004, 63, 1; S. V. Vdovina and V. A. Mamedov, Russ. Chem. Rev., 2008, 77, 1017; CrossRef M. Syamala, Org. Prep. Proced. Int., 2009, 41, 1. CrossRef
3. I. Essid and S. Touil, Tetrahedron Lett., Submitted for publication on 4 August, 2012.
4. C. O. Kappe, Eur. J. Med. Chem., 2000, 35, 1043. CrossRef
5. E. W. Hurst and R. Hull, J. Med. Pharm. Chem., 1961, 3, 215. CrossRef
6. B. Tozkoparan, M. Ertan, P. Kelicen, and R. Demirdamar, Farmaco, 1999, 54, 588; CrossRef Y. S. Sadanandam, M. M. Shetty, and P. V. Dwan, Eur. J. Med. Chem., 1992, 27, 87. CrossRef
7. C. M. Wright, R. J. Chovatiya, N. E. Jameson, D. M. Turner, G. Zhu, S. Werner, D. M. Huryn, J. M. Pipas, B. W. Day, P. Wipf, and J. L. Brodsky, Bioorg. Med. Chem., 2008, 16, 3291. CrossRef
8. F. Palacios, C. Alonso, and J. M. de los Santos, Chem. Rev., 2005, 105, 899; CrossRef P. Kafarski and B. Lejezak, Phosphorus & Sulfur, 1991, 63, 193; CrossRef R. E. Hoagland, ‘Biologically Active Natural Products’, ed. by H. G. Culter, ACS Symposium Series 380. American Chemical Society: Washington DC, 1988, p. 182; A. D. F. Toy and E. N. Walsh, ‘Phosphorus Chemistry in Everyday Living’, American Chemical Society, Washington D. C., 1987; R. Engel, ‘Handbook of Organophosphorus Chemistry’, M. Dekker Inc., New York, 1992.
9. N. Said, S. Touil, and H. Zantour, Phosphorus, Sulfur and Silicon Rel. Elem., 2004, 179, 2487. CrossRef