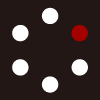
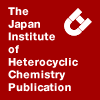
HETEROCYCLES
An International Journal for Reviews and Communications in Heterocyclic ChemistryWeb Edition ISSN: 1881-0942
Published online by The Japan Institute of Heterocyclic Chemistry
e-Journal
Full Text HTML
Received, 28th September, 2012, Accepted, 30th November, 2012, Published online, 6th December, 2012.
DOI: 10.3987/COM-12-12594
■ Glycosylated Methoxy-Macrolactins from a Marine Sediment Bacterium Bacillus subtilis
Hee Jae Shin,* Fakir Shahidullah Tareq, Ji Hye Kim, Min Ah Lee, Hyi-Seung Lee, Yeon-Ju Lee, and Jong-Seok Lee
Marine Natural Products Chemistry Laboratory, Korea Institute of Ocean Science & Technology, 787, Haeanro, Ansan 426-744, Korea
Abstract
Fermentation of a bacterium Bacillus subtilis isolated from the marine sediment sample collected at Gageocho, Republic of Korea in sea water based modified Bennett’s medium followed by double ethyl acetate (EA) extraction of the supernatant, sequential fractionations and purifications afforded three new glycosylated methoxy−macrolactins (1−3). The characterization of these compounds was achieved by various spectroscopic techniques including HRESIMS and extensive 1D and 2D NMR data analyses. The stereoconfiguration was assigned based on the chemical derivatization studies and literature data reviews. Methoxy−macrolactins were found to exhibit good antimicrobial properties when tested against bacteria and fungi. But, these compounds did not display any antiplasmodial activity against Plasmodium falciparum (D10).INTRODUCTION
To date, a number of pharmaceutically active compounds have been isolated from marine organisms and associated microorganisms with varying degrees of action, such as antibacterial, antifungal, anti-tumor, anti-cancer, anti-viral, cytotoxic, and immunosuppressive.1 Drug discovery research from marine organisms adjacent to terrestrial sources is increasing day by day and involves interdisciplinary research including biochemistry, biology, ecology, organic chemistry and pharmacology.2,3 Moreover, as the emergence of drug resistant pathogens is increasing enormously, there is a continuing interest on the microbial products by the pharmaceutical as well as agrochemical industries in the development of new therapeutic agents. Macrolactins are a group of 24-membered macrolides possessing potent antibacterial, antifungal and antiviral activities.4 Most of these macrolactins were derived as secondary metabolites from the marine microorganisms, while several of them were produced by some soil microorganisms as well. We have focused our attention on the isolation and biological activity investigation of secondary metabolites from the strain 109GGC020 that revealed 100% 16S rRNA sequencing similarity to B. subtilis. This bacterium was found to produce antibacterial, antifungal and antiviral compounds like peptides, macrolides, and unsaturated fatty acids.4-8 We found from these reviews that varieties of bioactive compounds have been isolated from the culture of the species B. subtilis at variable conditions and collected from different origins, which inspired us to advance further study on this species. And, in the course of our study three new glycosylated methoxy−macrolactins (1−3) were isolated and revealed as good antimicrobial agents.
