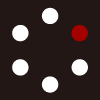
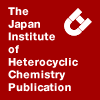
HETEROCYCLES
An International Journal for Reviews and Communications in Heterocyclic ChemistryWeb Edition ISSN: 1881-0942
Published online by The Japan Institute of Heterocyclic Chemistry
e-Journal
Full Text HTML
Received, 19th August, 2012, Accepted, 12th October, 2012, Published online, 19th October, 2012.
DOI: 10.3987/COM-12-12569
■ Ketalization of Glycerol with Acetone to O-Heterocyclic Compounds over ZrO2-SiO2 Solid Acid Catalysts
Chunni Fan, Chenghua Xu,* Chuanqi Liu, Zunyu Huang, Jianying Liu, and Zhixiang Ye
Air Environmental Modeling and Pollution Controlling, Key Laboratory of Sichuan Higher Education Institutes, Chengdu University of Information Technology, No. 24, Block 1, Xuefu Road, Chengdu, Sichuan Province 610225, China
Abstract
The solid acid catalysts ZrO2-SiO2 mixed oxides were prepared by sol-gel, characterized by X-ray diffraction, NH3-temperature programmed desorption, fourier transform infrared spectroscopy, and applied in the glycerol catalytic ketalization with acetone to O-heterocyclic compounds in the present work. Simultaneously, the effects of the catalysts composition, calcination temperature and ketalization parameters such as reaction time, acetone/glycerol, catalyst amount and reaction temperature on the ketalization were also investigated in detail. The results showed that the main product for the glycerol ketalization with acetone was solketal with a 5-membered ring along with a small amount of 2,2-dimethyl-1,3-dioxan-5-ol with a 6-membered ring. ZrO2-SiO2 with a Zr/Si molar ratio of 0.5 calcined at 500 oC exhibited the best catalytic property in ketalization, gave 90.91% of glycerol conversion and 89.69% of solketal selectivity under the optimum conditions. It was also found that glycerol catalytic ketalization with acetone to solketal was mainly governed by kinetics.Biodiesel as a renewable fuel has been paid more and more attentions recently and successfully implemented in many countries. However, during the present biodiesel production from the transesterification of vegetable oils and animal fats with methanol, glycerol as a by-product will be produced.1 Therefore, the reasonable utilization is very important for the sustainable development of biodiesel industry. As reported, glycerol can be catalytically converted to propanediols2,3 and hydrogen or syngas4,5 over noble metal catalysts through hydrogenolysis and reforming, respectively. Additionally, two adjacent hydroxyl groups in glycerol molecular structure are reported to function with ethanediamine to form value-added chemicals pyrazinyl compounds with a N-heteroatom ring.6,7
Moreover, it has also been discovered that glycerol can be used in the synthesis of ketals with an O-heteroatomic ring through ketalization with acetone. The obtained product such as solketal ((2,2-dimethyl-1,3-dioxolan-4-yl)methanol) can serve as a high-octane component of gasolines, reduce the freezing point of diesel fuel and improve their lubricating properties.8,9 As well known, the used catalysts in the conventional ketalization are inorganic proton acids such as H2SO4, H3PO4 or HCl. However, they can lead to the production of an amount of by-products along with serious erosion for devices and environmental pollution. Therefore, the solid acidic catalysts, such as cation-exchanged resins,10,11 heteropolyacids (HPAs),12 zeolites13,14 and Ti-exchanged montmorillonite14 have been attracted more and more interests. In addition, some Zr-containing mixed oxides such as WOx/ZrO2, MoOx/ZrO2 and SO42-/ZrO2, have also been applied in glycerol catalytic ketalization with acetone (see Scheme 1),15 however their catalytic life have not been reported. As reported, TiO2-SiO2 and ZrO2-SiO2 mixed oxides can provide Lewis and Brønsted sites simultaneously.16-19 Our previous work20 investigated the acidity and catalytic property of TiO2-SiO2 mixed oxides in glycerol ketalization with acetone. It indicated that TiO2-SiO2 gave rise to a high yield of solketal (about 90%) with a 5-membered ring containing O-heteroatom.
In the present work, another kind of solid acid catalysts ZrO2-SiO2 mixed oxides for glycerol catalytic ketalization with acetone to ketals with O-heteroatomic ring are synthesized by sol-gel method, characterized by X-ray diffraction (XRD), NH3-temperature programmed desorption (NH3-TPD), fourier transform infrared spectroscopy (FT-IR). Moreover, the effect of ZrO2-SiO2 composition, catalysts calcination temperature and ketalization parameters such as reaction time, acetone/glycerol, catalyst amount and reaction temperature on the glycerol ketalization, are further investigated in detail.
