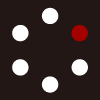
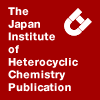
HETEROCYCLES
An International Journal for Reviews and Communications in Heterocyclic ChemistryWeb Edition ISSN: 1881-0942
Published online by The Japan Institute of Heterocyclic Chemistry
e-Journal
Full Text HTML
Received, 4th October, 2012, Accepted, 12th October, 2012, Published online, 22nd October, 2012.
DOI: 10.3987/COM-12-S(N)128
■ Formal Synthesis of (±)-Clausenamide by NHC-Catalyzed γ-Lactam Formation
Ming He, Michael Rommel, and Jeffrey W. Bode*
Laboratory of Organic Chemistry, Department of Chemistry and Applied Biosciences, ETH Zürich, Wolfgang-Pauli-Strasse 10, CH-8093 Zuerich, Switzerland
Abstract
(±)-Clausenamide is a biologically active γ-lactam isolated as a racemic mixture from Clausena lansium. Reported medicinal properties include hepatoprotective effects as well as neuroprotective and nootropic activity. In order to improve synthetic access to this molecule and its analogues, and to devise a route amenable to asymmetric catalysis, we have developed a new formal synthesis of (±)-clausenamide based on an N-heterocyclic carbene (NHC) catalyzed annulation of cinnamaldehyde and an unsaturated imine. The key γ-lactam forming annulation proceeds at room temperature in good yield and excellent diastereoselectivity. Preliminary efforts towards an enantioselective variant are also reported.Chinese folk medicine uses teas made from Clausena lansium to treat both acute and chronic hepatitis. Subsequent studies have attributed these hepatoprotective effects to the γ-lactam natural product (±)-clausenamide, which was isolated from this source in 1984 as about 0.04% of the dry leaf weight.1,2 Preliminary biological evaluations showed that clausenamide (1) showed a marked hepatoprotective effect against chemical toxins, such as carbon tetrachloride and thioacetamide. More recently, interest in clausenamide has focused on its potential neuroprotective and nootropic effects.3 Numerous studies have identified a protective effect of (–)-clausenamide on memory and neuronal DNA damage upon induced cerebral artery occlusion (i.e. stroke).4 Several other members of the clausenamide family, including neoclausenamide (2)5 and homoclausenamide (3)6 have been isolated and have begun to attract attention (Scheme 1).7
These promising biological activities have inspired several groups to develop synthetic routes to clausenamide with the aim of providing access to analogues by a concise, scalable approach. Unusual for a chiral, naturally occurring substance, clausenamide is isolated as a racemic mixture. Separation of the enantiomers and examination of their biological properties has revealed that only the (3R,4S,5S) enantiomer is neurologically active. Therefore, while racemic clausenamide can be obtained from natural sources, a synthetic, enantioselective route would be highly desirable. At least three reports of its synthesis or formal synthesis have already appeared,8 along with the synthesis of neoclausenamide and homoclausenamide.
In this report, we document the NHC-catalyzed synthesis of lactam 8 and its elaboration into (±)-clausenamide core 5, which has been previously converted into the natural product by a short synthetic sequence.5 Our studies provide an improved, straightforward route to racemic clausenamide as well as the framework for a catalytic, enantioselective synthesis through the use of chiral N-heterocyclic carbene catalysts.9
The most obvious synthetic disconnection of clausenamide is opening of the γ-lactam to reveal a γ-amino acid. The synthesis of this densely functionalized stereochemical array presents a considerable challenge and most researchers have instead elaborated preformed γ-lactams en route to clausenamide. Two syntheses, including the work of Hartwig and Born that serves as our inspiration have passed through lactam 5, which we believed to be accessible by our previously reported NHC-catalyzed annulations of imines and α,β-unsaturated aldehyde.10 Our retrosynthetic analysis of clausenamide therefore took advantage of our ability to form lactam 8 by NHC-catalyzed homoenolate addition and our conviction that this product could be converted to lactam 5 (Scheme 2).
