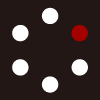
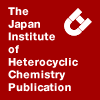
HETEROCYCLES
An International Journal for Reviews and Communications in Heterocyclic ChemistryWeb Edition ISSN: 1881-0942
Published online by The Japan Institute of Heterocyclic Chemistry
e-Journal
Full Text HTML
Received, 30th August, 2012, Accepted, 24th September, 2012, Published online, 2nd October, 2012.
DOI: 10.3987/COM-12-12576
■ Conversion of Two Eremophilane Glucosides from Pittocaulon praecox into Eremophilanolides
Amira Arciniegas, Jhon Ironzi Maldonado, Ana-L. Pérez-Castorena,* and Alfonso Romo de Vivar
Instituto de Química, Universidad Nacional Autónoma de México, Circuito Exterior, Ciudad Universitaria, Coyoacán 04510, D.F., Mexico
Abstract
Two eremophilanolides (3, 4), an aldehyde (6), an intramolecular addition product (7), and two water addition products (8, 9) were obtained by acid treatment of the eremophilane glucosides 10 and 11. A reaction mechanism supported by deuterium labeling is proposed for the conversion of eremophilane glycosides into eremophilanolides.INTRODUCTION
It is well established that the biogenesis of sesquiterpene lactones begins with the cyclization of farnesyl pyrophosphate giving rise to germacranes. The Markovnikov type cyclization of germacranes produces eudesmanes while the anti-Markovnikov cyclization gives guaianes. Eremophilanes are the result of the migration of the C-10 methyl group to C-5. Oxidation at C-12 and C-8 or C-6 affords germacranolides, eudesmanolides or eremophilanolides.1,2
Additionally, the furanoeremophilanes have been proposed as biogenetic precursors of eremophilanolides, and this transformation has been carried out by photosensitized oxygenation of some natural furanoeremophilanes.3-5 Since the formation of furan by oxidation of 2-butene-1,4-diol is known,6 the eremophilanes containing 1,4-dihydroxy or 1-hydroxy-4-carbonyl groups as 1 and 2 can be precursors of furanoeremophilanes and then of eremophilanolides.
Therefore, the glucosides 10 and 11, which were isolated from Pittocaulon praecox as part of our studies of the genus Pittocaulon,7 could be considered as intermediates in the biosynthesis of eremophilanolides.
In order to support this assumption and to establish a possible mechanism for the conversion of eremophilane glycosides into lactones, the hydrolysis of 10 and 11 in D2O with DCl was carried out.
RESULTS AND DISCUSSION
The glucosides 10 and 11 were hydrolyzed with 10% HCl at 70 °C for 30 minutes to afford in both cases the same pair of lactones 3 and 4. When the same reaction was carried out using 10% DCl in D2O they afforded lactone 5 (Scheme 1), the D-incorporated product corresponding to 4 was not isolated neither detected.
The hydrolysis at room temperature of compound 10 afforded the aldehyde 6 and that of compound 11 yielded the intramolecular addition product 7 and the water addition products 8 and 9 (Scheme 2).
The presence of the aldehyde 6 among the products of hydrolysis and specially of the deuterated lactone 5 allowed to propose the reaction mechanism shown in Scheme 3. In this, the keto-enol equilibria explain the presence of deuterium atoms at C-1 and C-9 in compound 5.
The structures of compounds 3-9 were elucidated by analysis of their spectroscopic data.