RESULTS AND DISCUSSION
Identification of the isolated three new compounds was done based on spectroscopic data. Methoxy-macrolactin 1 was isolated as an amorphous solid and the molecular formula was determined to be C31H46O10 on the basis of high-resolution ESIMS showing a pseudomolecular ion peak at m/z 601.2983 [M + Na]+ in combination with 1H and 13C data. The IR absorption bands at 1733 cm−1 and 3374 cm−1 (brd.) suggested the presence of carbonyl and hydroxyl groups, respectively. Ultraviolet absorbencies at
234 and 261 nm were assigned to a chromophore with extended conjugation. The 1H and 13C NMR resonances (Table 1) with 1H−1H COSY and HSQC data revealed the presence of twelve sp2 olefinic methines, six sp3 methylenes, an sp3 methyl, four oxygenated methines, a methoxy, a lactone carbonyl carbon and a sugar moiety. These data analysis suggested that compound 1 belongs to the macrolactin family4 and possesses structural similarities to macrolactin B but differs from the molecular formula of macrolactin B by the addition of –CH3. In addition, the geometries of 1 were also found different from macrolactin B in the coupling constant values of olefinic protons. A methoxy group was figured out by a distinct singlet observed at δH 3.24 which was supported by the associated carbon resonated at δC 56.3 deduced from HSQC spectra. The attachment of the methoxy group was confirmed by the HMBC correlation of the methoxy proton at δH 3.24 to the carbon resonated at δC 81.9 (C-15). Additionally, an anomeric proton at δH 4.30 (H-1′) and carbon signals at δC 63.0, 78.2, 71.9, 78.3, 75.2 and 100.9 revealed the presence of β-glucopyranose moiety in 1.9-11 The position of the glucopyranosyl moiety at C-7 was confirmed by the HMBC correlation between the anomeric proton at δH 4.30 (H-1′) and the carbon at δC 78.2 (C-7). The presence of the hydroxy group at C-13 was also corroborated by HMBC correlations and by 1H–1H COSY correlations (Figure 2). The point of cyclization of the ester in macrolactone ring of 1 was indicated by the chemical shift of H-23 at δH 5.02, which was clearly coupled to the H3-24 methyl group and supported by an HMBC correlation (Figure 2) of H-23 with a carbonyl carbon at δC 168.1 (C-1). The connectivity of all other carbons and protons were established by COSY, HMBC and ROESY data. The configurations of the carbon-carbon double bonds (Z and E form) of 1 were assigned based on their 1H coupling constant values observed in CD3OD and ROESY correlations (Figure 4). The geometries of the conjugated dienes at C-2, C-4, C-8, C-10, C-16 and C-18 were determined to be Z, E, Z, Z, E and E, respectively on the basis of the coupling constants (J2,3 = 11.5 Hz; J4,5 = 15.0 Hz; J8,9 = 12.0 Hz; J10,11 = 11.5 Hz; J16,17 = 15.0 Hz; and J18,19 = 15.2 Hz) and ROESY correlations (H-2/H-3; H-3/H-5; H-8/H-9; H-10/H-11, H-9/H-12; H-16/H-18; H-17/H-19). The absolute configuration of 1 at selected stereocenters C-7 and C-13 was determined by acid hydrolysis, followed by Mosher’s MTPA method12-15 and assigned based on proton resonances of the S–MTPA and R–MTPA diester derivatives. A consistent distribution of positive and negative ΔδH values (ΔδH = δS − δR) around C-7 and C-13 allowed the assignment of S-configuration for C-7 and C-13 positions (Figure 3). Long range 1H-1H correlations between H-13 and H-15 and also between H-12 and H-15 were observed in ROE experiment, which were highly indicative of the syn relationship between H-13 and H-15 (Figure 3. (1c)). Consequently, the configuration of C-15 was considered to be R.15,16 The absolute configuration of glucopyranose moiety was ascribed to possess D-form by comparing the optical rotation value [0.5 mg, [α]23D +32 (c 0.3, H2O)] and TLC analysis (Rf = 0.48) with an authentic sample.17
The molecular formula of methoxy-macrolactin 2 was established as C31H46O10 based on high-resolution ESIMS (m/z 601.2982 [M + Na] +) in combination with 1H and 13C data. The IR absorption bands at 1685 cm−1 and 3341 cm−1 suggested the presence of carbonyl and hydroxyl moieties, respectively. The 1H and 13C NMR chemical shifts were found close to those of 1. The major differences between 1 and 2 were observed at the positions of the methoxy group and sp2 methines in 2. The long range HMBC correlation of the methoxy proton at δH 3.23 (-OCH3) to the oxygenated methine carbon at δC 81.9 confirmed that the methoxy group was attached at C-19. Furthermore, the sp2 methine carbons resonated at δC 130.1, 131.9, 133.3 and 130.9 were located at C-15, C-16, C-17 and C-18, respectively constituting 1H−1H COSY and HMBC correlations. Based on the coupling constant values and ROESY correlations, the geometries of the carbon-carbon double bonds at C-2, C-4 and C-8 were determined to be the same as 1. However, the direct measurement of the coupling constant between H-10 and H-11 was problematic because of the spectral overlap; nonetheless it was analyzed in conjunction with neighboring protons, and the clear ROESY correlation between H-9 and H-11, and the absence of ROE between H-9 and H-12 indicated the configuration of C-10 to be E (Figure 4). In addition, the geometries of the conjugated dienes at C-15 and C-17 were assigned as E by the combination of the large coupling constant values (J15,16 = 15.2 Hz and J17,18 = 15.0 Hz) and ROESY correlations between H-15 and H-17, H-16 and H-18, respectively. The absolute configuration of the selected stereocenters at C-7 and C-13 in 2 was considered to be the same as those of 1 as both compounds possess similar optical rotation values and 2 is likely either a biosynthetic precursor to 1 or they share a late stage biosynthetic intermediate.