As a starting point for our synthetic approach to clausenamide, we selected the annulation of cinnamaldehyde (9) and N-sulfonylimine 10 (Scheme 3). By performing this reaction at room temperature, we found that we could produce lactam 8 in good yield and on a gram scale as an 8:1 mixture of diasteroisomers. After the deprotection of the sulfonamide protecting group, the minor diastereomer was easily removed by column chromatography and the desired cis isomer 11 was obtained in excellent yield. This cis-lactam was methylated by deprotonation with sodium hydride followed by trapping with methyl iodide to give N-Me lactam 12 in 60% overall yield from annulation product 8.
To complete the formal synthesis of clausenamide, lactam 12 was subjected to ozonolysis followed by the addition of phenylmagnesium bromide to the unpurified aldehyde afforded, as a single diastereomer, secondary alcohols 13 in 63% yield. This material has been previously prepared by Hartwig and Born by an 8-step route and its conversion to (±)-clausenamide has been described. The preparation of 13 therefore constitutes a formal synthesis of this natural product.
Overall, our improved access to 13 occurs in a 5-step sequence from cinnamaldehyde and features a diastereoselective, NHC-catalyzed annulation to form the key lactam structure by a simple, scalable reaction. Both of the reaction components are derived from readily available cinnamaldehyde.
With the longer term goal of developing a catalytic, enantioselective approach to the biologically active (–)-clausenamide in mind, we have briefly evaluated the feasibility of conducting the NHC-catalyzed γ-lactam formation with a chiral catalyst. As we have previously documented,11 this γ-lactam forming annulation proceeds only with imidazolium-derived N-heterocyclic carbenes; triazolium-derived catalyst 14 that has proven to be outstanding for numerous highly enantioselective annulations are unfortunately unreactive in this process.12 When the annulations were attempted in CH2Cl2 and with Hünig’s base, which are the more usual conditions for the use of triazolium salts, the only observed product was small amounts of the hetero-Diels-Alder adduct 15 (Scheme 4). We therefore examined the use of (S,R)-aminoindanol-derived imidazolium precatalyst 16,13 but found that this catalyst was also non-productive in our initial studies.
We attributed the failure of the annulation to proceed with the imidazolium-precatalyst to inhibition by the highly electrophilic imine 10. To modulate the electrophilicity, we prepared the N-sulfonyl imine of para-methoxycinnamaldehyde (17), which was expected to be less electrophilic and therefore less inclined to inhibit the chiral N-heterocyclic carbene catalyst. With this substrate, we observed modest conversion to the desired γ-lactam 18 with 25% ee (Scheme 5). The use of the imine derived from α-methylcinnamaldehyde (19) offered modest improvements, with the product obtained in 53% ee. Although further improvement is clearly needed, these studies establish that enantioselective NHC-catalysis can be a viable approach to the asymmetric synthesis of clausenamide and analogues. Emerging results in this area using alternative substrates and catalyst may be suitable for the synthesis of clausenamide.14
In summary, we have documented a concise and facile synthesis of the natural product (±)-clausenamide (1) featuring an NHC-catalyzed annulation. The key C–C bond forming annulation to access the γ-lactam core proceeds under mild, operationally friendly reaction conditions using commercially available IMesCl as the catalyst. Varying the annulation partners or the aryl substituent introduced by nucleophilic addition will allow access to clausenamide-analogs with the same synthetic route. We have also briefly explored the use of chiral NHC precatalysts for affecting an enantioselective annulation. Although further studies and improved catalysts are needed, this work established an NHC-catalyzed annulation that can serve as an entry to the enantioselective synthesis of clausenamide.