Compound 3, obtained as colorless prisms mp 140-142° (hexane-EtOAc), exhibited a molecular formula C15H22O3 by HRFABMS. In the UV spectrum showed a band at 220 nm and the IR displayed bands at 1743 and 1643 cm-1 which are typical of an α,β-conjugated γ-lactone. An additional band at 3473 cm-1 indicated the presence of a hydroxyl group. In the 1H NMR spectrum, the signals of an olefinic (3H, δ 1.80, dd, J = 2.0, 1.5 Hz), a secondary (3H, δ 1.08, d, J = 7.0 Hz) and a tertiary (3H, δ 0.6, s) methyl groups suggested an eremophilanolide profile. The α equatorial hydroxyl group at C-3 of compounds 10 and 11 was also present in 3 since H-3 appeared at δ 3.46 (ddd, J = 10.5, 10.5, 4.5 Hz), and correlated with CH3-14 in the NOESY experiment, indicating its β-axial orientation, since CH3-14 is oriented β on biogenetic grounds.2 The signal at δ 4.65 (1H, br dd, J = 12.0, 7.0 Hz) attributed to H-8 showed, besides the correlations with CH2-9, homoallylic couplings with CH3-13 in the COSY experiment. In addition, the fact that the secondary methyl group resonated at a lower field than the tertiary methyl group was consequent with a trans-annelation of rings A and B in 3.8 Moreover, the cross peaks observed in the NOESY experiment (Figure 1) of H-10 with H-4 and H-8 indicated the α orientation of these hydrogen atoms since on biogenetic grounds H-4 is α oriented.2
Compound 4 exhibited the same molecular ion m/z 250 and very similar spectroscopic data that compound 3. In the 1H NMR spectrum the tertiary methyl group resonated at lower field (δ 1.05, s) than the secondary methyl group (δ 0.99, d, J = 6.4 Hz), in opposite order of that of compound 3, indicating a cis decaline system.8 The NOESY experiment (Figure 1) showed cross peaks of CH3-14 with H-3β, Η-6β, and H-10 which also interacted with H-8, in agreement with structure 4. The physical and spectroscopic features of this compound were compared with those reported in the literature.5
Compound 5 showed the molecular formula C15D6H16O3 (HRFABMS). The absence, in the 1H NMR spectrum of 5 of the signals assigned to CH2-1, CH2-9, H-8, and H-10 in lactone 3 accounted for six deuterium atoms, the deuterium of the hydroxyl group should have been exchanged when the reaction mixture was washed with H2O. Moreover, the signals of CH2-2 (δ 2.05, dd, J = 12.5, 5.0 Hz, H-2a and δ 1.35, br t, J = 11.0 Hz, H-2b) appeared coupled only with each other and with H-3, in agreement with the presence of deuterium atoms at C-1, and in the 13C NMR spectrum the signals of CD-8 and CD-10 appeared as triplet and those of CD2-1 and CD2-9 as quintuplet. The position and multiplicity of the signals of the other hydrogen and carbon atoms remain unchanged with respect to the same groups in 3. Compound 6, obtained as yellow oil, exhibited in the IR spectrum bands of hydroxyl, and carbonyl groups (3508, 1721 and 1667 cm-1). In the 1H NMR spectrum the signal at δ 9.76 (br s) indicated the presence of a formyl group. The chemical shifts of the vinylic hydrogen H-9 (δ 5.78) and of the oxygenated methine H-3 (δ 3.62) signals were similar to the same signals in compound 10. In the higher field part of the spectrum, the signal at δ 1.16 (d, J = 7.2 Hz) was assigned to CH3-13 since it correlated with H-11 (δ 2.61, br qd, J = 7.2, 3.6 Hz) which also correlated with H-7 (δ 3.01, ddd, J = 13.5, 4.5, 3.6 Hz) in the COSY experiment. The formyl group was placed at C-11 by the cross peaks, observed in the HMBC experiment, between its proton and C-7, C-11 and C-13. The NOESY experiment showed that H-7 was oriented β by its correlations with CH3-14 which on biogenetic grounds are β oriented.2 The configuration of C-11 was not determined.
