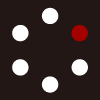
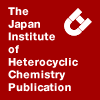
HETEROCYCLES
An International Journal for Reviews and Communications in Heterocyclic ChemistryWeb Edition ISSN: 1881-0942
Published online by The Japan Institute of Heterocyclic Chemistry
e-Journal
Full Text HTML
Received, 4th October, 2012, Accepted, 18th October, 2012, Published online, 29th October, 2012.
DOI: 10.3987/COM-12-12597
■ A Total Synthesis of Herboxidiene Methyl Ester
Rajaratnam Premraj, Malcolm D. McLeod, Gregory W. Simpson, and Martin G. Banwell*
Research School of Chemistry, Australian National University, Canberra, ACT 0200, Australia
Abstract
The total synthesis of the methyl ester, 35, of herboxidiene (1, a.k.a. GEX1A and TAN-1609), a polyketide displaying both herbicidal and anti-tumor activity, is described. The convergent reaction sequence involves, in its closing stages, the union of the phosphine oxide 3 with the aldehyde 21 to deliver, via a Horner–Wittig reaction with accompanying epimerization at C12, compound 33 that after deprotection affords an alcohol, 34, capable of participating in a regio- and diastereo-selective epoxidation reaction to give target 35. Phosphine oxide 3 was prepared via the intramolecular hetero-Michael addition of a secondary alcohol to a tethered and Z-configured acrylate while aldehyde 21 was generated, in the crucial step of the relevant reaction sequence, via an Ireland–Claisen rearrangement reaction.INTRODUCTION
In 1992 Issacs et al. reported1 the isolation of the polyketide herboxidiene (1) (a.k.a. GEX1A and TAN-1609) from Streptomyces sp. A7847 and five years later Edmunds and co-workers detailed2 the establishment of the absolute stereochemistry of the compound. Since this time five other structurally related compounds (in the so-called GEX1 series) have been isolated by Yoshida et al.3 from the culture broth of another Streptomyces sp. Herboxidiene was shown to exhibit selective phytotoxicity against a range of broadleaf annual weeds whilst being harmless to wheat.1,2 It also activates the LDL receptor,4 increases G1 and G2 cell phase populations5 and displays anti-tumor activity in various cell lines.3 Certain aspects of the mechanism of action responsible for the latter effects appear to have been elucidated recently by Mizukami et al.6 Specifically, this group has shown that herboxidiene targets the SAP155 protein, a subunit of SF3b responsible for pre-mRNA splicing and representing a novel target for anti-tumor drugs.
The significant biological properties and intriguing structure of herboxidiene have prompted a number of synthetic studies with the first total synthesis of the compound having been reported in 1999 by Kocienski and co-workers.7 Groups lead by Panek,8 Forsyth,9 Ghosh10 and Urpi11 have each reported distinctive syntheses in the interim, while Edmunds and his colleagues have described the preparation of a number of aromatic hybrids.12 It is against this background that we now provide full details of a total synthesis of herboxidiene methyl ester,13 the immediate precursor to compound 1 associated with Kocienski’s total synthesis7 of the natural product. The retrosynthetic analysis of target 1 employed in the current study is described in the following section.
Retrosynthetic Analysis
The key disconnection employed in the work described here was at C10/C11 and thus leading to side-chain and pyran-core fragments 2 and 3, respectively, that would be joined in an Horner–Wittig reaction to form the central diene unit of target 1 (Figure 1). Side-chain 2 was to be assembled via the Ireland–Claisen rearrangement14 of the propionate ester 4 which would, in turn, be prepared from the vinyl ketone 5. On the other hand, and based on earlier model studies,15 the all-equatorially substituted pyran core 6 was to be assembled through an intramolecular hetero-Michael addition reaction involving the ω-hydroxylated and Z-configured α,β-unsaturated ester 7. Elaboration of compound 6 to the phosphine oxide 3 would involve side-chain extension and Michaelis–Arbuzov16 reactions. The successful implementation of the approach defined by this retrosynthetic analysis is presented in the following sections.
RESULTS AND DISCUSSION
(i) Assembly of the Side-chain 2
The synthesis of the vinyl ketone precursor 5 to the side-chain compound 2 is shown in Scheme 1. Thus, the commercially available Roche ester 8 (>99% ee)17 was first converted into the corresponding and previously reported O-benzyl ether 9 (97%) under conditions that avoid racemization.18 Treatment of the latter compound with N,O-dimethylhydroxylamine hydrochloride in the presence of isopropylmagnesium chloride then afforded the Weinreb amide 10 (95%) which upon reaction with vinylmagnesium bromide gave the target ketone 5 in 92% yield.19 The spectral data derived from compound 5 were in full accord with the assigned structure while the observation of a specific rotation {[α]D} of +13.5 indicated that, at the very least, the material was enantiomerically enriched.
The route employed for the conversion of vinyl ketone 5 into the substrate (4) required for the proposed Ireland–Claisen rearrangement is shown in Scheme 2. After considerable experimentation, it was established that stereoselective reduction of ketone 5, so as to establish the required R-configuration at C17 in target 1, was best effected using zinc borohydride20 in dichloromethane. Using this reagent a ca. 4:1 mixture of the alcohols 11 and C17-epi-11 was obtained (quantitative combined yield).
Initially, the assignment of the illustrated stereochemistries to products 11 and C17-epi-11, which were readily separated from one another using preparative HPLC, was based on the proposition that a chelation-controlled reduction process21 is involved in the formation of the major product (Figure 2).
While the 1H and 13C NMR spectral data derived from alcohols 11 and C3-epi-11 matched those reported by Burke et al. for their respective enantiomers,22 the S-configuration of the newly created stereocenter within compound 11 was confirmed by converting it into its R- and S-Mosher esters and then undertaking appropriate comparisons23 of the derived 300 MHz 1H NMR data (see Table 2, Experimental for details).
O-Methylation of alcohol 11 using methyl iodide in the presence of potassium hydride afforded the allylic ether 12 (85%) that was then subjected to ozonolysis followed by reductive work-up with dimethylsulfide. In this way the aldehyde 13 was obtained in 72% yield. Due to its rather unstable nature, compound 13 was immediately treated with dimethylzinc in the presence of titanium tetrachloride24 at −78 °C and so generating, on an almost exclusive basis, the secondary alcohol 14 in 70% yield. The stereoselective nature of the transformation 13 → 14 presumably arises through the intermediacy of a chelate (Figure 3) in which titanium is simultaneously co-ordinated to the aldehyde carbonyl and the α-related methoxy group. Once again, the stereochemical outcome of this process was confirmed through a Mosher ester analysis of the same type as used (vide supra) to establish the structure of precursor 11 (see Table 3, Experimental for details).
Conversion of compound 14 into the corresponding tert-butyldimethylsilyl (TBDMS) ether 15 (80%) was readily achieved under standard conditions and the latter compound was subjected to hydrogenolysis using dihydrogen in the presence of Pearlman’s catalyst. In this manner the alcohol 16 was obtained in 97% yield. Oxidation of compound 16 using the Parikh–Doering reagent25 gave the corresponding but unstable aldehyde 17 (94%) which was immediately treated with the cuprate obtained by treating 2-bromopropene with tert-butyllithium then copper(I) bromide/dimethylsulfide.26 In this manner the allylic alcohol 18 was produced in 70% yield and as essentially the exclusive product of reaction. The stereoselective formation of compound 18 is, once again, attributed to the involvement of a chelation-controlled process. The completion of the synthesis of the substrate for the proposed Ireland–Claisen rearrangement involved acylation of alcohol 18 with propionic anhydride in the presence of 4-(N,N-dimethylamino)pyridine (DMAP) and pyridine. By such means the target ester 4 was obtained in 90% yield and the derived spectral data were in full accord with the assigned structure.
The pivotal Ireland–Claisen reaction14 was carried out under conditions defined by Smith et al.26 and involved initial treatment of the ester 4 with LDA in HMPA/tetrahydrofuran (THF) at –78 °C and the enolate so-formed was then intercepted with TBDMS-Cl and thereby generating the corresponding O-silyl ketene acetal. Thermally-induced rearrangement of this last species was effected at 50 °C and upon work-up the acid 19 incorporating the undesired configuration at C12 (herboxidiene numbering) was obtained. This outcome is attributed to the selective formation of the Z-silyl ketene acetal which engaged, via a chair-like transition state, in a [3,3]-sigmatropic rearrangement to give the observed compound 19 that possesses the unwanted R-configuration at C-12 but the required E-geometry about the Δ14,15-double bond. Since the stereochemistry at C12 could be inverted in a subsequent step of the reaction sequence (vide infra) compound 19 was carried through the synthetic sequence.27 Accordingly, acid 19 was subjected to lithium aluminium hydride-promoted reduction and thereby affording the corresponding primary alcohol 20 which was obtained in 66% yield after chromatographic purification.28 Treatment of the latter compound with the Dess–Martin periodinane (DMP)29 then afforded, in 62% yield, the corresponding aldehyde 21, the C12-epimer of the desired side-chain aldehyde 2.
(ii) Assembly of Pyran-core 3
Our second-generation synthesis13d of the pyranyl-containing core of herboxidiene is shown in Scheme 4 and used the commercially available alcohol 22 as staring material. This was oxidized, under Swern-type conditions, to the corresponding, previously reported30 and highly volatile aldehyde. The latter compound was immediately subjected to an olefination reaction31 using the ylide derived from ethyl bromoacetate and triphenylphosphine and thereby affording the E-configured α,β-unsaturated ester 23 in 98% yield (from alcohol 22). Diisobutylalumium hydride (DIBAl-H)-mediated reduction of compound 23 afforded the corresponding allylic alcohol 24 (85%) that was then subjected to Katsuki–Sharpless asymmetric epoxidation (KSAE)32 using the usual combination of reagents including diethyl D-(–)-tartrate as the chiral ligand. In this way the illustrated R,R-configured epoxy alcohol 25 was obtained in 70% yield and >95% ee as determined by Mosher ester analysis.13d
Reaction of compound 25 with trimethylaluminium in dichloromethane at 0 to 18 °C lead, via a chelation-controlled nucleophilic epoxide ring-opening reaction, to the methylated 1,2-diol 26 in 83% yield.33 The readily derived diacetate 27 (96%) was then subjected to ozonolysis followed by reductive work-up with triphenylphospine and so affording the aldehyde 28 in 79% yield. The spectral data derived from this last compound were identical, in all respects, with those obtained on a sample generated by a separate route.13c Subjection of compound 28 to the Still–Gennari modification34 of the Horner–Wadsworth–Emmons reaction using the anion derived from the commercially available bis(2,2,2-trifluoroethyl) (methoxycarbonylmethyl)phosphonate gave the α,β-unsaturated ester 29 in 80% yield. The Z-configuration about the double bond within this product follows from the observation of a 11.5 Hz coupling between the olefinic protons. In a pivotal conversion, diacetate 29 was subjected to reaction with potassium carbonate in methanol and so resulting in the formation of the pyran 6 incorporating cis-related and equatorial substituents at C3 and C7 (herboxidiene numbering). On the basis of our earlier studies of related cyclization processes,15 we believe the conversion 29 → 6 proceeds under kinetic control and that the Z-configuration about the double-bond of the Michael acceptor ensures the observed stereochemical outcome.