The molecular formula of 3 was determined to be C31H46O10 based on high-resolution ESIMS (m/z 601.2982 [M + Na]+) in combination with 1H and 13C NMR data. The extensive 1D and 2D NMR spectra for methoxy−macrolactin 3 were similar to those of 1 and 2. Diagnostic 1H−1H COSY, HSQC, HMBC and ROESY correlations demonstrated that 3 was an analogue of 2. The differences between 2 and 3 were the geometries of the carbon-carbon double bonds at C-10 and C-17, deduced from the careful investigation of the vicinal 1H coupling constants and ROESY correlations. The small coupling constant value (J = 11.0 Hz) between H-10 and H-11 indicated the configuration of C-10 to be Z, which was also supported by the clear ROESY correlations (H-9/H-10 and H-10/H-11). In the similar manner, the coupling constant value (J17,18 = 9.5 Hz) and the ROE correlations (H-16/H-19 and H-17/H-18) confirmed the geometry of C-17 to be Z. The configurations of the double bonds at C-2, C-4, C-8, and C-15 of 3 were assigned to be Z, E, E and E, respectively based on the coupling constants (11.2, 15.5, 15.2 and 15.2 Hz, respectively) and ROESY correlations (Figure 4). The absolute stereochemistry of 3 at the stereocenters C-7 and C-13 was confirmed by the modified Mosher’s method as S, which is the same as those of 1 (S.I.). The acid hydrolysis product of compound 3 was analyzed to confirm that the glucopyranose of 3 possessed D-form, the same as those of 1 and 2.
The absolute stereochemistry at C-23 in compounds 1−3 was assumed to be R because of their similar 1H and 13C chemical shifts at the position and optical rotation values with the reported macrolactins.15,18 Moreover, these compounds were considered to be produced by a unique metabolic pathway and share the stereocenters between macrolactins A and B and their derivatives.4,19 Thus, compounds 1-3 were determined to be new derivatives of macrolactin B.
Minimum growth inhibitory activity of compounds 1−3 were evaluated against bacteria and fungi (Table 2). These compounds displayed good activity against both Gram positive and Gram negative bacteria and moderate antifungal activity. It may be noted that macrolactins usually showed antibacterial and moderate or weak antifungal activities.20-22 These three glycosylated methoxy−macrolactins (1−3) were found to exhibit no antiplasmodial activity when tested against P. falciparum.
EXPERIMENTAL
General. Optical rotations were measured on a JASCO (DIP-1000) digital polarimeter. UV spectra were obtained on a Shimadzu UV-1650PC spectrophotometer. IR spectra were recorded on a JASCO FT/IR-4100 spectrophotometer. Spectroscopic data were acquired on a Varian Unity 500 spectrometer. High-resolution ESIMS were recorded on a hybrid ion-trap time-of-flight mass spectrometer (Shimadzu LC/MS-IT-TOF). Analytical HPLC was conducted with a PrimeLine Binary pump with RI-101(Shodex) and Variable UV Detector (M 525). Semi-preparative HPLC was performed using ODS (YMC-Pack-ODS-A, 250 × 10 mm i.d, 5 µm) and silica (YMC-Pack-SIL, 250 × 10 mm i.d, 5 µm) columns. Analytical HPLC was conducted on an ODS column (YMC-Pack-ODS-A, 250 × 4.6 mm i.d, 5 µm). All solvents used were either spectral grade or distilled prior to use. Natural sea water was collected from East Sea of South Korea at a depth of 20 m.