Compound 7 was obtained as white powder and showed bands in the IR spectrum of hydroxyl and conjugated carbonyl groups at 3356 and 1657 cm-1. It showed the same molecular formula (C21H32O8 by HRFABMS) and similar NMR spectroscopy to those of compound 11. Saturation of the 7(11) double bond was evident since in the 1H NMR spectrum H-7 appeared at δ 2.56 (dd, J = 13.5, 4.0 Hz) and CH3-13 at δ 1.30, and in the 13C NMR spectrum only two signals attributable to vynilic carbons were observed. The above was congruent with the intramolecular addition of the 2'-hydroxyl group of the glucose to the 7(11) double bond, additionally a NOESY interaction between H-2' and CH3-13 was observed, suggesting the α orientation of this methyl group and therefore the S configuration of C-11. The β-orientation of H-7 was determined by the interaction of this proton with CH3-14, observed in the same experiment.
Compounds 8 and 9 showed in the IR bands of hydroxyl and conjugated carbonyl groups (3396 and 1641 cm-1 in compound 8 and 3373 and 1655 cm-1 in 9) and in the UV spectrum absorption at 240 and 238 nm, respectively. They exhibited the same molecular formula C21H34O9 (HRESIMS) with 18 uma more than compound 11. In the 1H NMR spectra they showed the signals of H-7 at δ 3.01 in 8 and at δ 2.85 in 9 and in the 13C NMR spectra the respective C-11 signals appeared at δ 76.1 and 74.2, indicating that they are products of water addition to the 7(11) double bond of 11. The C-8 carbonyl group in compound 8 resonated at lower field (δ 205.2) and absorbed at lower frequency in the IR spectrum (1641 cm-1) than the same group in 7 (δ 202.6 and 1655 cm-1, respectively). The above is in agreement with the presence of a hydrogen bond between the carbonyl and the hydroxyl group in 8. Moreover, the difference between the chemical shifts of the signals of CH2-12 is Δδ 0.63 in 8, while in those of 9 is Δδ 0.07, thus indicating a restriction of the free rotation of C-11 and therefore of C-12 due to the chelation between the carbonyl and the hydroxyl group in compound 8. The NOESY experiment (Figure 2) of compound 8 showed the correlation of H-4 (δ 1.28) with H-6α (δ 1.45) and of Η-6β (δ 2.29) with H-12a (δ 4.02) which, in agreement with the Dreiding models, is compatible with an 11S configuration in 8 and therefore 11R in compound 9. The interaction between H-7 and CH3-14 observed in the NOESY of both compounds indicated that H-7 was β-oriented. This last interaction was observed, for compound 8, in an experiment taken in DMSO-d6 in order to distinguish between the resonances of CH3-14 (δ 1.04) and CH3-13 (δ 1.10).
EXPERIMENTAL
General experimental procedures. Melting points were determined on a Fisher-Jones melting point apparatus and are uncorrected. Optical rotations were determined on a Perkin-Elmer 343 polarimeter. UV and IR spectra were recorded on a Shimadzu UV 160U and a Bruker Tensor 27 spectrometer, respectively. 1D and 2D NMR spectra were obtained on a Varian-Unity Inova 500 MHz spectrometer with tetramethylsilane (TMS) as internal standard. EIMS data were determined on a Bruker Daltonics Analysis 3.2 mass spectrometer. HRFABMS were performed at 10.000 resolution using electric field scans and polyethylene glycol ions (Fluka 200 and 300) as reference material. HRESIMS were performed on a BrukermicrOTOF II mass spectrometer with mass resolution of 16.500 FWHM, mass interval 50-20.000 m/z, and speed 40 Hz. Column chromatography was carried out under vacuum (VCC) on silica gel G 60 (Merck, Darmstadt, Germany). Flash chromatography was performed on silica gel 60 (230-400 Macherey-Nagel). TLC was carried out on Si gel 60 and preparative TLC on Si gel GF254 (Merck), layer thickness 2.0 mm.