EXPERIMENTAL
(E)-4-Methoxy-N-((E)-3-phenylallylidene)benzenesulfonamide (10) Cinnamaldehyde dimethyl acetal (1.0 equiv) and the arylsulfonamide (1.0 equiv) were mixed in a flask equipped with a Dean-Stark condenser. The neat mixture was heated to 180 °C for 30 min. The resulting melt was cooled to room temperature and the solid was crystallized from toluene to yield the product. mp 125–190 °C; 1H NMR (400 MHz, CDCl3) δ 8.74 (d, 1H, J = 9.5 Hz), 7.89 (d, 2H, J = 8.9 Hz), 7.54–7.40 (m, 6H), 7.00–6.93 (m, 3H,), 3.85 (s, 3H); 13C NMR (100 MHz, CDCl3) δ 170.5, 163.7, 153.8, 134.2, 131.7, 130.2, 129.7, 129.3, 128.7, 124.7, 114.5, 55.8; IR (thin film) v 3054, 2987, 2305, 1581, 1499, 1421, 1266, 1153, 1092, 896, 857, 807, 743, 705 cm-1; ESI-MS: 302.1 (M+H)+.
cis-1-(4-Methoxyphenylsulfonyl)-4-phenyl-5-(2-para-tolylethylidene)pyrrolidin-2-one (8). Into an oven dried flask was weighed cinnamaldehyde (0.90 g, 6.8 mmol, 1.0 equiv), imine 10 (2.05 g, 6.8 mmol, 1.0 equiv) and IMesCl (0.35 g, 1.0 mmol, 0.15 equiv). The flask was evacuated and back-filled with argon. To this mixture was added 68 mL of tBuOH followed by the addition of DBU (0.10 mL, 0.68 mmol, 0.10 equiv). The resulting mixture was allowed to stir at rt for 14 h, concentrated under reduced pressure, and purified by flash chromatogrphay (4:1 hexanes/EtOAc) to afford the lactam product as an 8:1 mixture of diastereomers (1.8 g, 4.2 mmol, 61% yield). mp 135–136 °C; 1H NMR (400 MHz, CDCl3) δ 7.96 (d, 2H, J = 8.9 Hz), 7.28–7.21 (m, 3H), 7.15–7.04 (m, 2H), 6.86 (d, 2H, J = 8.1 Hz), 6.39 (d, 1H, J = 15.7 Hz), 5.58 (dd, 1H, J = 15.7, 8.2 Hz), 5.31 (m, 1H), 4.93* (m, 1H), 3.99 (m, 1H), 3.34* (m, 1H), 3.83 (s, 3H), 3.86* (s, 3H), 2.98 (dd, 1H, J = 17.2, 13.2 Hz), 3.15* (dd, 1H, J = 17.6, 8.4 Hz), 2.72 (dd, 1H, J = 17.2, 7.2 Hz), 2.61* (dd, 1H, J = 17.6, 3.2 Hz), 2.51 (s, 3H), 2.61* (s, 3H), 2.22 (s, 3H), 2.37* (s, 3H); 13C NMR (100 MHz, CDCl3) δ172.1, 164.1, 135.8, 135.7, 134.7, 131.3, 131.1, 130.3, 129.4, 128.9, 128.7, 128.1, 127.9, 126.9, 126.7, 126.6, 123.2, 114.1, 65.8, 55.8, 43.5, 35.2; IR (thin film) v 3054, 2987, 2305, 1735, 1654, 1596, 1498, 1421, 1265, 1166, 1092, 898, 835, 739 cm-1; ESI-MS: 434.1 (M+H)+. (*indicates peaks assigned to the minor diastereomer).
cis-4-Phenyl-5-styrylpyrrolidin-2-one (11). Naphthalene (1.8 mmol, 0.24 g, 12 equiv) was dissolved in 2.0 ml DME. Na (0.043 g, 1.8 mmol, 12 equiv) was added to the solution, and the mixture was stirred at room temperature for 2 h to form a dark green solution. The protected lactam (0.065 g, 0.15 mmol, 1.0 equiv) dissolved in 1.0 mL of DME was added to the solution at 0 °C, and the mixture was warmed up slowly to room temperature. After 1 h, water was added and the mixture was extracted with Et2O. The combined extracts were washed with brine, dried over MgSO4, and concentrated. Purification by flash chromatography (1:1 hexane/EtOAc) afforded the desired product (24 mg, 0.092 mmol, 61% yield). mp 70–71 °C; 1H NMR (400 MHz, CDCl3) δ 7.34–7.11 (m, 10 H), 6.46 (d, 1H, J = 16.0 Hz), 6.32 (s, 1H), 5.59 (dd, 1H, J = 16.0, 6.9 Hz), 4.60 (t, 1H, J = 7.2 Hz), 3.94 (dd, 1H, J = 16.2, 8.3 Hz), 2.80–2.67 (m, 2H); 13C NMR (100 MHz, CDCl3) δ 177.7, 138.4, 136.3, 131.9, 128.7, 128.3, 128.0, 127.7, 127.5, 127.3, 126.6, 94.6, 60.2, 45.5, 35.4; IR (thin film) v 3424, 3055, 2987, 2306, 1702, 1422, 1265, 896, 739, 706 cm-1; EI-MS: 263.1 (M+).