Isolation and identification of the strain 109GGC020. The strain 109GGC020 was isolated from a sediment sample collected from Gageocho, Republic of Korea in 2010. To isolate pure strain one gram of the sediment sample was diluted in sterilized sea water (10-1, 10-2, 10-3 and 10-4) under aseptic conditions and 30 µL from each dilution was spread onto modified Bennett’s media agar plate (For 1 L 100% natural sea water media composition was 1% dextrose, 0.1% yeast extract, 0.1% beef extract, 0.2% tryptone, 1.8% agar and pH adjusted to 7.2). The plates were incubated for 6 days at 28 °C, and the resulting colony of the strain 109GGC020 was isolated and maintained on modified Bennett’s agar media plates. Finally, the strain was stocked into 40% glycerol in sea water and utilized for further analysis. The strain was identified as Bacillus subtilis on the basis of 16S rRNA sequence analysis (GenBank Accession no. JQ927413). This strain is currently preserved in the Microbial Culture Collection, KORDI, with the name of B. subtilis, ID-109GGC020.
Seed and large-scale cultures of the strain 109GGC020. The salinity and pH of seawater at the sampling site were 32.3 (g/L) and 8.01, respectively. Since, the physiological process of microorganisms depends on the surrounding conditions,23 the optimum growth condition of the strain was determined through culture in combination of different pH, salinity and temperature before proceeding of large scale culture. The salt concentration, pH and temperature were found 18.3 g/L, 7.02 and 24 °C, respectively for the optimum growth of the strain. The seed culture was carried out in modified Bennett’s medium (composition was same as above) into 100 mL flask (3 flasks) containing 50 mL medium. A single colony of the strain from the agar plate was inoculated aseptically into these flasks and incubated at 24 °C for 2 days on a rotary shaker at 120 rpm. An aliquot (0.2% v/v) from the seed culture was inoculated aseptically into 2 L flasks (total 50 flasks) containing 1 L sterilized culture medium. The production culture was incubated under optimum growth conditions for 7 days and then harvested.
Extraction and isolation of compounds. The culture broth (50 L) was centrifuged to separate cells from broth media and the broth was extracted with EA (2 × 60 L). The EA layer was evaporated to dryness under reduced pressure at 40 °C. The residual suspension (8.4 g) was subjected to an ODS open column chromatography followed by stepwise gradient elution with MeOH−H2O (v/v) (1:4, 2:3, 3:2, 4:1 and 100:0) as eluent. The fraction eluted with MeOH−H2O (3:2, v/v) again subjected to further fractionations by C18 MPLC to yield 10 subfractions. Semi-preparative silica HPLC (10% CHCl3 in MeOH; flow rate: 1.5 mL/min; detector: UV) was used to collect fractions from subfractions. Compounds 1−3 (0.04%, 0.02% and 0.03% of the crude extract, respectively) were then further purified on an analytical ODS HPLC (flow rate: 0.6 mL/min; detector: UV) from different fractions using the following isocratic programs: 65% MeOH in H2O; 60% MeOH in H2O.
Methoxy−macrolactin 1. Amorphous solid; [α]23D −175 (c 0.1, MeOH); UV (MeOH) λmax 234, 261 nm; IR (MeOH) νmax 3374 cm−1, 1733 cm−1; 1H and 13C NMR data, Table 1; HRESIMS m/z 601.2983 [M + Na]+ (calcd. for C31H46O10Na, 601.2989).
Methoxy−macrolactin 2. Amorphous solid; [α]23D −155 (c 0.1, MeOH); UV (MeOH) λmax 227, 256 nm; IR (MeOH) νmax 3341 cm−1, 1685 cm−1; 1H and 13C NMR data, Table 1; HRESIMS m/z 601.2982 [M + Na]+ (calcd. for C31H46O10Na, 601.2989).
Methoxy−macrolactin 3. Amorphous solid; [α]23D −205 (c 0.1, MeOH); UV (MeOH) λmax 232, 261 nm; IR (MeOH) νmax 3389 (br), 1681 cm-1; 1H and 13C NMR data, Table 1; HRESIMS m/z 601.2982 [M + Na]+ (calcd. for C31H46O10Na, 601.2989).