Acid Hydrolysis of Compounds 10 and 11: Compounds 10 and 11 (100 mg each) were separately heated at 70 ºC for 0.5 h in 10% HCl. The reaction mixtures were extracted with CH2Cl2, washed with H2O, and purified through respective flash column chromatographies eluted with hexane-EtOAc 7:3 to afford compounds 3 (7.5 mg from 10 and 10.5 mg from 11) and 4 (5.0 mg from 10 and 3.8 mg from 11).
Compounds 10 and 11 (100 mg each) were separately heated at 70 ºC for 0.5 h in 10% DCl. The reaction mixtures were extracted with CH2Cl2, washed with H2O, and purified through flash column chromatography eluted with hexane-EtOAc 6:4 to afford compound 5 (6.0 mg from 10 and 8.5 mg from 11).
Compound 10 (78 mg) was stirred with 10% HCl at room temperature for 2 h. The reaction mixture was extracted with CH2Cl2, washed with H2O, and purified through a flash column chromatography eluted with hexane-EtOAc 7:3 to afford compound 6 (18 mg).
Compound 11 (100 mg) was stirred with 10% HCl at room temperature for 16 h. The reaction mixture was neutralized to pH 7 with 5% KOH, liophylized and purified through a VCC eluted with EtOAc and EtOAc-MeOH 99:1 mixture to afford compound 7 (18 mg), 8 (5 mg) and 9 (7 mg).
Compound 3: Colorless needles (hexane-EtOAc); mp 140-142 °C; [α]D25 –157.0 (c 0.14, MeOH); UV (MeOH) λmax (log ε): 220 (2.4), 248 nm (4.2); IR (CHCl3): ν 3473, 1743, 1685 cm˗1; 1H NMR (CDCl3, 500 MHz): δ 4.65 (1H, brdd, J = 12.0, 7.0 Hz, H-8), 3.43 (1H, ddd, J = 10.5, 10.5, 5.0 Hz, H-3), 2.78 (1H, d, J = 13.5 Hz, H-6β), 2.16 (1H, ddd, J = 12.5, 7.0, 3.0 Hz, H-9α), 2.07 (1H, m, H-2a), 1.87 (1H, brd, J = 13.5 Hz, H-6α), 1.80 (3H, dd, J = 2.0, 1.5 Hz, H-13), 1.50 (1H, m, H-1a), 1.48 (1H, m, H-10), 1.37 (1H, m, H-2b), 1.35 (1H, m, H-1b), 1.30 (1H, dq, J = 10.5, 7.0 Hz, H-4), 1.25 (1H, ddd, J = 12.5, 12.5, 12.0 Hz, H-9β), 1.08 (3H, d, J = 7.0 Hz, H-15), 0.60 (3H, s, H-14); 13C NMR (CDCl3, 125 MHz): δ 174.7 (C, C-12), 160.4 (C, C-7), 122.1 (C, C-11), 80.0 (CH, C-8), 71.6 (CH, C-3), 50.2 (CH, C-4), 42.2 (CH, C-10), 40.8 (C, C-5), 38.7 (CH2, C-6), 35.9 (CH2, C-9), 35.6 (CH2, C-2), 26.9 (CH2, C-1), 11.6 (CH3, C-14), 10.5 (CH3, C-15), 8.1 (CH3, C-13). MS (EI, 70 eV) m/z (%): 250 [M]+ (20), 232 (25), 217 (30), 121 (100); HRMS (FAB+) m/z: 251.1651 (calcd. 251.1647 for C15H23O3, [M+H]+).