cis-1-Methyl-4-phenyl-5-styrylpyrrolidin-2-one (12). To lactam 11 (20.0 mg, 0.074 mmol, 1.00 equiv) in 0.5 mL of THF, was added NaH (2.1 mg, 0.088 mmol, 1.2 equiv), followed by the addition of MeI (0.22 mmol, 0.14 mL, 3.0 equiv). The reaction mixture was stirred at room temperature for 40 min. Water was added and the mixture was extracted with Et2O, washed with brine, and dried over Na2SO4. The crude product was purified by flash chromatography (1:1 hexane/EtOAc) to provide the methylated product 12 (0.069 mmol, 19 mg, 93% yield). mp 94–96 °C; 1H NMR (400 MHz, CDCl3) δ 7.33–7.11 (m, 10 H), 6.40 (d, 1H, J = 15.8 Hz), 5.52 (dd, 1H, J = 15.8, 8.1 Hz), 4.42–4.37 (m, 1H,), 3.84 (dd, 1H, J = 16.6, 8.5 Hz), 2.86 (dd, 1H, J = 16.0, 9.2 Hz), 2.86 (s, 3H), 2.57 (dd, 1H, J = 16.0, 8.5 Hz); 13C NMR (100 MHz, CDCl3) δ 174.6, 138.5, 136.2, 133.5, 128.8, 128.7, 128.4, 128.2, 127.4, 126.7, 125.8, 76.9, 67.0, 43.2, 35.7, 28.6; IR (thin film) v 3055, 2986, 2306, 1686, 1421, 1266, 896, 748, 704 cm-1; EI-MS: 277.1 (M+).
cis-5-(Hydroxy(phenyl)methyl)-1-methyl-4-phenylpyrrolidin-2-one (13). Lactam 12 (44 mg, 0.16 mmol, 1.0 equiv) in 2.0 ml CH2Cl2 was treated with a stream of O3 in O2 for 30 min. After purging with O2 for 20 min, DMS was added at –78 °C and the mixture stirred for 1 h at –78 °C and 2 h at 0 °C. To this solution was added PhMgBr (3.0 equiv, 0.48 mmol) at 0 °C and stirred for 30 min at 0 °C. The mixture was quenched by the addition of 2 N HCl and extracted with EtOAc. The combined extracts were washed with brine, dried over Na2SO4, and concentrated. Purification by flash chromatography (1:1 hexane/ EtOAc) afforded the desired product (28 mg, 0.10 mmol, 63% yield over two steps). The spectral data matched a previous report. mp 188–190 °C; 1H NMR (400 MHz, DMSO-d6) δ 7.46–7.17 (m, 10 H), 5.36 (d, 1H, J = 4.9 Hz), 4.26(d, 1H, J = 4.8 Hz), 4.16 (d, 1H, J = 8.0 Hz), 3.80 (m, 1H), 3.05 (dd, 1H, J = 15.0, 13.4 Hz), 2.26 (dd, 1H, J = 15.0, 8.4 Hz), 2.21 (s 3H); 13C NMR (100 MHz, CDCl3) δ 176.0, 142.4, 138.3, 129.0, 128.7, 128.3, 127.6, 125.4, 72.0, 70.6, 43.6, 35.2, 31.3; IR (thin film) v 3371, 3054, 2987, 2305, 1682, 1422, 1266, 896, 748, 704 cm-1; ESI-MS: 282.1 (M+H)+.