The elaboration of pyran 6 to the target building block 3 followed protocols related to those established by Nicolaou and Ley during the course of their syntheses of indanomycin (X-14547A).35 Thus, oxidation of the former compound with the Parikh–Doering reagent25 gave the corresponding aldehyde 30 in 60% yield. This proved spectroscopically identical with the material we had obtained by our earlier route.13c In light of its limited stability, compound 30 was immediately treated with methylmagnesium chloride. The resulting diastereoisomeric mixture of secondary alcohols was oxidized to the methyl ketone 31, albeit in just 17% yield, using DMP. Compound 31 corresponds to a degradation product derived from the ozonolysis of herboxidiene and the spectral data, including specific rotation, derived from our sample matched those reported by Edmunds et al.2 Reaction of compound 31 with vinylmagnesium bromide and treatment of the ensuing tertiary allylic alcohols (60%) with phosphorous tribromide then gave, in 93% yield, the rearranged primary allylic bromide 32 incorporating the required E-configured double bond. The latter compound was treated with diphenylethoxyphosphine and thus resulting in a Michaelis–Arbuzov reaction leading to the targeted and crystalline phosphine oxide 3 that was obtained in 85% yield.
With the required side-chain and core compounds, 21 and 3 respectively, in hand the proposed (Figure 1) coupling of these two building blocks using Horner–Wittig chemistry36 could be investigated. The outcomes of relevant studies are presented in the following section.
(iii) End-game – Union of Side-chain and Pyran-core
The foreshadowed linking of the herboxidiene subunits 3 and 21 involved treating the former compound (Scheme 5) with sodium hydride so as to form the corresponding C10 anion (herboxidiene numbering) and this was then reacted with the aldehyde 21 in THF at 55 °C. Cooling of the reaction mixture followed by work-up revealed that while the desired coupling reaction had taken place an accompanying saponification reaction had occurred and so giving, after acidic work-up, the C1 carboxylic acid rather than the methyl ester of the required coupling product. Accordingly the crude reaction mixture was treated with excess diazomethane. Analysis of the resulting esterified material revealed that it was composed of a 3:2 mixture of the desired compound 33 (38%) and its C12-epimer (25%) reflecting the stereochemistry of the starting aldehyde 21. The coupling products could be separated from one another by preparative HPLC and the major one (33) was then treated with tetra-n-butylammonium fluoride (TBAF) to give the alcohol 34 (68%) and thus setting up the possibility of a directed and diastereofacially-selective epoxidation of the Δ14,15-double-bond within this compound. In the event, and following a protocol established by Kocienski et al.7 during the closing stages of their synthesis of herboxidiene, compound 34 was treated, at –8 °C, with tert-butylhydroperoxide in the presence of VO(acac)237 and so providing herboxidiene methyl ester 35 (63%) together with very small amounts of what is tentatively identified as the diastereoisomeric epoxide which could be separated from its major co-product by semi-preparative HPLC.
The spectral data derived from compound 35 were consistent with those recorded by Kocienski et al.7 for the same compound. In particular, the chemical shifts observed in the 13C NMR spectra of the two materials match particularly well (Table 1). In contrast, an analogous comparison of these data with those reported by Panek for C-18-epi-35 reveals significant differences (see chemical shifts highlighted in bold) and suggests this technique provides a sensitive tool for detecting variations in the configuration of substituents along the herboxidiene framework. Since ester 35 has been hydrolyzed to the corresponding free acid, viz. to herboxidiene,7 the present work constitutes a formal total synthesis of the natural product itself.
CONCLUSION
The total synthesis of herboxidiene methyl ester (35) described herein exploits the Katsuki–Sharpless asymmetric epoxidation reaction, a chiral pool starting material and substrate-directed transformations for establishing the required stereochemistries associated with eight of the nine centers of chirality contained in the target molecule. The combination of such techniques has resulted in a reasonably concise formal total synthesis of herboxidiene and provides good prospects for acquiring useful quantities of this natural product and a range of analogues that could be exploited in developing a detailed understanding of the SAR associated with this class of compound.
EXPERIMENTAL
General Protocols
Proton (1H) and carbon (13C) NMR spectra were recorded on a Varian Gemini 300 spectrometer, operating at 300 MHz for proton and 75.4 MHz for carbon. All such spectra were recorded in deuterochloroform (CDCl3) solution at 22 °C. Chemical shifts are recorded as δ values in parts per million (ppm). For 1H NMR spectra recorded in deuterochloroform, the peak due to residual CHCl3 (δ 7.26) was used as the internal reference. Data are recorded as follows: chemical shift (δ), multiplicity (s : singlet, d : doublet, t : triplet, q : quartet, p : pentet, m : multiplet, dd : doublet of doublets etc., br : broad), coupling constant(s) (J Hz), relative integral (for proton spectra) and assignment (where possible). The digital resolution within spectra varies slightly depending on the selection of spectral width and number of data points allocated to the time domain. However, in all cases this is, at worst, of the order of 0.4 Hz/point for one dimensional spectra. The protonicities of the carbon atoms observed in 13C NMR spectra were determined by attached proton test (apt) experiments, i.e. by the phase of the resonance relative to the solvent signal, viz. C and CH2 were of the same phase as the CDCl3 “triplet”, whilst CH and CH3 resonances were of the opposite phase. The central peak (δ 77.0) of the CDCl3 triplet was used as the reference for {1H}13C NMR spectra. APT 13C NMR spectral data are recorded as follows: chemical shift (δ) and protonicity (C = quaternary, CH = methine, CH2 = methylene, CH3 = methyl, C/CH2 = quaternary or methylene, CH/CH3 = methine or methyl). Infrared spectra were recorded with either a Perkin–Elmer 683G Infrared Spectrophotometer or a Perkin–Elmer 1800 Series Fourier Transform Infrared Spectrophotometer. Samples were analyzed as thin liquid films on potassium bromide (KBr) plates. Low and high resolution electron-impact mass spectra were recorded at 70 eV on a VG Fisons AUTOSPEC three sector (E / B / E) double-focussing Mass Spectrometer, using positive-ion electron impact techniques (unless otherwise specified). Chemical ionization (CI) mass spectra were recorded on the VG Fisons AUTOSPEC spectrometer, with NH3 as the reactant gas. Mass spectral data are listed as mass-to-charge ratio (m/z), assignment (where possible) and relative intensity (% of base peak). Optical rotations were measured using a Perkin–Elmer 241 Polarimeter at the sodium D line (589 nm) with spectroscopic grade chloroform (CHCl3) as solvent (unless otherwise specified) at room temperature (ca. 22 °C) and concentration (c) (g/100 mL) indicated in a cell with a path length (l) of 1 dm. Specific rotations {[α]DT} were calculated using the equation: [α]DT = (100.α) / (l.c) and estimated to be within ± 0.05 × 10–1 deg cm2 g–1. Melting points were recorded with a Kofler hot stage apparatus and are uncorrected. Ozonolyses were performed using a Wallace and Tiernan Ozonator with the oxygen flow rate and power adjusted to approximately 25 L/h and 200 V, respectively. Analytical thin layer chromatography (TLC) was conducted on aluminum-backed 0.2 mm thick silica gel 60 F254 plates (Merck) and the chromatograms were visualized under a 254 nm UV lamp and/or by treatment with a reagent solution [either anisaldehyde/sulfuric acid/ethanol (EtOH) (2:5:93), phosphomolybdic acid/EtOH (8 g:200 mL) or phosphomolybdic acid/ceric (IV) sulfate/sulfuric acid/water (37.5 g:7.5 g:37.5 mL:720 mL)] followed by heating with a hair dryer or on a hot plate. Flash chromatography was conducted according to the method of Still and co-workers38 using 230–400 mesh silica and the analytical reagent (AR) grade solvents indicated. High performance liquid chromatography (HPLC) was conducted on a Waters µ-PorasilTM semi-preparative silica column (7.8 × 300 nm) connected to an ISCO Model 2350 pump and eluting with the indicated HPLC-grade solvents. The peaks were detected using a Waters Lambda-Max Model 481 UV Detector connected to a Spectra-Physics SP4270 Reporting Integrator. Many reagents were available from the Aldrich Chemical Company and were used as supplied. Drying agents and other inorganic salts were purchased from AJAX or BDH Chemicals. Reaction solvents and reagents were purified according to established procedures.39 The concentrations of alkyl lithium solutions obtained from Aldrich were determined by titration with sec-butanol (1.0 M solution in toluene) using 1,10-phenanthroline as indicator.40 Tetrahydrofuran (THF) and diethyl ether (Et2O) were dried with sodium and then distilled under nitrogen from sodium benzophenone ketyl. Benzene, toluene, dichloromethane (CH2Cl2), hexane and N,N-dimethylformamide (DMF) were distilled from calcium hydride. Pyridine was distilled from and stored over potassium hydroxide pellets. Diisopropylamine was refluxed over calcium hydride for 4 h then distilled under vacuum and stored under nitrogen. Dimethyl sulfoxide (DMSO) was dried with 4 Å molecular sieves, then distilled and stored over 4 Å molecular sieves. Triethylamine was refluxed over calcium hydride for 2 h then distilled from calcium hydride and stored over potassium hydroxide pellets. Reactions employing air- and/or moisture-sensitive reagents were carried out under an atmosphere of dry, oxygen-free nitrogen in oven- or flame-dried apparatus. Organic solutions obtained from work-up and/or extraction of reaction mixtures were dried with magnesium sulfate (MgSO4) unless otherwise specified. Solutions were concentrated under reduced pressure on a rotary evaporator with the water bath temperature generally not exceeding 30 °C. When reactions were conducted at, or below 0 °C, the internal temperature was monitored using an alcohol thermometer.