Compound 4: Colorless needles (hexane-EtOAc); mp 155-158 °C; [α]D25 +257.0 (c 0.1, CHCl3); IR (CHCl3): ν 3460, 1743, 1680 cm˗1; 1H NMR (CDCl3, 400 MHz): δ 4.65 (1H, brdd, J = 10.4, 7.6 Hz, H-8), 3.47 (1H, ddd, J = 10.8, 10.8, 5.6 Hz, H-3), 2.92 (1H, d, J = 14.0 Hz, H-6α), 2.16 (1H, ddd, J = 10.4, 6.8, 2.8 Hz, H-9α), 1.96 (1H, m, H-1β), 1.86 (1H, brd, J = 14.0 Hz, H-6β), 1.81 (3H, t, J = 1.6 Hz, H-13), 1.80 (1H, m, H-2a), 1.72 (1H, m, H-9β), 1.65 (1H, m, H-10), 1.47 (1H, m, H-1α), 1.44 (1H, m, H-2b), 1.31 (1H, m, H-4), 1.05 (3H, s, H-14), 0.99 (3H, d, J = 6.4 Hz, H-15); 13C NMR (CDCl3, 125 MHz): δ 174.7 (C, C-12), 160.2 (C, C-7), 121.0 (C, C-11), 80.1 (CH, C-8), 72.6 (CH, C-3), 40.1 (C, C-5), 39.5 (CH, C-10), 38.1 (CH, C-4), 36.5 (CH2, C-6), 34.9 (CH2, C-9), 30.2 (CH2, C-2), 24.9 (CH2, C-1), 22.9 (CH3, C-15), 11.0 (CH3, C-14), 8.3 (CH3, C-13). MS (EI, 70 eV) m/z (%): 250 [M]+ (100), 232 (20), 217 (35), 121 (15).
Compound 5: Colorless oil, 1H NMR (CDCl3, 500 MHz): δ 3.42 (1H, ddd, J = 10.0, 10.0, 5.0 Hz, H-3), 2.78 (1H, d, J = 13.5 Hz, H-6β), 2.05 (1H, dd, J = 12.5, 5.0 Hz, H-2a), 1.86 (1H, brd, J = 13.5 Hz, H-6α), 1.80 (3H, t, J = 1.5 Hz, H-13), 1.35 (1H, brt, J = 11.0 Hz, H-2b), 1.30 (1H, dq, J = 10.0, 6.5 Hz, H-4), 1.08 (3H, d, J = 6.5 Hz, H-15), 0.60 (3H, s, H-14); 13C NMR (CDCl3, 125 MHz): δ 174.7 (C, C-12), 160.3 (C, C-7), 122.1 (C, C-11), 79.5 (CD, t, C-8), 71.6 (CH, C-3), 50.1 (CH, C-4), 41.2 (CD, t, C-10), 40.7 (C, C-5), 38.7 (CH2, C-6), 35.4 (CH2, C-2), 35.1 (CD2, quint, C-9), 26.0 (CD2, quint, C-1), 11.5 (CH3, C-14), 10.6 (CH3, C-15), 8.1 (CH3, C-13). MS (EI, 70 eV) m/z (%): 256 [M]+ (25), 238 (20), 222 (40), 124 (100); HRMS (FAB+) m/z: 257.2028 (calcd. 257.2024 for C15D6H17O3, [M+H]+).