CATALYST COMPOSITION
Powder XRD results of ZS samples (Figure 1) indicate that all the mixed oxides show no obvious diffraction peaks in the 2θ range from 15o to 30o, it shows that SiO2 species in samples are amorphous.15 However, there are some strong characteristic peaks tetragonal ZrO2 (PDF No. 49-1644) of at 2θ= 30.2o, 35.4o, 50.6o, 60.1o and 74.5o, respectively.16,18 Moreover, the intensities of these characteristic peaks strengthen with increasing Zr amount in ZS samples. It indicates that zirconia species in samples mainly exist as tetragonal phase and there is no evidence of the zircon (ZrSiO4) formation, which is similar to the results reported elsewhere.16,17
FT-IR spectra of compressed KBr pellets containing 1 wt% ZS samples calcined at 500 oC for 4 h (Figure 2) show that all catalysts give rise to two strong adsorption peaks at about 3500 cm-1 and 1636 cm-1, respectively. They are generally assigned to the stretching and bending vibration of H-O-H bonds of water molecules adsorbed on the samples.21 Moreover, two peaks are reduced with an increasing Zr/Si molar ratio in ZS samples, indicating that their hydrophilicity is also decreased. From Figure 2, it can be also found that pure SiO2 exhibits a broad band at 1097 cm-1 with a shoulder at about 1220 cm-1 which is due to the Si-O-Si asymmetric stretching vibration, and simultaneously gives other two peaks assigned to the Si-OH stretching of terminal silanols and the ring structure of Si-O-Si bridges at 960 cm-1 and 800 cm-1,16,22 respectively. Moreover, these peaks are also reduced with an increase of Zr/Si ratio. These results indicate that the presence of ZrO2 leads to the formation Si-O-Zr linkages in replacement of Si-O-Si bonds, and Zr species can also interact with the terminal Si-OH groups to form Si-O-Zr linkages in ZS samples.
From NH3-TPD results of ZS samples (Figure 3), it is not difficult to discover that NH3-adsorption ability of ZS catalysts increases with the increasing ZrO2 content and reaches the maximum when Zr/Si molar ratio = 0.5. Moreover, pure SiO2 only exhibits a weak NH3-desorption peak, which is due to only the presence of terminal Si-OH groups only providing some weak Brønsted acidic sites.17 With the increase of ZiO2 content, the formation of more Zr-O-Si linkages in ZS-X samples will give rise to the formation of new Lewis acidic sites, and activate the ionization of terminal Si-OH groups to increase the strength of Brønsted acidic sites due to the difference of the polarity between Si-O and Zr-O bonds. However, the presence of too much ZrO2 species will consume the terminal Si-OH groups during the formation of Zr-O-Si linkages tested by the above FT-IR results, thus the amount of total acidic sites will be decreased in some extent.
Figure 4 exhibits the catalytic property of ZS-X mixed oxide catalysts with a different Zr/Si moral ratios in the glycerol ketalization with acetone under the conditions of catalyst amount 2.2 g per 100 g glycerol, acetone/glycerol molar ratio 4 and reaction at 70 oC for 3 h. It can be observed that pure SiO2 as catalyst gives a poor glycerol conversion, the glycerol conversion and solketal selectivity over ZS-X catalysts are found to increase with the increasing ZrO2 amount to a maximum of 90.2% when Zr/Si molar ratio = 0.5, and then decrease in some extent with the further increasing Zr/Si molar ratio. This trend is completely coincident with the relationship between the amount of total acidic sites and ZrO2 content in ZS-X catalysts (compare Figure 3(b) and Figure 4). These results indicate that the glycerol ketalization with acetone mainly occurs over the Lewis and Brønsted acidic sites provided by Si-O-Zr linkages or terminal Si-OH groups, respectively. Meanwhile, the selectivity towards solketal is found to be higher than that of 2,2-dimethyl-1,3-dioxan-5-ol (5-ol). The similar results have also been obtained over the other solid acid catalysts reported in previous studies.12-15
CALCINATION TEMPERATURE
From the above results, it is found that the ZS-0.5 catalyst with a Zr/Si ratio of 0.5 provides the most amount of total acidic sites and also exhibits the highest catalytic activity in glycerol ketalization with acetone. The present work also investigates the effect of calcination temperature on physicochemical property and catalytic activity of ZS-0.5 catalysts. From Figure 5, it can be observed that too low or too high calcination temperature can lead to the formation of less total acidic sites over the surface of ZS-0.5 catalyst. It is perhaps because that the low temperature is disadvantageous to the formation of Zr-O-Si linkages, however, the too high calcination temperature can lead to the destruction of Zr-O-Si linkages. They will result in the decrease on the total acidic sites. From the XRD results (Figure 6), it can be found that the intensities of peaks characteristic of tetragonal zirconia at 2θ= 30.2o, 35.4o, 50.6o and 60.1o, increase when ZS-0.5 catalyst is calcined at a low temperature (400 oC) and too high temperature (>600 oC). It shows that the formation of Zr-O-Si linkages which can provides more acidic sites, mainly from the interaction between amorphous SiO2 species and tetragonal zirconia phase.