Specific Synthetic Conversions
Methyl (2S)-3-Benzyloxy-2-methylpropionate (9): Trifluoromethanesulfonic acid (3.40 mL, 38.1 mmol) was added, via syringe, to a magnetically stirred solution of methyl (2S)-3-hydroxy-2-methylpropionate (8) (15.0 g, 127 mmol, ex SIGMA) and benzyl 2,2,2-trichloroacetimidate (28.3 mL, 152.4 mmol) in a mixture of cyclohexane (200 mL) and CH2Cl2 (100 mL) maintained under a nitrogen atmosphere at 0 °C (ice bath). The reaction mixture was allowed to warm to room temperature, stirred for 16 h then filtered through a sintered glass funnel. The filtrate was washed with NaHCO3 (1 × 200 mL of a saturated aqueous solution) and water (1 200 mL) then dried, filtered and concentrated under reduced pressure. The oily residue thus obtained was subjected to flash chromatography (silica, 1:9 v/v ethyl acetate (EtOAc)/hexane elution) and concentration of the appropriate fractions (Rf 0.25) afforded the title compound 9 (25.6 g, 97%) as a clear, colorless oil, [α]D +12.5 (c 2.4); 1H NMR (300 MHz, CDCl3): δ 7.30–7.38 (complex m, 5H), 4.52 (s, 2H), 3.70 (s, 3H), 3.68 (m, 1H), 3.50 (dd, J 9.1 and 5.9 Hz, 1H), 2.81–2.75 (complex m, 1H), 1.18 (d, J 7.1 Hz, 3H); 13C NMR (75.5 MHz, CDCl3): δ 175.6 (C), 138.4 (C), 128.6 (CH), 127.9 (CH), 127.8 (CH), 73.3 (CH2), 72.2 (CH2), 52.0 (CH3), 40.4 (CH), 14.2 (CH3); IR (KBr): ṽmax 2862, 1740, 1455, 1201, 1098, 738, 699 cm–1; MS (70 eV): m/z (%): 208 (9) [M]+•, 177 (4) [M–CH3O•]+, 121 (16), 107 (53), 91 (100); HRMS (EI): m/z calcd for C12H16O3 [M]+•: 208.1099; found: 208.1096.
(2S)-3-Benzyloxy-N-methoxy-N-methyl-2-methylpropanamide (10): Isopropylmagnesium chloride (155.8 mL of a 2.0 M solution in THF, 311.5 mmol, ex ALDRICH) was added, steadily and over 0.5 h, to a magnetically stirred suspension of ester 9 (21.6 g, 103.8 mmol) and N,O-dimethylhydroxylamine hydrochloride (15.7 g, 161.0 mmol) in THF (230 mL) maintained under a nitrogen atmosphere at –15 °C. The resulting mixture was stirred for 0.5 h at –15 °C by which time TLC analysis revealed complete consumption of the starting material. At this point the reaction mixture was quenched with NH4Cl (150 mL of a saturated aqueous solution), diluted with water (150 mL) and extracted with Et2O (3 100 mL). The combined organic extracts were washed with brine (1 100 mL) then dried (MgSO4), filtered and concentrated under reduced pressure. The oily residue thus obtained was subjected to flash chromatography (silica, 3:7 v/v EtOAc/hexane elution) and concentration of the appropriate fractions (Rf 0.3) afforded the Weinreb amide 10 (23.4 g, 95%) as a clear, colorless oil, [α]D +4.62 (c 2.0); 1H NMR (300 MHz, CDCl3): δ 7.34–7.27 (complex m, 5H), 4.52 (ABq, J 12.1 Hz, 2H), 3.72 (app. t., J 8.6 Hz, 1H) 3.70 (s, 3H), 3.43 (dd, J 8.8 and 5.9 Hz, 1H), 3.25 (m, 1H), 3.22 (s, 3H), 1.12 (d, J 7.1 Hz, 3H); 13C NMR (75.5 MHz, CDCl3): δ 175.8 (C), 138.3 (C), 128.2 (CH), 127.5 (CH), 127.4 (CH), 73.1 (CH2), 72.5 (CH2), 61.5 (CH3), 35.8 (CH), 32.2 (CH3), 14.2 (CH3); IR (KBr): ṽmax 2862, 1660, 1454, 1102, 994, 739, 699 cm−1; MS (70 eV): m/z (%): 238 (11) [M+H]+, 206 (11) [M–CH3O•]+, 177 (25), 131 (70), 91 (100), 77 (44), 65 (58); HRMS (EI): m/z calcd for C13H20NO3 [M+H]+: 238.1443; found: 238.1442.
(2S)-1-Benzyloxy-2-methyl-4-penten-3-one (5): Vinylmagnesium bromide (126.4 mL of a 1.0 M solution in THF, 126.4 mmol, ex ALDRICH) was added, dropwise, to a magnetically stirred solution of Weinreb amide 10 (20.0 g, 84.3 mmol) in THF (833 mL) maintained under a nitrogen atmosphere at 0 °C (ice bath). The reaction mixture was stirred for a further 0.5 h at this temperature then quenched with NH4Cl (450 mL of a saturated aqueous solution). The aqueous layer was extracted with Et2O (3 250 mL) and the combined organic extracts were washed with brine (1 300 mL) then dried, filtered and concentrated under reduced pressure. The oily residue thus obtained was subjected to flash chromatography (silica, 1:4 v/v EtOAc/hexane elution) and concentration of the appropriate fractions (Rf 0.5) afforded the title ketone 5 (15.8 g, 92%) as a pale-yellow oil, [α]D +13.5 (c 2.8); 1H NMR (300 MHz, CDCl3): δ 7.35–7.25 (complex m, 5H), 6.45 (dd, J 17.5 and 10.4 Hz, 1H), 6.25 (dd, J 17.5 and 1.5 Hz, 1H), 5.79 (dd, J 10.4 and 1.5 Hz, 1H), 4.49 (ABq, J 12.1 Hz, 2H), 3.70 (dd, J 9.2 and 8.4 Hz, 1H), 3.47 (dd, J 9.2 and 5.9 Hz, 1H), 3.18 (m, 1H), 1.12 (d, J 7.0 Hz, 3H); 13C NMR (75.5 MHz, CDCl3): δ 202.5 (C), 138.1 (CH2), 135.5 (CH), 128.4 (C), 128.3 (CH), 127.5 (CH), 73.2 (CH2), 72.0 (CH2), 43.6 (CH), 13.9 (CH3) (one signal obscured or overlapping); IR (KBr): ṽmax 2859, 1697, 1678, 1454, 1198, 1101, 1028, 737, 698 cm–1; MS (70 eV): m/z (%): 205 (10) [M+H]+, 107 (35), 98 (75), 91 (100), 83 (48), 77 (25), 65 (35); HRMS (EI): m/z calcd for C13H17O3 [M+H]+: 205.1229; found: 205.1226.
(3S,4S)-4-Methyl-5-(phenylmethoxy)-1-penten-3-ol (11) and (3R,4S)-4-methyl-5-(phenylmethoxy)-1-penten-3-ol (C17-epi-11): Zinc borohydride (318.1 mL of a 0.43 M solution in Et2O, 136.8 mmol, which was prepared from zinc chloride and sodium borohydride20) was added, dropwise, to a magnetically stirred solution of vinyl ketone 5 (18.6 g, 91.2 mmol) in CH2Cl2 (890 mL) maintained under a nitrogen atmosphere at –78 °C. The resulting mixture was stirred for 2 h at –78 °C then warmed to 0 °C and stirred at this temperature for a further 0.5 h. The reaction was then quenched by transferring the reaction mixture, via cannula, to a rapidly stirred solution of HCl (600 mL of a 1 M aqueous solution) maintained at 0 °C. The aqueous layer was extracted with CH2Cl2 (3 250 mL) and the combined organic extracts were washed successively with NaHCO3 (1 250 mL of a saturated aqueous solution) and brine (1 250 mL) then dried (MgSO4), filtered and concentrated under reduced pressure. The ensuing oily residue was subjected to HPLC (µ-Porasil 40 × 100 mm cartridge column, 1:9 v/v EtOAc/hexane elution, flow rate 30 mL/min) and two fractions, A and B, were obtained.
Concentration of fraction A (Rt 12 min) afforded compound 11 (15.4 g, 82%) as a clear, colorless oil, [α]D –9.6 (c 2.8); 1H NMR (300 MHz, CDCl3): δ 7.39–7.28 (complex m, 5H), 5.88 (ddd, J 17.2, 10.6 and 5.4 Hz, 1H), 5.33–5.16 (complex m, 2H), 4.51 (s, 2H), 4.28–4.25 (complex m, 1H), 3.56–3.49 (complex m, 2H), 3.0 (br s, 1H), 2.12–2.05 (complex m, 1H), 0.91 (d, J 7.0 Hz, 3H); 13C NMR (75.5 MHz, CDCl3): δ 138.5 (CH), 137.9 (CH2), 128.3 (CH), 127.6(1) (CH), 127.5(5) (CH), 115.0 (C), 75.0 (CH), 73.6 (CH2), 73.3 (CH2), 38.2 (CH), 11.5 (CH3); IR (KBr): ṽmax 3440, 2966, 1454, 1097, 737, 698 cm–1; MS (70 eV): m/z (%): 206 (2) [M]+•, 188 (3), 144 (6), 108 (46), 91 (100); HRMS (EI): m/z calcd for C13H18O2 [M]+•: 206.1307; found: 206.1310.
Concentration of fraction B (Rt 16.5 min) afforded compound C3-epi-11 (3.40 g, 18%) as a clear, colorless oil, [α]D +28.2 (c 2.5); 1H NMR (300 MHz, CDCl3): δ 7.35–7.29 (complex m, 5H), 5.88 (ddd, J 17.2, 10.6 and 5.4 Hz, 1H), 5.29–5.13 (complex m, 2H), 4.52 (s, 2H), 4.03 (t, J 6.7 Hz, 1H), 3.62 (dd, J 9.3 and 4.3 Hz, 1H), 3.47 (dd, J 9.3 and 7.2 Hz, 1H), 3.37 (br s, 1H), 1.91 (m, 1H), 0.91 (d, J 7.1 Hz, 3H); 13C NMR (75.5 MHz, CDCl3): δ 139.7 (CH), 138.1 (CH2), 128.7 (CH), 128.0 (CH), 127.9 (CH), 116.1 (C), 76.9 (CH), 74.8 (CH2), 73.7 (CH2), 38.8 (CH), 14.0 (CH3); IR (KBr): ṽmax 3430, 2962, 2857, 1454, 1097, 737, 698 cm–1; MS (70 eV): m/z (%): 206 (4) [M]+•, 188 (5), 144 (8), 108 (50), 91 (100), 82 (55); HRMS (EI): m/z calcd for C13H18O2 [M]+•: 206.1307; found: 206.1308.