Compound 6: yellow oil; [α]D25 +69.0 (c 0.21, CHCl3); UV (MeOH) λmax (log ε): 238 nm (2.98); IR (KBr): ν 3508, 1721, 1667 cm˗1; 1H NMR, (CDCl3, 500 MHz): δ 9.76 (1H, br s, H-12), 5.78 (1H, d, J = 1.8 Hz, H-9), 3.62 (1H, ddd, J = 10.8, 10.8, 4.5 Hz, H-3), 3.01 (1H, ddd, J = 13.5, 4.5, 3.6 Hz, H-7), 2.61 (1H, br qd, J = 7.2, 3.6 Hz, H-11), 2.46 (1H, dddd, J = 15.0, 15.0, 5.1, 1.8 Hz, H-1a), 2.35 (1H, ddd, J = 15.0, 4.8, 2.7 Hz, H-1b), 2.16 (1H, dddd, J = 12.0, 5.1, 4.9, 2.7 Hz, H-2a), 1.98 (1H, dd, J = 13.5, 4.5 Hz, H-6a), 1.70 (3H, brt, J = 13.5 Hz, H-6b), 1.45 (1H, dddd, J = 15.0, 12.0, 10.8, 2.7 Hz, H-2b), 1.34 (1H, dq, J = 10.8, 6.9 Hz, H-4), 1.16 (3H, d, J = 7.2 Hz, H-13), 1.12 (3H, s, H-14), 1.08 (3H, d, J = 6.9 Hz, H-15); 13C NMR (CDCl3, 125 MHz): δ 204.1 (CH, C-12), 198.0 (C, C-8), 168.6 (C, C-10), 124.0 (CH, C-9), 70.9 (CH, C-3), 50.2 (CH, C-4), 45.4 (CH, C-11), 44.1 (CH, C-7), 40.0 (C, C-5), 39,1 (CH2, C-6), 35.0 (CH2, C-2), 31.0 (CH2, C-1), 17.2 (CH3, C-14), 10.3 (CH3, C-15), 9.6 (CH3, C-13); MS (EI, 70 eV) m/z (%): 250 [M]+ (4), 222 [M-28]+ (65), 175 (40), 121 (100); HRMS (FAB+) m/z: 251.1645 (calcd. 251.1647 for C15H23O3, [M+H]+).
Compound 7: Yellow oil; [α]D25 +45.4 (c 0.21, MeOH); UV (MeOH) λmax (log ε): 238 nm (3.98); IR (KBr): ν 3356, 1657 cm˗1; 1H NMR (CD3OD, 500 MHz): δ 5.64 (1H, d, J = 1.5 Hz, H-9), 4.63 (1H, d, J = 12.0 Hz, H-12a), 4.23 (1H, d, J = 8.0 Hz, H-1'), 3.86 (1H, dd, J = 12.0, 2.0 Hz, H-6'a), 3.70 (1H, dd, J = 12.0, 5.0 Hz, H-6'b), 3.56 (1H, ddd, J = 11.0, 10.5, 4.5 Hz, H-3), 3.54 (1H, d, J =12.0 Hz, H-12b), 3.42 (1H, t, J = 9.0 Hz, H-3'), 3.38 (1H, m, H-5'), 3.35 (1H, t, J = 9.0 Hz, H-4'), 3.25 (1H, dd, J = 9.0, 8.0 Hz, H-2'), 2.64 (1H, dd, J = 13.5, 4.0 Hz, H-6a), 2.56 (1H, dd, J = 13.5, 4.0 Hz, H-7), 2.48 (1H, dddd, J =14.5, 14.5, 5.0, 1.5 Hz, H-1a), 2.31 (1H, ddd, J =14.5, 4.5, 2.5 Hz, H-1b), 2.14 (1H, dddd, J = 12.5, 5.0, 4.5, 4.5 Hz, H-2a), 1.54 (1H, t, J = 13.5 Hz, H-6b), 1.38 (1H, dddd, J = 14.5, 12.5, 11.0, 2.5 Hz, H-2b), 1.30 (3H, s, H-13), 1.26 (1H, dq, J = 10.5, 7.0 Hz, H-4), 1.16 (3H, s, H-14), 1.09 (3H, d, J = 7.0 Hz, H-15); 13C NMR (CD3OD, 125 MHz): δ 200.7 (C, C-8), 171.3 (C, C-10), 125.2 (CH, C-9), 100.4 (CH, C-1'), 79.9 (CH, C-5'), 75.8 (CH2, C-12), 75.3 (CH, C-3'), 74.7 (C, C-11), 74.1 (CH, C-2'), 72.1 (CH, C-4'), 71.6 (CH, C-3), 62.6 (CH2, C-6'), 51.9 (CH, C-4), 51.2 (CH, C-7), 41.2 (C, C-5), 37.4 (CH2, C-6), 36.3 (CH2, C-2), 31.8 (CH2, C-1), 16.9 (CH3, C-14), 15.0 (CH3, C-13), 10.9 (CH3, C-15); MS (EI, 70 eV) m/z (%): 412 [M]+ (2), 250 [M-162]+ (35); HRMS (FAB+) m/z: 413.2174 (calcd. 4132175 for C21H33O8, [M+H]+).