Figure 7 shows the catalytic results of glycerol ketalization with acetone over ZS-0.5 catalysts calcined at the different temperature. It can be clearly observed that both glycerol conversion and solketal selectivity first increase and then decrease with the increasing calcination temperature, while the selectivity towards 5-ol with a 6-membered ring exhibits a reverse trend. It further proves that too low or high calcination temperature will influence the formation of Zr-O-Si linkages related with the acidic sites of catalysts. More acidic sites and strong acidity are helpful to the glycerol ketalization with acetone and the formation of 5-membered ring ketal (solketal).12,13
KETALIZATION PARAMETERS
Under the conditions of catalyst amount 2.2 g per 100 g glycerol, molar ratio of acetone to glycerol 4 and reaction temperature 70 oC, the effect of reaction time on the glycerol ketalization with acetone over ZS-X catalyst calcined at 500 oC is investigated. From Figure 8(a), it is easily found that both glycerol conversion and solketal selectivity increase with the increasing reaction time. It indicates that a long reaction time is helpful to glycerol conversion. In consideration of the increase rate of glycerol conversion with reaction time, the proper reaction time is selected to be 180 min in the following work.
The effect of acetone/glycerol molar ratio on the glycerol ketalization is also investigated while the catalyst amount, reaction temperature and time (180 min) are kept constant. From Figure 8(b), it can be discovered that both glycerol conversion and solketal selectivity also increase with the increasing acetone amount when molar ratio of acetone to glycerol being < 4, and exhibit no obvious increase with the further increasing acetone amount (acetone/glycerol molar ratio >4). It is possibly because that the presence of proper excess acetone can lead to the ample dispersion of glycerol in reaction mixture which is advantageous to ketalization, and however too much acetone can result in the decrease on the catalyst concentration which is disadvantageous to ketalization and the formation of product solketal with 5-membered ring.
As well-known, an important function of catalyst is to reduce the activation energy. According to Arrhenius’ law, both adding catalyst and increasing reaction temperature can increase the reaction rate. From Figure 8(c) and Figure 8(d), it can be found that the increase of reaction temperature and catalyst amount promotes the conversion of glycerol and formation of solketal, and inhibit the formation of 5-ol. It proves that the formation of solketal with 5-membered ring is the kinetics-governed reaction which possesses a higher activation energy than the thermodynamics-governed one (5-ol formation).12,13
From the catalytic cycle results (Figure 1S in the Supplementary material) of ZS-0.5, it is shown that its catalytic activity almost has no obvious decrease after 5 cycles under the conditions of catalyst amount 2.2 g per 100 g glycerol, molar ratio of acetone to glycerol 4, and reaction at 70 oC for 180 min, the glycerol conversion can keep a stable value of about 85%. It indicates that ZS-0.5 exhibits an excellent catalytic stability in the glycerol ketalization with acetone.
EXPERIMENTAL
CATALYSTS PREPARATION
ZrO2-SiO2 mixed oxides were synthesized by sol-gel method as follows: 22.3 mL tetraethyl orthosilicate (TEOS) as Si sources were added to 50 mL anhydrous EtOH. After the ample mixture, 50 mL distilled water and 10 mL AcOH were successively titrated under the vigorous stirring to form homogeneous solution A. Additionally, 10 mL AcOH were added into another aqueous solution containing a certain amount of ZrOCl2 and stirred for 30 min to obtain solution B. After finishing, solution B was introduced into solution A under stirring, thus a transparent sol solution would be obtained and subsequently aged for 24 h at room temperature to form gel. The gel was dried at 120 oC for 12 h and calcined at a desired temperature for 4 h. The white ZrO2-SiO2 mixed oxides powder materials with different Zr contents (denoted as ZS-X, wherein X was the Zr/Si molar ratio) would be prepared.
CATALYSTS CHARACTERIZATION
XRD patterns of catalysts were recorded on a DX-2700 powder diffractometer (Dandong, China) operated at 30 kV and 20 mA, using Cu Kα radiation. FT-IR spectra were recorded by using compressed KBr pellets containing 1 wt% of dry catalyst on a Shimadzu RF-IRPrestige-21 spectrophotometer in the range of 400-4000 cm-1. The surface acidity of catalyst was tested by NH3-TPD on a TP-5080 adsorption instrument (Tianjing, China): 0.2 g samples (0.38-0.83 mm) was loaded and pretreated with N2 at 400 oC for 1 h to remove the adsorbed water. The adsorption of NH3 and the removal of physically-adsorbed NH3 on catalyst surface were carried out at room temperature. The desorption of chemically-adsorbed NH3 was carried out by heating from 30 to 850 oC (heating rate of 10 oC·min-1) in a 30 mL·min-1 of Ar gas, the desorbed NH3 amount per gram catalyst, which was detected by the thermal conductivity detector (TCD), represented the total acidity.