ACKNOWLEDGEMENTS
This work was conducted at the University of California–Santa Barbara and was supported by an NSF CAREER award to J.W.B. M.R. received a postdoctoral fellowship from the German Academic Exchange Service (DAAD). We thank Yi-Huang for assistance in the preparation of this manuscript.
References
1. C. Zhu and J. Zhang, Yaoxue Xuebao, 2003, 38, 654.
2. Y.-R. Chen, M.-H. Yang, L. Huang, T. Geng, and U. Benz, Ger. Offen., 3431257 (EP 172514).
3. X. Z. Zhu, X.-Y. Li, and J. Liu, Eur. J. Pharmacol., 2004, 500, 221. CrossRef
4. K. Tang and J.-T. Zhang, Neurol. Res., 2003, 25, 713. CrossRef
5. M.-H. Yang, Y.-Y. Chen, and L. Huang, Chin. Chem. Lett., 1991, 2, 291.
6. M.-H. Yang, Y.-Y. Chen, and L. Huang, Phytochemistry, 1988, 27, 445. CrossRef
7. L. Yang, Q. Y. Zheng, D.-X. Wang, Z.-T. Huang, and M.-X. Wang, Org. Lett., 2008, 10, 2461. CrossRef
8. W. Hartwig and L. Born, J. Org. Chem., 1987, 52, 4352; CrossRef M. W. Cappi, W.-P. Chen, R. W. Flood, Y.-W. Liao, S. M. Roberts, J. Skidmore, J. A. Smith, and N. M. Williamson, Chem. Comm., 1998, 1159; CrossRef T. Yakura, Y. Matsumura, and M. Ikeda, Synlett, 1991, 343. CrossRef
9. For recent reviews on enantioselective annulations catalyzed by chiral NHCs, see: P.-C. Chiang and J. W. Bode, in N-Heterocyclic Carbenes, The Royal Society of Chemistry, 2011, pp. 399–435; V. Nair, R. S. Menon, A. T. Biju, C. R. Sinu, R. R. Paul, A. Jose, and V. Sreekumar, Chem. Soc. Rev. 2011, 40, 5336; CrossRef A. Grossmann and D. Enders, Angew. Chem. Int. Ed., 2012, 51, 314; CrossRef X. Bugaut and F. Glorius, Chem. Soc. Rev., 2012, 41, 3511; CrossRef H. U. Vora, P. Wheeler and T. Rovis, Adv. Synth. Catal., 2012, 354, 1617; CrossRef J. Douglas, G. Churchill, and A. D. Smith, Synthesis, 2012, 2295. CrossRef
10. M. He and J. W. Bode, Org. Lett., 2005, 7, 3131. CrossRef
11. J. R. Struble, J. Kaeobamrung, and J. W. Bode, Org. Lett., 2008, 10, 957. CrossRef
12. P.-C. Chiang and J. W. Bode, TCI Mail, 2011, 149, 2.
13. J. R. Struble and J. W. Bode, Tetrahedron, 2008, 64, 6961. CrossRef
14. V. Nair, V. Varghese, B. P. Babu, C. R. Sinu, and E. Suresh, Org. Biomol. Chem., 2010, 8, 761; CrossRef D. E. A. Raup, B. Cardinal-David, D. Holte, and K. A. Scheidt, Nat. Chem., 2010, 2, 766; CrossRef X. Zhao, D. A. DiRocco, and T. Rovis, J. Am. Chem. Soc., 2011, 133, 12466; CrossRef J. Dugal-Tessier, E. A. O'Bryan, T. B. H. Schroeder, D. T. Cohen, and K. A. Scheidt, Angew. Chem. Int. Ed., 2012, 51, 4963; CrossRef B. Zhang, P. Feng, L.-H. Sun, Y. Cui, S. Ye, and N. Jiao, Chem. Eur. J., 2012, 18, 9198. CrossRef