(3R,4S)-4-Methyl-5-(phenylmethoxy)-1-penten-3-yl (2R)-2-methoxy-2-phenyl-2-trifluoromethyl-acetate [(R)-Mosher ester of compound 11]: Oxalyl chloride (12.5 µL, 0.14 mmol) then DMF (1 drop) were added to a magnetically stirred solution of (R)-(+)-MTPA (74 mg, 0.32 mmol) in CH2Cl2 (2 mL) maintained at 0 °C (ice bath) under a nitrogen atmosphere. The reaction mixture was allowed to warm to room temperature, stirred for a further 0.75 h and then transferred, via cannula, to a chilled (0 °C) and magnetically stirred solution of the alcohol 11 (24 mg, 0.12 mmol), triethylamine (34 µL, 0.24 mmol) and DMAP (2 mg) in CH2Cl2 (3 mL). The cold bath was removed and the reaction mixture was stirred at room temperature for 16 h. The solution was then partitioned between CH2Cl2 (10 mL) and NaHCO3 (10 mL of a saturated aqueous solution) and the phases separated. The aqueous layer was extracted with CH2Cl2 (3 × 10 mL) and the combined organic fractions were washed with brine (1 × 10 mL) then dried, filtered and concentrated under reduced pressure to give a pale-yellow oil. Subjection of the crude material to flash chromatography (silica, 1:4 v/v EtOAc/hexane elution) provided, after concentration of the appropriate fractions (Rf 0.4), the title ester (27 mg, 55%) as a clear, colorless oil. 1H NMR (300 MHz, CDCl3): δ 7.60–7.30 (complex m, 10H), 5.84 (ddd, J 17.2, 10.4 and 7.0 Hz, 1H), 5.67 (dd, J 7.1 and 4.4 Hz, 1H), 5.30 (m, 2H), 4.50–4.33 (m, 2H), 3.53 (s, 3H), 3.26 (dd, J 6.1 and 2.7 Hz, 2H), 2.10 (m, 1H), 0.90 (d, J 6.9 Hz, 3H).
(3R,4S)-4-Methyl-5-(phenylmethoxy)-1-penten-3-yl (2S)-2-methoxy-2-phenyl-2-trifluoromethylacetate [(S)-Mosher ester of compound 11]: Oxalyl chloride (25 µL, 0.29 mmol) then DMF (1 drop) were added to a magnetically stirred solution of (S)-(–)-MTPA (120 mg, 0.51 mmol) in CH2Cl2 (2 mL) maintained at 0 °C (ice bath) under a nitrogen atmosphere. The reaction mixture was allowed to warm to room temperature, and stirred for a further 0.75 h and then transferred, via cannula, to a chilled (0 °C) and magnetically stirred solution of the alcohol 11 (50 mg, 0.24 mmol), triethylamine (68 µL, 0.48 mmol) and DMAP (2 mg) in CH2Cl2 (3 mL). The cold bath was removed and the reaction mixture was stirred at room temperature for 16 h. The solution was then partitioned between CH2Cl2 (10 mL) and NaHCO3 (10 mL of a saturated aqueous solution) and the phases separated. The aqueous layer was extracted with CH2Cl2 (3 × 10 mL) and the combined organic fractions were washed with brine (1 × 10 mL) then dried, filtered and concentrated under reduced pressure to give a pale-yellow oil. Subjection of the crude material to flash chromatography (silica, 1:4 v/v EtOAc/hexane elution) provided, after concentration of the appropriate fractions (Rf 0.4), the title compound (48 mg, 47%) as a clear, colorless oil. 1H NMR (300 MHz, CDCl3): δ 7.52–7.30 (complex m, 10H), 5.76 (dd, J 10.6 and 4.0 Hz, 1H), 5.67 (complex m, 1H), 5.24 (d, J 16.5 Hz, 1H), 5.23 (s, 1H), 4.50 (ABq, J 14.3 Hz, 2H), 3.50 (s, 3H), 3.34 (d, J 6.6 Hz, 2H), 2.12–2.08 (complex m, 1H), 0.96 (d, J 7.0 Hz, 3H).
(3S,4S)-3-Methoxy-4-methyl-5-(phenylmethoxy)-1-pentene (12): A solution of alcohol 11 (4.50 g, 21.8 mmol) in THF (20 mL) was added, dropwise, to a magnetically stirred suspension of KH (6.3 g of 35% (w/w) dispersion in mineral oil, 54.6 mmol) in THF (150 mL) maintained under a nitrogen atmosphere. The resulting mixture was stirred at room temperature for 0.25 h then chilled (0 °C) and treated, via syringe, with a solution of methyl iodide (3.4 mL, 54.6 mmol) in THF (25 mL). The reaction mixture was then allowed to warm to room temperature and stirred for a further 0.25 h then cautiously poured onto ice (50 g). The resulting mixture was extracted with Et2O (3 200 mL) and the combined organic phases were dried, filtered and concentrated under reduced pressure. The ensuing oily residue was subjected to flash chromatography (silica, 1:9 v/v EtOAc/hexane elution) and concentration of the appropriate fractions (Rf 0.6) afforded the title bis-ether 12 (4.10 g, 85%) as a clear, colorless oil, [α]D +12.2 (c 1.3); 1H NMR (300 MHz, CDCl3): δ 7.35–7.27 (complex m, 5H), 5.69 (ddd, J 16.5, 10.9 and 7.7 Hz, 1H), 5.24–5.17 (complex m, 2H), 4.50 (s, 2H), 3.62 (t, J 7.0 Hz, 1H), 3.51 (dd, J 8.1 and 4.5 Hz, 1H), 3.31 (dd, J 9.0 and 6.4 Hz, 1H), 3.27 (s, 3H), 1.94–1.85 (complex m, 1H), 0.96 (d, J 7.1 Hz, 3H); 13C NMR (75.5 MHz, CDCl3): δ 138.7 (C), 137.1 (CH), 128.3 (CH), 127.6 (CH), 127.5 (CH), 117.4 (CH2), 83.5 (CH), 73.1 (CH2), 72.4 (CH2), 56.8 (CH3), 38.5 (CH), 12.2 (CH3); IR (KBr): ṽmax 2902, 2856, 1454, 1095, 925, 736, 698 cm–1; MS (70 eV): m/z (%): 220 (2) [M]+•, 205 (2), 188 (1), 129 (16), 112 (16), 91 (83), 82 (50), 71 (100), 67 (24); HRMS (EI): m/z calcd for C14H20O2 [M]+•: 220.1463; found: 220.1464.
(2R,3S)-2-Methoxy-3-methyl-4-(phenylmethoxy)butanal (13): A magnetically stirred solution of alkene 12 (2.0 g, 9.1 mmol) in CH2Cl2 (200 mL) was cooled to −78 °C then treated with a stream of ozone gas until a blue color persisted. The reaction mixture was then warmed to –30 °C and dimethyl sulfide (DMSO) (1.3 mL, 18.2 mmol) added in small portions. The reaction mixture was allowed to warm to room temperature and the solvent was removed under reduced pressure. The residue thus obtained was subjected to flash chromatography (silica, 1:9 v/v EtOAc/hexane elution) and concentration of the appropriate fractions (Rf 0.4) afforded the title aldehyde 13 (1.45 g, 72%) as a clear, colorless, but very unstable oil, [α]D +22.5 (c 2.2); 1H NMR (300 MHz, CDCl3): δ 9.68 (d, J 1.5 Hz, 1H), 7.35–7.29 (complex m, 5H), 4.50 (ABq, J 12.0 Hz, 2H), 3.72 (m, 1H), 3.45 (m, 2H), 3.43 (s, 3H), 2.31 (m, 1H), 0.91 (d, J 7.1 Hz, 3H); 13C NMR (75.5 MHz, CDCl3): δ 203.8 (CH), 138.1 (C), 128.4 (CH), 127.6 (CH), 86.5 (CH), 73.0 (CH2), 71.0 (CH2), 58.9 (CH3), 35.6 (CH), 11.5 (CH3) (one signal obscured or overlapping); IR (KBr): ṽmax 2934, 1732, 1454, 1204, 1091, 738, 699 cm–1; MS (70 eV): m/z (%): 193 (18) [M–HCO•]+, 131 (18), 107 (40), 91 (100), 71 (24).
(2R,3R,4S)-3-Methoxy-4-methyl-5-(phenylmethoxy)pentan-2-ol (14): A magnetically stirred solution of the aldehyde 13 (1.0 g, 4.5 mmol) in CH2Cl2 (45 mL) was cooled to –78 °C then treated with neat TiCl4 (0.5 mL, 4.5 mmol). After 0.08 h, (Me)2Zn (2.25 mL of 2.0 M solution in THF, 4.5 mmol) was added. The resulting mixture was stirred at –78 °C for 0.25 h then water was cautiously added at −78 °C and the reaction mixture allowed to warm to room temperature and extracted with CH2Cl2 (3 100 mL). The combined organic extracts were washed with brine (1 25 mL) before being dried, filtered and then concentrated under reduced pressure. The oily residue thus obtained was subjected to flash chromatography (silica, 3:7 v/v EtOAc/hexane elution) and concentration of the appropriate fractions (Rf 0.25) afforded the title compound 14 (0.75 g, 70%) as a clear, colorless oil, [α]D +8.6 (c 2.8); 1H NMR (300 MHz, CDCl3): δ 7.35–7.31 (complex m, 5H), 4.51 (ABq, J 12.0 Hz, 2H), 3.80 (m, 1H), 3.48 (s, 3H), 3.44 (d, J 6.4 Hz, 2H), 3.07 (m, 1H), 2.60 (br s, 1H), 2.10–2.03 (complex m, 1H), 1.19 (d, J 6.4 Hz, 3H), 0.92 (d, J 7.0 Hz, 3H); 13C NMR (75.5 MHz, CDCl3): δ 138.1 (C), 128.4 (CH), 127.7 (CH), 127.6 (CH), 86.4 (CH), 73.1 (CH2), 72.6 (CH2), 67.9 (CH), 61.0 (CH3), 35.2 (CH), 19.4 (CH3), 11.8 (CH3); IR (KBr): ṽmax 3451, 2972, 1454, 1365, 1090, 737, 698 cm–1. MS (70 eV): m/z (%): 238 (1) [M]+•, 193 (21), 131 (20), 107 (13), 91 (100), 87 (40), 71 (24); HRMS (EI): m/z calcd for C14H22O3 [M]+•: 238.1569; found: 238.1560.
(2R,3R,4S)-3-Methoxy-4-methyl-5-(phenylmethoxy)pentan-2-yl (2R)-2-methoxy-2-phenyl-2-trifluoromethylacetate [(R)-Mosher ester of compound 14]: Oxalyl chloride (11.5 µL, 0.13 mmol) then DMF (1 drop) were added to a magnetically stirred solution of (R)-(+)-MTPA (31 mg, 0.13 mmol) in CH2Cl2 (0.5 mL) maintained at 0 °C (ice bath) under a nitrogen atmosphere. The reaction mixture was allowed to warm to 18 °C, stirred for a further 0.75 h at this temperature then transferred, via cannula, to a chilled (0 °C) and magnetically stirred solution of the alcohol 14 (15 mg, 63 µmol), triethylamine (18.4 µL, 0.13 mmol) and DMAP (2 mg) in CH2Cl2 (3 mL). The cold bath was removed, the reaction mixture stirred at room temperature for 16 h then the solution partitioned between CH2Cl2 (10 mL) and NaHCO3 (10 mL of a saturated aqueous solution) and the phases separated. The aqueous layer was extracted with CH2Cl2 (3 10 mL) and the combined organic fractions were washed with brine (1 10 mL) before being dried, filtered and concentrated under reduced pressure to give a pale-yellow oil. Subjection of the crude material to flash chromatography (silica, 1:4 v/v EtOAc/hexane elution) provided, after concentration of the appropriate fractions (Rf 0.4), the title compound (19 mg, 65%) as a clear, colorless oil. 1H NMR (300 MHz, CDCl3): δ 7.55–7.28 (complex m, 10H), 5.17 (m, 1H), 4.49 (s, 2H), 3.54 (s, 3H), 3.47–3.41 (complex m, 2H), 3.33 (d, J 5.5 Hz, 1H), 3.31 (s, 3H), 2.05–1.90 (complex m, 1H), 1.23 (d, J 6.3 Hz, 3H), 0.87 (d, J 7.1 Hz, 3H).