Absolute stereochemistry determination by Mosher’s method. Preparation of (S)−MTPA and (R)−MTPA diesters (1a and 1b) of 1. Compound 1 (1.9 mg) was hydrolyzed using 3N HCl for 6 h at 90 °C followed by EA extraction to obtained aglycon (1.4 mg). The aglycon was then dissolved in 150 µL pyridine and stirred at room temperature (rt) for 10 min. To the reaction vial, 20 µL (R)-(−)- α-methoxy-α-(trifluoromethyl)phenylacetyl chloride (MTPA−Cl) was added and the mixture was stirred at rt for 16 h for the preparation of (S)−MTPA esters (1a). Completion of the reaction was monitored by ESI-MS. The reaction mixture was dried in vacuo and redissolved in EA, washed with H2O, and purified on a C18 HPLC using 30% H2O in MeOH as eluent to obtain 1a (0.5 mg). All 1H signals were assigned by 1H−1H COSY experiment. In an entirely analogous way, (R)−MTPA ester (1b, 0.4 mg) was prepared using (S)-(+) –MTPA−Cl (1H NMR data, S.I.).
Preparation of (S) and (R)−MTPA diesters (3a and 3b) of 3. (S)−MTPA and (R)−MTPA diesters (3a and 3b) of 3 were prepared following the same procedure described above (1H NMR data, S.I.).
TLC comparison of the glucose in 1-3. After acid hydrolysis of compounds 1-3 at the above condition, the reation mixture was partitioned with EA/H2O. The aqueous layer was analyzed for the determination of the absolute configuration of the glucose in compounds 1-3. MeOH : EA (1:4) solvent system was used for TLC analysis of the glucose.17 Both authentic α- and β-D-glucopyranoses were run and Rf values were recorded.
Antibacterial and antifungal tests. Antimicrobial activity of compounds 1−3 were determined by using a standard ‘broth dilution assay’24 against Gram positive and Gram negative bacteria and fungi. Antibacterial and antifungal tests were performed in nutrient broth and yeast maltose broth, respectively. A serial double dilution of each compound was prepared in 96-microtiter plates over the range of 0.5−256 µg/mL. An overnight broth culture of each strain was prepared and final concentration of organisms in each culture was adjusted to 1.5 × 108 cfu/mL by comparing the culture turbidity with the 0.5 McFarland Standard. Culture broth (30 µL) was added to each dilution of compounds 1−3 and the final volume of each well was adjusted to 200 µL using the respective culture medium, and the plates were incubated 24 h at 37 °C for bacteria and 48 h at 30 °C for fungi.25,26 Finally, the minimum inhibitory concentration (MIC) was recorded following the lowest concentration of samples at which microorganisms did not demonstrate visible growth.
Antiplasmodial activity test. The antiplasmodial activity test was carried out as described earlier Frédérich.27 The activity was monitored using a chloroquine-sensitive strain of P. falciparum (D10). All experiments were performed in triplicate on a single occasion and repeated twice. In the assays, chloroquine and artemisinin were used as positive controls. The results were expressed as 50% inhibitory concentrations (IC50), representing the concentration of drug that induced, respectively, a 50% parasitemia decrease compared with the control culture. The parasites were continuously cultured according to the methods described by Trager and Jensen28 and the parasite lactate dehydrogenase activity was used as a measure of parasite viability.29 The IC50 values of chloroquine diphosphate (Sigma) and artemisinin, used as antiplasmodial references, were determined at concentrations of 0.01, 0.05, 0.1, and 1 µM. All compounds were also tested at four different concentrations (0.1, 1, 5 and 10 µM), and the results were expressed as 50% inhibitory concentrations (IC50), representing the concentration of drug that affords a 50% parasitemia decrease and cytotoxicity (SK-OV-3) compared with the control culture.
ACKNOWLEDGEMENTS
The authors express gratitude to Dr. C. Kun, Korea Basic Science Institute, Ochang, Korea, for providing HRESIMS data. This research was supported in part by the Ministry of Land, Transport and Maritime Affairs, Korea and Korea Institute of Ocean Science and Technology. (Grant PE98816 to H. J. S.)