Compound 8: Yellow oil; [α]D25 +33.5 (c 0.20, MeOH); UV (MeOH) λmax (log ε): 240 nm (3.1); IR (KBr): ν 3396, 1641 cm˗1; 1H NMR (CD3OD, 500 MHz): δ 5.74 (1H, d, J = 1.5 Hz, H-9), 4.30 (1H, d, J = 7.3 Hz, H-1'), 4.02 (1H, d, J = 10.5 Hz, H-12a), 3.92 (1H, dd, J = 11.5, 2.0 Hz, H-6'a), 3.63 (1H, dd, J = 11.5, 7.0 Hz, H-6'b), 3.56 (1H, ddd, J = 10.5, 10.5, 4.0 Hz, H-3), 3.39 (1H, d, J =10.5 Hz, H-12b), 3.38 (1H, t, J = 9.0 Hz, H-3'), 3.32 (1H, m, H-5'), 3.21 (1H, t, J = 9.0 Hz, H-4'), 3.20 (1H, t, J = 9.0 Hz, H-2'), 3.01 (1H, dd, J = 14.0, 4.0 Hz, H-7), 2.52 (1H, dddd, J = 14.5, 14.5, 5.0, 1.5 Hz, H-1a), 2.37 (1H, ddd, J = 14.5, 4.0, 2.5 Hz, H-1b), 2.29 (1H, dd, J = 13.0, 4.0 Hz, H-6β), 2.13 (1H, dddd, J = 12.5, 5.0, 4.5, 2.5 Hz, H-2a), 1.45 (1H, brt, J = 13.0 Hz, H-6α), 1.40 (1H, m, H-2b), 1.28 (1H, m, H-4), 1.19 (3H, s, H-14)*, 1.18 (3H, s, H-13)*, 1.10 (3H, d, J = 6.5 Hz, H-15), *Exchangeable signals; 13C NMR (CD3OD, 125 MHz): δ 205.2 (C, C-8), 173.1 (C, C-10), 125.3 (CH, C-9), 103.7 (CH, C-1'), 78.2 (CH, C-5'), 77.8 (CH, C-3'), 76.1 (C, C-11), 75.4 (CH, C-2'), 74.2 (CH2, C-12), 72.2 (CH, C-4'), 71.5 (CH, C-3), 63.4 (CH2, C-6'), 51.6 (CH, C-4), 47.1 (CH, C-7), 41.4 (C, C-5), 39.1 (CH2, C-6), 36.1 (CH2, C-2), 31.9 (CH2, C-1), 20.9 (CH3, C-13)*, 17.4 (CH3, C-14)*, 10.9 (CH3, C-15), *Exchangeable signals; MS (EI, 70 eV) m/z (%): 453 [M +Na]+ (65); HRESIMS m/z: 453.2083 (calcd. 453.2095 for C21H34NaO9, [M+Na]+).