(2R,3R,4S)-3-Methoxy-4-methyl-5-(phenylmethoxy)pentan-2-yl (2S)-2-methoxy-2-phenyl-2-trifluoromethylacetate [(S)-Mosher ester of compound 14]: Oxalyl chloride (11.5 µL, 0.13 mmol) then DMF (1 drop) were added to a magnetically stirred solution of (S)-(–)-MTPA (31 mg, 0.13 mmol) in CH2Cl2 (0.5 mL) maintained at 0 °C (ice bath) under a nitrogen atmosphere. The reaction mixture was allowed to warm to room temperature, stirred for a further 0.75 h then transferred, via cannula, to a chilled (0 °C) and magnetically stirred solution of the alcohol 14 (15 mg, 63 µmol), triethylamine (18.4 µL, 0.13 mmol) and DMAP (2 mg) in CH2Cl2 (3 mL). The cold bath was removed, the reaction mixture stirred at room temperature for 16 h then partitioned between CH2Cl2 (10 mL) and NaHCO3 (10 mL of a saturated aqueous solution) and the phases separated. The aqueous layer was extracted with CH2Cl2 (3 10 mL) and the combined organic fractions were washed with brine (1 10 mL) before being dried, filtered and concentrated under reduced pressure to give a pale-yellow oil. Subjection of the crude material to flash chromatography (silica, 1:4 v/v EtOAc/hexane elution) provided, after concentration of the appropriate fractions (Rf 0.4), the title compound (19 mg, 65%) as a clear, colorless oil. 1H NMR (300 MHz, CDCl3): δ 7.58–7.28 (complex m, 10H), 5.20 (m, 1H), 4.46 (s, 2H), 3.60 (s, 3H), 3.40–3.34 (complex m, 2H), 3.26 (dd, J 9.0 and 5.4 Hz, 1H), 3.05 (s, 3H), 1.94–1.90 (complex m, 1H), 1.29 (d, J 6.4 Hz, 3H), 0.84 (d, J 6.9 Hz, 3H).
(2R,3R,4S)-2-(tert-Butyldimethylsiloxy)-3-methoxy-4-methyl-5-(phenylmethoxy)-pentane (15): A magnetically stirred solution of alcohol 14 (1.00 g, 4.2 mmol) and imidazole (0.43 g, 6.3 mmol) in DMF (30 mL) maintained under nitrogen was treated with tert-butyldimethylsilyl chloride (TBDMSCl) (0.76 g, 5.04 mmol) and the resulting mixture was heated at 60 °C (oil bath) for 3 h. The reaction mixture was then cooled, poured onto crushed ice (25 g) and extracted with Et2O (3 40 mL). The combined organic extracts were dried, filtered and then concentrated under reduced pressure. The oily residue thus obtained was subjected to flash chromatography (silica, 1:4 v/v EtOAc/hexane elution) and concentration of the appropriate fractions (Rf 0.7) then afforded the title compound 15 (1.20 g, 80%) as a clear, colorless oil, [α]D +10.4 (c 1.5); 1H NMR (300 MHz, CDCl3): δ 7.36–7.28 (complex m, 5H), 4.51 (s, 2H), 3.85 (m, 1H), 3.50 (m, 1H), 3.44 (s, 3H), 3.30 (dd, J 9.0 and 6.2 Hz, 1H) 3.10 (dd, J 7.1 and 3.4 Hz, 1H), 1.99 (m, 1H), 1.10 (d, J 6.4 Hz, 3H), 0.90 (s, 9H), 0.86 (d, J 7.0 Hz, 3H), 0.07 (s, 6H); 13C NMR (75.5 MHz, CDCl3): δ 138.6 (C), 128.3 (CH), 127.7 (CH), 127.5 (CH), 85.5 (CH), 73.3 (CH2), 73.0 (CH2), 70.5 (CH), 61.0 (CH3), 35.0 (CH), 25.9 (CH3), 20.2 (CH3), 18.1 (C), 10.9 (CH3), –4.7 (CH3) (one signal obscured or overlapping); IR (KBr): ṽmax 2930, 1455, 1255, 1099, 834, 776 cm–1; MS (70 eV): m/z (%): 295 (6) [M–C4H9•]+, 203 (12), 187 (17), 173 (30), 159 (45), 91 (100), 73 (40); HRMS (EI): m/z calcd for C16H27O3Si [M–C4H9•]+: 295.1730; found: 295.1730.
(2S,3R,4R)-4-(tert-Butyldimethylsiloxy)-3-methoxy-2-methylpentan-1-ol (16): A mixture of compound 15 (1.0 g, 2.84 mmol), palladium hydroxide on carbon (Pearlman’s catalyst) (0.15 g) and THF (50 mL) was stirred vigorously under an atmosphere of hydrogen (760 mmHg) for 3 h. The reaction mixture was then filtered through a 2 cm deep pad of TLC-grade silica and the filtrate concentrated under reduced pressure. The ensuing oily residue was subjected to flash chromatography (silica, 3:7 v/v EtOAc/hexane elution) and concentration of the appropriate fractions (Rf 0.5) then afforded the title alcohol 16 (0.7 g, 97%) as a clear, colorless oil, [α]D –3.6 (c 1.5); 1H NMR (300 MHz, CDCl3): δ 4.04 (m, 1H), 3.60–3.45 (complex m, 2H), 3.43 (s, 3H), 2.90 (dd, J 7.2 and 4.9 Hz, 1H), 1.90–1.84 (complex m, 1H), 1.20 (d, J 6.3 Hz, 3H), 0.93 (d, J 6.8 Hz, 3H), 0.91 (s, 9H), 0.12 (s, 6H) (signal due to OH group proton not observed); 13C NMR (75.5 MHz, CDCl3): δ 88.1 (CH), 69.2 (CH), 66.4 (CH2), 59.9 (CH3), 36.9 (CH), 25.8 (CH3), 18.4 (CH3), 18.0 (C), 13.2 (CH3), –4.8 (CH3), –4.9 (CH3); IR (KBr): ṽmax 3444, 2961, 2888, 2861, 1463, 1256, 1094, 835, 809, 775, 734 cm–1; MS (70 eV): m/z (%): 263 (1) [M+H]+, 215 (7), 173 (67), 159 (84), 89 (55), 73 (100); HRMS (EI): m/z calcd for C13H31O3Si [M+H]+: 263.2043; found: 263.2047.
(2S,3R,4R)-4-(tert-Butyldimethylsiloxy)-3-methoxy-2-methylpentanal (17): A solution of triethylamine (1.72 mL, 12.4 mmol) and alcohol 16 (0.5 g, 1.9 mmol) in CH2Cl2 (5 mL) was added, dropwise, to a magnetically stirred solution of the sulfur trioxide/pyridine complex (1.2 g, 7.6 mmol) in DMSO/CH2Cl2 (15.5 mL of a 3:2 v/v mixture) maintained at 0 °C (ice bath) under a nitrogen atmosphere. The reaction mixture was allowed to warm to room temperature and stirred for a further hour before being quenched with water (25 mL) and extracted with hexane (3 25 mL). The combined organic extracts were washed with brine (1 15 mL), then dried, filtered and concentrated under reduced pressure. The oily residue thus obtained was subjected to flash chromatography (silica, 1:9 v/v EtOAc/hexane elution) and concentration of the appropriate fractions (Rf 0.4) afforded the title aldehyde 17 (0.5 g, 94%) as a clear, colorless, but unstable oil. Since this aldehyde is highly prone to epimerization it was immediately subjected to the next step of the reaction sequence.
(3R,4S,5R,6R)-6-(tert-Butyldimethylsiloxy)-2,4-dimethyl-5-methoxy-1-penten-3-ol (18): Neat 2-bromopropene (6.23 mL, 70.1 mmol) was added, dropwise, to a magnetically stirred solution of tert-butyllithium (83.3 mL of a 1.7 M solution in pentane, 141.7 mmol, ex ALDRICH) in Et2O (180 mL) maintained under a nitrogen atmosphere at –78 °C. The resulting mixture was stirred at this temperature for 0.75 h and then treated with a solution of copper(I) bromide-dimethyl sulfide complex (7.3 g, 35.6 mmol) in DMSO (45 mL). The resulting mixture was stirred at –78 °C for a further 0.5 h and then treated with a solution of aldehyde 17 (1.35 g, 5.18 mmol) in Et2O (20 mL). Stirring was continued at this temperature for 0.5 h then the reaction mixture was quenched with NH4Cl (50 mL of a saturated aqueous solution). The slurry obtained in this manner was warmed to room temperature and extracted with Et2O (3 50 mL). The combined organic extracts were then dried, filtered and concentrated under reduced pressure. The ensuing residue was subjected to flash chromatography (silica, 1.5:8.5 v/v EtOAc/hexane elution) and concentration of the appropriate fractions (Rf 0.4) afforded the title compound 18 (1.10 g, 70%) as a clear, colorless oil, [α]D +3.2 (c 1.2); 1H NMR (300 MHz, CDCl3): δ 5.10 (s, 1H), 4.92 (s, 1H), 4.24 (s, 1H), 3.98 (m, 1H), 3.50 (s, 3H), 3.10 (dd, J 6.4 and 4.2 Hz, 1H), 1.84 (m, 1H), 1.68 (s, 3H), 1.14 (d, J 6.5 Hz, 3H), 0.90 (s, 9H), 0.84 (d, J 7.1 Hz, 3H), 0.10 (s, 6H) (signal due to OH group proton not observed); 13C NMR (75.5 MHz, CDCl3): δ 145.0 (C), 111.2 (CH2), 89.0 (CH), 77.2 (CH), 70.1 (CH), 60.3 (CH3), 36.1 (CH3), 25.9 (CH3), 19.5 (CH), 19.3 (CH3), 18.1 (C), 7.3 (CH3), –4.6 (CH3), –4.7 (CH3); IR (KBr): ṽmax 3447, 2931, 1462, 1256, 1093, 1068, 988, 900, 835, 776 cm–1; MS (70 eV): m/z (%): 245 (4) [M–C4H9•]+, 227 (4), 159 (100), 89 (49), 73 (100); HRMS (EI): m/z calcd for C12H25O3Si [M–C4H9•]+: 245.1573; found: 245.1570.