CATALYTIC TEST
Glycerol catalytic ketalization with acetone was carried out in a 50 mL glass reactor fitted with a condenser, magnetic stirrer and thermostat. ZS-X mixed oxides as catalyst, glycerol and acetone were added successively into the reactor. The reaction started when the temperature reached up to the desired value. The catalytic test involved the effect of reaction temperature, acetone/glycerol molar ratio, catalyst amount and reaction time on ketalization. The unconverted glycerol and formed products were analyzed quantitatively by a GC-2000 gas chromatography (Chongqing, China) equipped a KB-Wax column (30 m ×0.32 mm ×0.5 um, Kromat Co., USA) and a flame ionization detector (FID). The qualitative analysis of the goal products was carried out on an HP 6890/5973 GC/MS gas chromatography-mass spectroscopy. The typical GC pattern of sample including acetone, glycerol, solketal and 5-ol was shown in Figure 2S of Supporting Information.
ACKNOWLEDGEMENTS
We are grateful to the Youth Science and Technology Foundation of Sichuan Province in China (Grant No. 2012JQ0047) for financial support of this project.
References
1. R. S. Karinen and A. O. I. Krause, Appl. Catal. A, 2006, 306, 128. CrossRef
2. E. P. Maris and R. J. Davis, J. Catal., 2007, 249, 328. CrossRef
3. T. Kurosaka, H. Maruyama, I. Naribayashi, and Y. Sasaki, Catal. Commun., 2008, 9, 1360. CrossRef
4. A. J. Byrd, K. K. Pant, and R. B. Gupta, Fuel, 2008, 87, 2956. CrossRef
5. D. A. Simonetti, E. L. Kunkes, and J. A. Dumesic, J. Catal., 2007, 247, 298. CrossRef
6. R. Sarkari, C. Anjaneyulu, V. Krishna, R. Kishore, M. Sudhakar, and A. Venugopal, Catal. Commun., 2011, 12, 1067. CrossRef
7. C. Q. Liu, C. H. Xu, T. W. Xia, Y. Guo, and J. Y. Liu, Heteroat. Chem., 2012, 23, 377. CrossRef
8. A. L. Maksimov, A. I. Nekhaev, D. N. Ramazanov, Y. A. Arinicheva, A. A. Dzyubenko, and S. N. Khadzhiev, Pet. Chem., 2011, 51, 61. CrossRef
9. L. N. Batista, R. A. S. S. Gil, and L. B. R. Lopes, J. Therm. Anal. Calorim., 2011, 106, 697. CrossRef
10. J. Deutsch, A. Martin, and H. Lieske, J. Catal., 2007, 245, 428. CrossRef
11. L. Roldan, R. Mallada, J. M. Fraile, J. A. Mayoral, and M. Menéndez, Asia-Pac. J. Chem. Eng., 2009, 4, 279.
12. P. Ferreira, I. M. Fonseca, A. M. Ramos, J. Vital, and J. E. Castanheiro, Appl. Catal. B, 2010, 98, 94. CrossRef
13. C. X. A. Silva, V. L. C. Goncalves, and C. J. A. Mota, Green Chem., 2009, 11, 38. CrossRef
14. T. Mitsudome, T. Matsuno, S. Sueoka, T. Mizugaki, K. Jitsukawa, and K. Kaneda, Heterocycles, 2012, 84, 371. CrossRef
15. R. S. Reddy, P. Sudarsanam, B. Mallesham, G. Raju, and B. M. Reddy, J. Ind. Eng. Chem., 2011, 17, 377. CrossRef
16. P. K. Doolin, S. Alerasool, D. J. Zalewski, and J. F. Hoffman, Catal. Lett., 1994, 25, 209. CrossRef
17. Y. Zhang, L. Pan, C. G. Gao, and Y. X. Zhao, J. Sol-Gel Sci. Technol., 2011, 58, 572. CrossRef
18. Y. Wang, J. H. Ma, D. Liang, M. M. Zhou, F. X. Li, and R. F. Li, J. Mater. Sci., 2009, 44, 6736. CrossRef
19. S. W. Wang, X. X. Huang, and J. K. Guo, J. Mater. Sci., 1997, 32, 197. CrossRef
20. C. N. Fan, C. H. Xu, C. Q. Liu, Z. Y. Huang, J. Y. Liu, and Z. X. Ye, React. Kinet. Mech. Catal., 2012, 107, 189. CrossRef
21. M. W. Jung, J. Sol-Gel Sci. Technol., 2000, 19, 563. CrossRef
22. Z. Q. Zhan and H. G. Zeng, J. Non-Cryst. Solids, 1999, 243, 26. CrossRef