Compound 9: Yellow oil; [α]D25 +9.0 (c 0.20, MeOH); UV (MeOH) λmax (log ε): 238 nm (2.98); IR (KBr): ν 3373, 1655 cm˗1; 1H NMR (CD3OD, 500 MHz): δ 5.64 (1H, d, J = 1.5 Hz, H-9), 4.21 (1H, d, J = 8.0 Hz, H-1'), 4.02 (1H, d, J = 11.0 Hz, H-12a), 3.95 (1H, d, J =11.0 Hz, H-12b), 3.86 (1H, dd, J = 12.0, 2.0 Hz, H-6'a), 3.64 (1H, dd, J = 12.6, 7.0 Hz, H-6'b), 3.55 (1H, ddd, J = 10.5, 10.5, 4.0 Hz, H-3), 3.39-3.24 (3H, m, H-3', H-4', H-5'), 3.18 (1H, dd, J = 9.0, 8.0 Hz, H-2'), 2.85 (1H, dd, J = 14.0, 4.0 Hz, H-7), 2.48 (1H, dddd, J = 14.5, 14.5, 5.0, 1.5 Hz, H-1a), 2.40 (1H, dd, J = 13.0, 4.0 Hz, H-6β), 2.31 (1H, ddd, J = 14.5, 4.0, 2.5 Hz, H-1b), 2.11 (1H, dddd, J = 12.5, 5.0, 4.5, 2.5 Hz, H-2a), 1.58 (1H, brt, J = 13.0 Hz, H-6α), 1.38 (1H, dddd, J = 14.5, 12.5, 10.5, 2.5 Hz, H-2b), 1.28 (1H. dq, J = 10.5, 6.5 Hz, H-4), 1.17 (3H, s, H-13), 1.12 (3H, s, H-14), 1.08 (3H, d, J = 6.5 Hz, H-15); 13C NMR (CD3OD, 125 MHz): δ 202.6 (C, C-8), 171.1 (C, C-10), 125.4 (CH, C-9), 105.1 (CH, C-1'), 78.1 (2CH, C-5', C-3'), 77.4 (CH2, C-12), 75.2 (CH, C-2'), 74.2 (C, C-11), 71.8 (CH, C-4'), 71.6 (CH, C-3), 62.8 (CH2, C-6'), 51.8 (CH, C-4), 47.8 (CH, C-7), 41.3 (C, C-5), 38.2 (CH2, C-6), 36.3 (CH2, C-2), 31.7 (CH2, C-1), 20.2 (CH3, C-13), 17.1 (CH3, C-14), 10.9 (CH3, C-15), MS (EI, 70 eV) m/z (%): 453 [M+Na]+ (65); HRESIMS m/z: 453.2087 (calcd. 453.2095 for C21H34NaO9, [M+Na]+).
ACKNOWLEDGEMENTS
We are indebted to Carmen Márquez, Eréndira García, Ma. Rocío Patiño, Beatríz Quiróz, Ma. Isabel Chávez, Héctor Ríos, Angeles Peña, Elizabeth Huerta, Nieves Zavala, Luis Velasco, Javier Pérez, Lizbeth Triana for technical assistance.
References
1. N. H. Fischer, E. J. Olivier, and H. D. Fisher, ‘The Biogenesis and Chemistry of Sesquiterpene Lactones,’ in Progress in the Chemistry of Organic Natural Products, Vol. 38, ed. by W. Herz, H. Guisebach, and G. W. Kirby, Springer-Verlag, New York, 1979, pp. 48-55.
2. J. Richards and J. Hendricsson, ꞌBiosynthesis of Terpenes, Steroids and Acetogenins,ꞌ W. A. Benjamin Inc., New York, 1964, pp. 225-237.
3. K. Naya, R. Kanazawa, and M. Sawada, Bull. Chem. Soc. Jpn., 1975, 48, 3220. CrossRef
4. K. Naya, N. Nogi, Y. Moriyama, H. Takashima, and T. Imagawa, Bull. Chem. Soc. Jpn., 1977, 50, 3002. CrossRef
5. K. Naya, M. Shimizu, H. Nishio, M. Takeda, S. Oka, and K. Hirota, Bull. Chem. Soc. Jpn., 1991, 64, 1071. CrossRef
6. C. C. Vu and L. A. Peterson, J. Label. Compd. Radiopharm., 2005, 48, 117. CrossRef
7. A. Arciniegas, A. L. Pérez-Castorena, E. Gastélum, J. L. Villaseñor, and A. Romo de Vivar, Heterocycles, 2009, 78, 1253. CrossRef
8. J. Jizba, Z. Zamek, L. Novortŷ, and E. Najdenova, Coll. Czech. Chem. Commun., 1978, 43, 1113. CrossRef