References
1. I. Bhatnagar and S. K. Kim, Mar. Drugs., 2010, 8, 2673. CrossRef
2. R. J. Capon, Eur. J. Org. Chem., 2001, 4, 633. CrossRef
3. B. Haefner, Drug Discov. Today, 2003, 8, 536. CrossRef
4. K. Gustafson, M. Roman, and W. Fenical, J. Am. Chem. Soc., 1989, 111, 7519. CrossRef
5. J. S. Yoo, C. J. Zheng, S. Lee, J. H. Kwak, and W. G. Kim, Bioorg. Med. Chem. Lett., 2006, 16, 4889. CrossRef
6. K. Babasaki, T. Takao, Y. Shimonishi, and K. Kurahashi, J. Biochem., 1985, 98, 585.
7. M. A. Mondol, J. H. Kim, M. A. Lee, F. S. Tareq, H. S. Lee, Y. J. Lee, and H. J. Shin, J. Nat. Prod., 2011, 74, 1606. CrossRef
8. C. J. Zheng, S. Lee, C. H. Lee, and W. G. Kim, J. Nat. Prod., 2007, 70, 1632. CrossRef
9. K. Bock and C. Pederson, ‘Advances in Carbohydrate Chemistry and Biochemistry’, Vol. 41, ed. by R. S. Jipson and D. Horfon, Academic Press, Inc., New York, 1983, p. 27.
10. T. A. Mansoor, P. B. Shinde, X. Luo, J. Hong, C. O. Lee, C. J. Sim, B. W. Son, and J. H. Jung, J. Nat. Prod., 2007, 70, 1481. CrossRef
11. G. R. Pettit, Y. Tang, and J. C. Knight, J. Nat. Prod., 2005, 68, 974. CrossRef
12. I. Ohtani, T. Kusumi, Y. Kashman, and H. Kakisawa, J. Am. Chem. Soc., 1991, 113, 4092. CrossRef
13. A. Arnone, G. Nasini, and O. V. Pava, Phytochemistry, 1998, 48, 507. CrossRef
14. D. C. Oh, J. J. Scott, C. R. Currie, and J. Clardy, Org. Lett., 2009, 11, 633. CrossRef
15. M. A. Mondol, F. S. Tareq, J. H. Kim, M. A. Lee, H. S. Lee, Y. J. Lee, and H. J. Shin, J. Nat. Prod., 2011, 74, 2582. CrossRef
16. G. Ryu, W. B. Choi, and H. B. Lee, Bull. Korean Chem. Soc., 2002, 23, 1429. CrossRef
17. F. S. Tareq, J. H. Kim, M. A. Lee, H. S. Lee, Y. J. Lee, J. S. Lee, and H. J. Shin, Org. Lett., 2012, 14, 1464. CrossRef
18. M. A. Mondol, J. H. Kim, H. S. Lee, Y. J. Lee, and H. J. Shin, Bioorg. Med. Chem. Lett., 2011, 21, 3832. CrossRef
19. K. Schneider, X. H. Chen, J. Vater, P. Franke, G. Nicholson, R. Borriss, and R. D. Süssmuth, J. Nat. Prod., 2007, 70, 1417. CrossRef
20. C. Jaruchoktaweechai, K. Suwanborirux, S. Tanasupawatt, P. Kittakoop, and P. Menasveta, J. Nat. Prod., 2000, 63, 984. CrossRef
21. X. L. Lu, Q. Z. Xu, Y. H. Shen, X. Y. Liu, B. H. Jiao, W. D. Zhang, and K. Y. Ni, Nat. Prod. Res., 2008, 22, 342. CrossRef
22. C. Xue, L. Tian, M. Xu, Z. Deng, and W. Lin, J. Antibiot., 2008, 61, 668.
23. D. N. Thomas and G. S. Dieckmann, Science, 2002, 295, 641. CrossRef
24. G. Appendino, S. Gibbons, A. Giana, A. Pagani, G. Grassi, M. Stavri, E. Smith, and M. M. Rahman, J. Nat. Prod., 2008, 71, 1427. CrossRef
25. M. Oluwatuyi, G. W. Kaatz, and S. Gibbons, Phytochemistry, 2004, 65, 3249. CrossRef
26. J. Q. Yu, J. C. Lei, H. Yu, X. Cai, and G. L. Zou, Phytochemistry, 2004, 65, 881. CrossRef
27. M. Frédérich, M. De Pauw, C. Prosperi, M. Tits, V. Brandt, J. Penelle, M. Hayette, P. DeMol, and L. Angenot, J. Nat. Prod., 2001, 64, 12. CrossRef
28. W. Trager and J. B. Jensen, Science, 1976, 193, 673. CrossRef
29. M. T. Makler, J. M. Ries, J. A. Williams, J. E. Bancroft, R. C. Piper, B. L. Gibbins, and D. J. Hinrichs, Am. J. Trop. Med. Hyg., 1993, 48, 739.