(2'R,3'S,4'S,5'R)-6'-(tert-Butyldimethylsiloxy)-2',4'-dimethyl-5'-methoxy-1'-penten-3'-yl propionate (4): A magnetically stirred solution of allylic alcohol 18 (150 mg, 0.5 mmol) in pyridine (4 mL) was treated with propionic anhydride (0.16 mL, 1.2 mmol) and a catalytic amount of DMAP (2 mg, 0.02 mmol). The resulting mixture was stirred at 18 °C for 16 h then poured onto crushed ice (ca. 10 g) and extracted with Et2O (3 25 mL). The combined organic extracts were then washed with HCl (1 × 10 mL of a 1 M aqueous solution) and brine (1 × 10 mL) before being dried, filtered and concentrated under reduced pressure. Subjection of this material to flash chromatography (silica, 1:4 v/v EtOAc/hexane elution) gave, after concentration of the appropriate fractions (Rf 0.6), the title ester 4 (160 mg, 90%) as a clear, colorless oil, [α]D –22 (c 1.6); 1H NMR (300 MHz, CDCl3): δ 5.25 (d, J 8.0 Hz, 1H), 5.02–5.00 (complex m, 2H), 3.91 (m, 1H), 3.43 (s, 3H), 2.92 (dd, J 6.5 and 2.8 Hz, 1H), 2.34 (q, J 7.5 Hz, 2H), 2.06–1.99 (complex m, 1H), 1.70 (s, 3H), 1.14 (t, J 7.6 Hz, 3H), 1.10 (d, J 6.4 Hz, 3H), 0.90 (s, 9H), 0.89 (d, J 8.8 Hz, 3H), 0.08 (s, 6H); 13C NMR (75.5 MHz, CDCl3): δ 173.5 (C), 142.0 (C), 115.5 (CH2), 85.0 (CH), 79.9 (CH), 70.2 (CH), 60.5 (CH3), 35.5 (CH3), 27.9 (CH2), 26.0 (CH3), 20.1 (CH), 18.1 (C), 18.0 (CH3), 9.6 (CH3), 9.2 (CH3), −4.6 (CH3) (two signals overlapping); IR (KBr): ṽmax 2931, 1741, 1463, 1256, 1184, 1095, 1006, 903, 835, 776 cm–1; MS (70 eV): m/z (%): 301 (4) [M–C4H9•]+, 227 (18), 199 (37), 159 (100), 125 (75), 89 (60), 73 (92); HRMS (EI): m/z calcd for C15H29O4Si [M–C4H9•]+: 301.1835; found: 301.1839.
(2S,6S,7R,8R,4E)-8-(tert-Butyldimethylsiloxy)-7-methoxy-2,4,6-trimethyl-4-nonenoic acid (19): A magnetically stirred solution of diisopropylamine (27 µL, 0.2 mmol) in THF (1.5 mL) was cooled to −10 °C then treated with n-butyllithium (113 µL of a 1.6 M solution in hexane, 0.18 mmol, ex ALDRICH). The resulting mixture was stirred at −10 °C for 0.25 h and then warmed to 18 °C and stirred at this temperature for a further 0.25 h. After this time the reaction mixture was cooled to –40 °C and THF (0.3 mL) then dry HMPA (0.15 mL) were added. The reaction mixture was then cooled to −78 °C and a solution of propionate ester 4 (50 mg, 0.14 mmol) was added dropwise over a period of 0.16 h. After 0.25 h TBDMSCl (0.03 g, 0.19 mmol) in THF (0.1 mL) was added rapidly with vigorous stirring. The resulting mixture was stirred at –78 °C for 0.25 h and then allowed to warm to 18 °C over a 0.75 h period. After heating to 60 °C for 6 h, the reaction mixture was diluted with HCl (5 mL of a 1 M aqueous solution) and extracted with Et2O (3 10 mL). The combined organic phases were washed with brine (1 10 mL) then dried, filtered and concentrated under reduced pressure. Subjection of this material to flash chromatography (silica, 1.5:8.5 v/v EtOAc/hexane elution) gave, after concentration of the appropriate fractions (Rf 0.2), the title acid 19 (38 mg, 75%) as a clear, colorless oil, [α]D +5.82 (c 2.15); 1H NMR (300 MHz, CDCl3): δ 5.20 (d, J 9.3 Hz, 1H), 3.83 (p, J 6.7 Hz, 1H), 3.43 (s, 3H), 2.80 (t, J 5.4 Hz, 1H), 2.65–2.53 (complex m, 2H), 2.38 (m, 1H), 2.05 (m, 1H), 1.61 (s, 3H), 1.13 (d, J 6.7 Hz, 3H), 1.11 (d, J 6.7 Hz, 3H), 1.00 (d, J 6.7 Hz, 3H), 0.90 (s, 9H), 0.06 (s, 6H) (signal due to CO2H group proton not observed); 13C NMR (75.5 MHz, CDCl3): δ 182.4 (C), 131.8 (CH), 130.1 (C), 89.7 (CH), 70.1 (CH), 60.8 (CH3), 43.7 (CH2), 37.9 (CH), 33.8 (CH), 25.9 (CH3), 20.1 (CH3), 18.1 (C), 16.4 (CH3), 15.8 (CH3), 15.4 (CH3), –4.5(6) (CH3), −4.6(2) (CH3); IR (KBr): ṽmax 2958, 2931, 1709, 1254, 1093, 834, 776 cm–1; MS (70 eV): m/z (%): 358 (1) [M]+•, 301 (2) [M–C4H9•]+, 225 (8), 203 (90), 159 (57), 133 (59), 89 (69), 73 (100); HRMS (EI): m/z calcd for C15H29O4Si [M–C4H9•]+: 301.1835; found: 301.1836.
(2S,6S,7R,8R,5E)-8-(tert-Butyldimethylsiloxy)-7-methoxy-2,4,6-trimethyl-4-nonen-1-ol (20): LiAlH4 (0.28 mL of a 1 M solution in THF, 0.28 mmol, ex ALDRICH) was added, dropwise, to a magnetically stirred solution of the acid 19 (100 mg, 0.28 mmol) in Et2O (4 mL) maintained under a nitrogen atmosphere at 0 °C. The resulting mixture was allowed to warm to room temperature and stirred for a further 1 h then cooled and treated sequentially with water (2 mL), NaOH [2 mL of a 10% (w/v) aqueous solution] and water (5 mL). The resulting precipitate was filtered off and the solids thus retained were washed successively with Et2O (1 50 mL) and hot CHCl3 (1 25 mL). The combined filtrates were then dried, filtered and concentrated under reduced pressure to give a light-yellow oil. Subjection of this material to flash chromatography (silica, 1:4 v/v EtOAc/hexane elution) gave, after concentration of the appropriate fractions (Rf 0.4), the title alcohol 20 (63 mg, 66%) as a clear, colorless oil, [α]D +6.4 (c 1.6); 1H NMR (300 MHz, CDCl3): δ 5.20 (d, J 9.2 Hz, 1H), 3.83 (m, 1H), 3.53–3.47 (complex m, 2H), 3.44 (s, 3H), 2.80 (t, J 5.7 Hz, 1H), 2.58–2.55 (complex m, 1H), 2.10–2.03 (complex m, 1H), 1.87–1.80 (complex m, 2H), 1.62 (s, 3H), 1.52 (br s, 1H), 1.12 (d, J 6.2 Hz, 3H), 0.92 (d, J 6.7 Hz, 3H), 0.87 (d, J 6.9 Hz, 3H), 0.90 (s, 9H), 0.06 (s, 6H); 13C NMR (75.5 MHz, CDCl3): δ 132.0 (C), 130.8 (CH), 89.7 (CH), 70.1 (CH), 68.6 (CH2), 60.9 (CH3), 44.4 (CH2), 33.7(2) (CH), 33.6(9) (CH), 26.0 (CH3), 20.1 (CH3), 18.1 (C), 16.9 (CH3), 16.1 (CH3), 15.4 (CH3), −4.5(6) (CH3), –4.6(0) (CH3); IR (KBr): ṽmax 3337, 2957, 2930, 1461, 1384, 1255, 1093, 834, 775 cm−1; MS (70 eV): m/z (%): 344 (1) [M]+•, 312 (1), 287 (2) [M–C4H9•]+, 255 (12), 203 (82), 159 (58), 133 (56), 89 (75), 73 (100); HRMS (EI): m/z calcd for C19H40O3Si [M]+•: 344.2747; found: 344.2750.
(2S,6S,7R,8R,5E)-8-(tert-Butyldimethylsiloxy)-7-methoxy-2,4,6-trimethyl-4-nonenal (21): The Dess–Martin periodinane (90 mg, 0.22 mmol) was added to a magnetically stirred solution of alcohol 20 (51 mg, 0.15 mmol) in CH2Cl2 (1.5 mL) maintained at 18 °C under a nitrogen atmosphere. Stirring was continued for 0.75 h then the reaction mixture was diluted with Et2O (2 mL), NaHCO3 (2 mL of a saturated aqueous solution) and Na2S2O3 (2 mL of a 1 M aqueous solution) and stirring was continued until two clear layers were observed. The separated aqueous layer was extracted with Et2O (2 × 15 mL) and the combined organic fractions were then dried, filtered and concentrated under reduced pressure to give a light-yellow oil. Subjection of this material to flash chromatography (silica, 1:4 v/v EtOAc/hexane elution) gave, after concentration of the appropriate fractions (Rf 0.7), the title compound 21 (31 mg, 62%) as a clear, colorless oil. 1H NMR (300 MHz, CDCl3): δ 9.61 (d, J 2.3 Hz, 1H), 5.22 (d, J 9.3 Hz, 1H), 3.83 (m, 1H), 3.43 (s, 3H), 2.80 (t, J 5.5 Hz, 1H), 2.59–2.38 (complex m, 3H), 2.04–1.94 (complex m, 1H), 1.61 (s, 3H), 1.11 (d, J 6.7 Hz, 3H), 1.04 (d, J 7.0 Hz, 3H), 0.91 (d, J 6.6 Hz, 3H), 0.90 (s, 9H), 0.06 (s, 6H).
This compound is unstable and was not, therefore, subjected to full characterization but, rather, immediately subjected to the next step of the reaction sequence.
Methyl 2-{(2R,5S,6S)-6-[(E)-4-(diphenylphosphoryl)but-2-en-2-yl]-5-methyltetrahydro-2H-pyran-2-yl}acetate (3): The title compound was prepared from 4-penten-1-ol (22, ex ALDRICH) using the reaction sequence shown in Scheme 4 and according to a previously published procedure.13d The material obtained by this means was identical, an all respects, with an authentic sample.
Methyl (2S,3S,6R)-{[1E,3E,7E,11R,10R,9R,5S]-11-tert-butyldimethylsiloxy-10-methoxy-1,5,7,9-tetramethyldodeca-1,3,7-trienyl}-3-methyloxan-6-yl}ethanoate (33) and methyl (2S,3S,6R)-{[1E,3E,7E,11R,10R,9R,5R]-11-tert-butyldimethylsiloxy-10-methoxy-1,5,7,9-tetramethyldodeca-1,3,7-trienyl]-3-methyl-oxan-6-yl}ethanoate: A solution of phosphine oxide 3 (62 mg, 0.15 mmol) in THF (2 mL) was added, via syringe, to a magnetically stirred suspension of NaH (60 mg of a 35% w/w dispersion in mineral oil, 1.5 mmol) in anhydrous THF (10 mL) maintained under a nitrogen atmosphere. The resulting mixture was stirred at room temperature for 0.5 h and then treated with compound 21 (50 mg, 0.15 mmol). The reaction mixture was then heated to 55 °C and stirring was continued for 2 h. The cooled reaction mixture was then poured onto crushed ice and the resulting melt was acidified with concentrated HCl to pH~2–3. The mixture thus obtained was extracted with Et2O (3 20 mL) and the combined organic extracts were then dried, filtered and concentrated under reduced pressure to give a clear, colorless oil. This material was treated with diazomethane (8 mL of a 0.35 M solution in Et2O, 2.8 mmol) at 0 °C and the resulting mixture was allowed to warm to room temperature and left stirring for 0.5 h. The light-yellow solution thus obtained was poured into water (10 mL) and extracted with Et2O (3 10 mL). The combined organic extracts were then dried, filtered and concentrated under reduced pressure to give a pale-yellow oil. This material was subjected to semi-preparative HPLC (2,3-propanediol column, 1:99 v/v MTBE/hexane elution, flow rate 2 mL/min) and two fractions A and B were obtained.
Concentration of fraction A (Rt 30 min) afforded the title compound 33 (30 mg, 38%) as a clear, colorless oil, [α]D +0.35 (c 2.3); 1H NMR (300 MHz, CDCl3): δ 6.24–6.13 (complex m, 1H), 5.92–5.87 (complex m, 1H), 5.55 (m, 1H), 5.13 (m, 1H), 3.86–3.72 (complex m, 2H), 3.66 (s, 3H), 3.44 (s, 3H), 3.32 (d, J 9.7 Hz, 1H), 2.80–2.75 (complex m, 1H), 2.65–2.48 (complex m, 2H), 2.38 (m, 2H), 2.07–1.95 (complex m, 1H), 1.93–1.76 (complex m, 2H), 1.70 (s, 3H), 1.58 (s, 3H), 1.55–1.20 (complex m, 4H), 1.12 (d, J 6.4 Hz, 3H), 0.95 (d, J 6.7 Hz, 3H), 0.93 (partially obscured m, 3H), 0.90 (s, 9H), 0.68 (d, J 6.5 Hz, 3H), 0.06 (s, 6H); 13C NMR (75.5 MHz, CDCl3): δ 171.9 (C), 140.6 (CH), 134.1 (C), 131.5 (C), 130.8 (CH), 128.5 (CH), 123.8 (CH), 90.5 (CH), 89.8 (CH), 73.8 (CH), 70.1 (CH), 60.8 (CH3), 51.5 (CH3), 47.5 (CH2), 41.3 (CH2), 35.1 (CH), 33.7 (CH), 32.3 (CH2), 32.1 (CH), 31.6 (CH2), 26.0 (CH3), 20.2 (CH3), 20.1 (CH3), 18.1 (C), 17.7 (CH3), 16.2, (CH3), 15.5 (CH3), 12.0 (CH3), –4.6(0) (CH3) (one signal obscured or overlapping); IR (KBr): ṽmax 2954, 1743, 1455, 1254, 1197, 1067, 834, 775 cm−1; MS (70 eV): m/z (%): 550 (22) [M]+•, 493 (2), 449 (7), 347 (6), 265 (98), 203 (100), 159 (40), 133 (32), 95 (48), 73 (70); HRMS (EI): m/z calcd for C32H58O5Si [M]+•: 550.4054; found: 550.4060.
Concentration of fraction B (Rt 26 min) afforded methyl (2S,3S,6R)-{[1E,3E,7E,11R,10R,9R,5R)-11-tert-butyldimethylsiloxy-10-methoxy-1,5,7,9-tetramethyldodeca-1,3,7-trienyl]-3-methyloxan-6-yl}ethanoate (20 mg, 25%) as a clear, colorless oil. 1H NMR (300 MHz, CDCl3): δ 6.24–6.10 (complex m, 1H), 5.90 (d, J 11.0 Hz, 1H), 5.60 (dd, J 15.1 and 7.3 Hz, 1H), 5.13 (d, J 9.5 Hz, 1H), 3.84–3.74 (complex m, 2H), 3.66 (s, 3H), 3.43 (s, 3H), 3.31 (d, J 9.8 Hz, 1H), 2.80–2.74 (complex m, 1H), 2.64–2.51 (complex m, 2H), 2.44–2.36 (complex m, 2H), 2.07–2.00 (complex m, 1H), 1.95–1.81 (complex m, 2H), 1.70 (s, 3H), 1.58 (br s, 3H), 1.57–1.20 (complex m, 4H), 1.12 (d, J 6.4 Hz, 3H), 0.93 (d, J 3.9 Hz, 3H), 0.91 (d, J 4.1 Hz, 3H), 0.90 (s, 9H), 0.69 (d, J 6.6 Hz, 3H), 0.06 (s, 6H); 13C NMR (75.5 MHz, CDCl3): δ 171.9 (C), 140.7 (CH), 134.2 (C), 131.5 (C), 130.9 (CH), 128.4 (CH), 123.7 (CH), 90.8 (CH), 89.7 (CH), 73.8 (CH), 70.1 (CH), 60.9 (CH3), 51.5 (CH3), 47.5 (CH2), 41.3 (CH2), 34.8 (CH), 33.7 (CH), 32.3 (CH2), 32.2 (CH), 31.6 (CH2), 26.0 (CH3), 20.2 (CH3), 19.5 (CH3), 18.1 (C), 17.7 (CH3), 16.2, (CH3), 15.5 (CH3), 12.3 (CH3), –4.60 (CH3) (two overlapping peaks); IR (KBr): ṽmax 2954, 1743, 1455, 1254, 1197, 1067, 834, 775 cm−1; UV (methanol): λmax (ε) 239 (21,080) nm; MS (70 eV): m/z (%): 550 (40) [M]+•, 493 (2), 449 (11), 347 (10), 265 (90), 203 (100), 159 (50), 133 (42), 95 (56), 73 (74), 59 (22); HRMS (EI): m/z calcd for C32H58O5Si [M]+•: 550.4054; found: 550.4049.
Methyl (2S,3S,6R)-{[1E,3E,7E,11R,10R,9R,5S)-11-hydroxy-10-methoxy-1,5,7,9-tetramethyldodeca-1,3,7-trienyl]-3-methyloxan-6-yl}ethanoate (34): Tetra-n-butylammonium fluoride (61 µL of a 1 M solution in THF, 61 µmol) was added, dropwise, to a magnetically stirred solution of the compound 33 (23 mg, 0.04 mmol) in THF (2 mL) maintained at 0 °C under a nitrogen atmosphere. The resulting mixture was allowed to warm to room temperature and stirred for a further 6 h then water (5 mL) was added and the phases separated. The aqueous layer was extracted with CH2Cl2 (3 10 mL) and the combined organic phases were washed with brine (1 5 mL) then dried, filtered and concentrated under reduced pressure to give a light-yellow oil. Subjection of this material to flash chromatography (silica, 3:7 v/v EtOAc/hexane elution) gave, after concentration of the appropriate fractions (Rf 0.4), the title compound 34 (12 mg, 68%) as a clear, colorless oil. 1H NMR (300 MHz, CDCl3): δ 6.23–6.13 (complex m, 1H), 5.92–5.88 (complex m, 1H), 5.60–5.50 (complex m, 1H), 5.00–4.95 (complex m, 1H), 3.80–3.70 (complex m, 2H), 3.66 (s, 3H), 3.50 (s, 3H), 3.32 (d, J 9.7 Hz, 1H), 2.72–2.56 (complex m, 3H), 2.43–2.36 (complex m, 2H), 2.10–1.80 (complex m, 3H), 1.70 (s, 3H), 1.61 (s, 3H), 1.57–1.23 (complex m, 4H), 1.19 (d, J 6.5 Hz, 3H), 0.95 (d, J 6.5 Hz, 3H), 0.69 (m, 6H) (signal due to OH group proton not observed).
Methyl (2S,3S,6R)-{[1E,3E,11R,10R,9R,8R,7R,5S)-7,8-epoxy-11-hydroxy-10-methoxy-1,5,7,9-tetramethyldodeca-1,3-dienyl]-3-methyloxan-6-yl}ethanoate (35) and methyl (2S,3S,6R)-{[1E,3E,11R,10R,9R,8S,7S,5S)-7,8-epoxy-11-hydroxy-10-methoxy-1,5,7,9-tetramethyldodeca-1,3-dienyl]-3-methyloxan-6-yl}ethanoate: A magnetically stirred solution of compound 34 (20 mg, 0.047 mmol) in anhydrous CH2Cl2 (0.4 mL) maintained at –8 °C (cryobath) under a nitrogen atmosphere was treated with vanadyl bis(acetylacetonate) (0.12 mg, 0.5 µmol) then a solution of tert-butyl hydroperoxide (TBHP) (28 µL of a 5.0 M solution in decane, 0.14 mmol) in anhydrous CH2Cl2 (0.4 mL) added via a syringe pump over 48 h. After the complete addition of the stoichiometric oxidant the colorless solution was allowed to stir for a further 24 h at –8 °C. After this time the reaction mixture was diluted with Et2O (5 mL) and water (5 mL) and the layers shaken well and then separated. The aqueous phase was then extracted with CH2Cl2 (3 5 mL) and the combined organic extracts were washed with brine (1 5 mL) then dried before being filtered and concentrated under reduced pressure. The resulting yellow oil was subjected to semi-preparative HPLC (2,3-propanediol column, 1.5:8.5 v/v MTBE/hexane elution, flow rate 1 mL/min) and two fractions, A and B, were obtained.
Concentration of fraction A (Rt 35 min) afforded compound 35 (13 mg, 63%) as a clear, colorless oil, [α]D +1.3 (c 0.2) {lit.7 [α]D +0.9 (c 0.7, CHCl3)}; 1H NMR (300 MHz, CDCl3): δ 6.23 (dd, J 15.3 and 10.6 Hz, 1H), 5.90 (d, J 10.6 Hz, 1H), 5.43 (dd, J 15.3 and 8.8 Hz, 1H), 3.86–3.77 (complex m, 1H), 3.76–3.75 (complex m, 1H), 3.66 (s, 3H), 3.54 (s, 3H), 3.32 (d, J 9.8 Hz, 1H), 2.97 (t, J 5.4 Hz, 1H), 2.63–2.54 (complex m, 2H), 2.43–2.36 (complex m, 2H), 1.92 (dd, J 13.7 and 4.7 Hz, 1H), 1.86–1.81 (complex m, 1H), 1.70 (s, 3H), 1.67–1.56 (complex m, 3H), 1.54–1.30 (complex m, 3H), 1.28 (s, 3H), 1.18 (d, J 6.4 Hz, 3H), 1.04 (d, J 6.7 Hz, 3H), 0.87 (d, J 6.7 Hz, 3H), 0.66 (d, J 6.6 Hz, 3H) (signal due to OH group proton not observed); 13C NMR (75.5 MHz, CDCl3): δ 171.8 (C), 139.2 (CH), 135.2 (C), 128.1 (CH), 125.2 (CH), 90.6 (CH), 87.6 (CH), 73.8 (CH), 68.2 (CH), 66.0 (CH), 61.4 (CH3), 61.3 (C), 51.6 (CH3), 46.9 (CH2), 41.3 (CH2), 35.3 (CH), 35.2 (CH), 32.2 (CH2), 32.0 (CH), 31.6 (CH2), 22.1 (CH3), 19.0 (CH3), 17.6 (CH3), 16.5 (CH3), 11.9 (CH3), 11.9 (CH3); IR (KBr): ṽmax 3454, 2917, 2849, 1738, 1455, 1068, 756 cm–1; UV (methanol): λmax (ε) 246 (21,000) nm; MS (70 eV): m/z (%): 452 (10) [M]+•, 434 (4), 351 (4), 305 (4), 278 (12), 265 (12), 237 (5), 197 (14), 169 (58), 157 (50), 129 (100), 95 (84), 69 (66); HRMS (EI): m/z calcd for C26H44O6 [M]+•: 452.3138; found: 452.3137.
Concentration of fraction B (Rt 36 min) afforded trace amounts (<0.5 mg) of a light-yellow oil that is tentatively identified as methyl (2S,3S,6R)-{[1E,3E,11R,10R,9R,8S,7S,5S)-7,8-epoxy-11-hydroxy-10-methoxy-1,5,7,9-tetramethyldodeca-1,3-dienyl]-3-methyloxan-6-yl}ethanoate, the diastereoisomer of herboxidiene methyl ester.
ACKNOWLEDGEMENTS
We thank the Institute of Advanced Studies (ANU) and Dunlena Pty Ltd for financial support. RP is the grateful to the Australian Research Council for the provision of an APA(I) Scholarship.
References
1. B. G. Isaac, S. W. Ayer, R. C. Elliott, and R. J. Stonard, J. Org. Chem., 1992, 57, 7220. CrossRef
2. A. J. F. Edmunds, W. Trueb, W. Oppolzer, and P. Cowley, Tetrahedron, 1997, 53, 2785. CrossRef
3. Y. Sakai, T. Yoshida, K. Ochiai, Y. Uosaki, Y. Saitoh, F. Tanaka, T. Akiyama, S. Akinaga, and T. Mizukami, J. Antibiot., 2002, 55, 855. CrossRef
4. Y. Koguchi, M. Nishio, J. Kotera, K. Omori, T. Ohnuki, and S. Komatsubara, J. Antibiot., 1997, 50, 970. CrossRef
5. Y. Sakai, T. Tsujita, T. Akiyama, T. Yoshida, T. Mizukami, S. Akinaga, S. Horinouchi, M. Yoshida, and T. Yoshida, J. Antibiot., 2002, 55, 863. CrossRef
6. M. Hasegawa, T. Miura, K. Kuzuya, A. Inoue, S. W. Ki, S. Horinouchi, T. Yoshida, T. Kunoh, K. Koseki, K. Mino, R. Sasaki, M. Yoshida, and T. Mizukami, ACS Chem. Biol., 2011, 6, 229. CrossRef
7. P. R. Blakemore, P. J. Kocienski, A. Morley, and K. Muir, J. Chem. Soc., Perkin Trans. 1, 1999, 955. CrossRef
8. Y. Zhang and J. S. Panek, Org. Lett., 2007, 9, 3141. CrossRef
9. T. J. Murray and C. J. Forsyth, Org. Lett., 2008, 10, 3429. CrossRef
10. A. K. Ghosh and J. Li, Org. Lett., 2011, 13, 66. CrossRef
11. M. Pellicena, K. Krämer, P. Romea, and F. Urpí, Org. Lett., 2011, 13, 5350. CrossRef
12. A. J. F. Edmunds, G. Arnold, L. Hagmann, R. Schaffner, and H. Furlenmeier, Bioorg. Med. Chem. Lett., 2000, 10, 1365. CrossRef
13. For our earlier publications in the area see: a) M. G. Banwell, C. T. Bui, G. W. Simpson, and K. G. Watson, Chem. Commun., 1996, 723; CrossRef b) M. G. Banwell, C. T. Bui, D. C. R. Hockless, and G. W. Simpson, J. Chem. Soc., Perkin Trans. 1, 1997, 1261; CrossRef c) M. G. Banwell, C. T. Bui, and G. W. Simpson, J. Chem. Soc., Perkin Trans. 1, 1998, 791; CrossRef d) M. G. Banwell, M. D. McLeod, R. Premraj, and G. W. Simpson, Aust. J. Chem., 2000, 53, 659; CrossRef e) M. G. Banwell, M. D. McLeod, R. Premraj, and G. W. Simpson, Pure Appl. Chem., 2000, 72, 1631. CrossRef
14. See: a) S. Pereira and M. Srebnik, Aldrichchimica Acta, 1993, 26, 17; b) C.-L. Chen, K. Namba, and Y. Kishi, Org. Lett., 2009, 11, 409 and references cited therein. CrossRef
15. M. G. Banwell, B. D. Bissett, C. T. Bui, H. T. T. Pham, and G. W. Simpson, Aust. J. Chem., 1998, 51, 9. CrossRef
16. A. K. Bhattacharya and G. Thyagarajan, Chem. Rev., 1981, 81, 415. CrossRef
17. For a useful commentary on the synthesis and utilization of this compound see: M. Qiu, D.-Y. Wang, X.-P. Hu, J.-D. Huang, S.-B. Yu, J. Deng, Z.-C. Duan, and Z. Zheng, Tetrahedron: Asymmetry, 2009, 20, 210. CrossRef
18. U. Widmer, Synthesis, 1987, 568. CrossRef
19. J. M. Williams, R. B. Jobson, N. Yasuda, G. Marchesini, U.-H. Dolling, and E. J. J. Grabowski, Tetrahedron Lett., 1995, 36, 5461.
20. S. Narasimhan and R. Balakumar, Aldrichimia Acta, 1998, 31, 19.
21. See: N. Bajwa and M. P. Jennings, J. Org. Chem., 2008, 73, 3638, and references cited therein. CrossRef
22. S. D. Burke, R. A. Ng, J. A. Morrison, and M. J. Alberti, J. Org. Chem., 1998, 63, 3160. CrossRef
23. T. R. Hoye, C. S. Jeffrey, and F. Shao, Nat. Protoc., 2007, 2, 2451. CrossRef
24. M. T. Reetz, Acc. Chem. Res., 1993, 26, 462. CrossRef
25. J. R. Parikh and W. v. E. Doering, J. Am. Chem. Soc., 1967, 89, 5505. CrossRef
26. S. R. Schow, J. D. Bloom, A. S. Thompson, K. N. Winzenberg, and A. B. Smith, J. Am. Chem. Soc., 1986, 108, 2662. CrossRef
27. The formation of the corresponding E-silyl ketene acetal (so as to establish the required S stereochemistry within the Ireland–Claisen rearrangement product) was not investigated.
28. When compound 20 was subjected to sequential reaction with dimethyldioxirane, mineral acid then tetra-n-butylammonium fluoride two stereochemically distinct tetrahydrofurans were obtained, neither of which was identical to that obtained by degradation of herboxidiene itself (Ref. 2). Such observations served to confirm that compounds 19–21 possess the illustrated stereochemistry at C12.
29. R. E. Ireland and L. Liu, J. Org. Chem., 1993, 58, 2899. CrossRef
30. I. Coldham, A. J. M. Burrell, H. D. S. Guerrand, and N. Oram, Org. Lett., 2011, 13, 1267, and references therein.. CrossRef
31. a) A. G. M. Barrett, D. Hamprecht, and M. Ohkubo, J. Org. Chem., 1997, 62, 9376; CrossRef b) E. Lee, C. H. Yoon, T. H. Lee, S. Y. Kim, T. J. Ha, Y-s. Sung, S.-H. Park, and S. Lee, J. Am. Chem. Soc., 1998, 120, 7469; CrossRef c) X. Wei and R. J. K. Taylor, Tetrahedron Lett., 1998, 39, 3815; CrossRef d) X. Wei and R. J. K. Taylor, J. Org. Chem., 2000, 65, 616. CrossRef
32. T. Katsuki and V. S. Martin, Org. React., 1996, 48, 1.
33. T. Suzuki, H. Saimoto, H. Tomioka, K. Oshima, and H. Nozaki, Tetrahedron Lett., 1982, 23, 3597. CrossRef
34. W. C. Still and C. Gennari, Tetrahedron Lett., 1983, 24, 4405. CrossRef
35. a) M. P. Edwards, S. V. Ley, S. G. Lister, B. D. Palmer, and D. J. Williams, J. Org. Chem., 1984, 49, 3503; CrossRef b) K. C. Nicolaou, D. P. Papahatjis, D. A. Claremon, R. L. Magolda, and R. E. Dolle, J. Org. Chem., 1985, 50, 1440. CrossRef
36. A. D. Buss and S. Warren, J. Chem. Soc., Perkin Trans. 1, 1985, 2307, and references cited therein. CrossRef
37. T. Itoh, K. Jitsukawa, K. Kaneda, and S. Teranishi, J. Am. Chem. Soc., 1979, 101, 159, and references cited therein. CrossRef
38. W. C. Still, M. Kahn, and A. Mitra, J. Org. Chem., 1978, 43, 2923. CrossRef
39. D. D. Perrin and W. L. F. Armarego, ‘Purification of Laboratory Chemicals’ 3rd ed., Pergamon, Oxford, 1988.
40. S. C. Watson and J. F. Eastham, J. Organomet. Chem., 1967, 9, 165. CrossRef