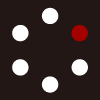
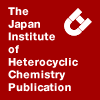
HETEROCYCLES
An International Journal for Reviews and Communications in Heterocyclic ChemistryWeb Edition ISSN: 1881-0942
Published online by The Japan Institute of Heterocyclic Chemistry
e-Journal
Full Text HTML
Received, 8th October, 2012, Accepted, 26th October, 2012, Published online, 31st October, 2012.
DOI: 10.3987/COM-12-12600
■ Synthesis of Neodesmosine, a Crosslinking Pyridinium Amino Acid of Elastin, via a Negishi Cross-Coupling
Hiroto Yanuma and Toyonobu Usuki*
Department of Materials and Life Sciences, Faculty of Science and Technology, Sophia University, 7-1 Kioicho, Chiyoda-ku, Tokyo 102-8554, Japan
Abstract
Neodesmosine, isolated from the hydrolysate of bovine ligamentum nuchae, is a crosslinking pyridinium amino acid of elastin. In this study, the first total synthesis of neodesmosine is reported via a Negishi cross-coupling reaction between 3,5-dihalogenated pyridines and the corresponding iodo amino acid.INTRODUCTION
Elastic fibers exist in vertebrate tissues and organs such as the lung, skin, blood vessels, and heart, and play an important role in providing their elasticity and stretch properties.1 These unique functions are mainly derived from their self-assembling nature and crosslinked structure. Elastin, the main component of elastic fibers, is an extremely insoluble extracellular matrix protein and consists of soluble precursor tropoelastin monomers connected in a three-dimensional crosslinked network by amino acids. The elastin crosslinkers are thus significant amino acids with respect to their elasticity and stretch properties.
As one of the crosslinking pyridinium amino acids of elastin, neodesmosine (1, Figure 1) was isolated from the acid hydrolysate of bovine ligamentum nuchae.2 Desmosine (2) and isodesmosine (3), known as attractive biomarkers for the diagnosis of COPD (chronic obstructive pulmonary disease),3 have also been identified as major crosslinking pyridinium amino acids (Figure 1).4 It has been known that the formation of the crosslinking amino acids occurs spontaneously after oxidative transformation of the lysine residues of elastin by lysyl oxidase.5 Although a model structure for elastin has been proposed,6 the three-dimensional structure of elastin including the crosslinking networks remains unknown due to its insoluble nature. Therefore, the chemical synthesis of these crosslinking amino acids would aid in the elucidation of the entire structure.
Recently, the first total synthesis of desmosine 2 starting from 4-hydroxypyridine or 3,5-dibromopyridine was achieved in our laboratory.7 The synthesis relied on palladium-catalyzed cross-coupling reactions between the pyridine cores and the corresponding segments as key steps. Herein, the first total synthesis of neodesmosine is described via a Negishi cross-coupling reaction8 between 3,5-dihalogenated pyridines and the corresponding iodo amino acid.
RESULTS AND DISCUSSION
The target molecule 1 consists of a pyridine core and two types of amino acids. Toward the total synthesis, our route commenced with optimization of the Negishi cross-coupling reaction of 3,5-dihalogenated pyridines 4/5 and protected γ-iodoalkylated L-glycine 6, which can be derived from commercially available 4-benzoxyl-(S)-3-[(tert-butoxycarbonyl)amino]-4-oxobutanoic acid in two steps (Table 1).9 Firstly, 3,5-diiodopyridine 5 was prepared from commercially available 3,5-dibromopyridine 4 using an excess amount of KI in the presence of CuI.10 The reaction was run for a week in order to complete the conversion of the bromide to the iodide and provide pure product 5, because the polarity of the desired product was the same as that of the starting material 4. Thus, the desired 5 was obtained in 63% yield.
The Negishi cross-coupling reactions were then investigated (Table 1, entries 1-6). For the insertion of zinc into protected γ-iodoalkylated L-glycine 6, a modified protocol was applied, in which the extra zinc from the organozinc reagent can be removed via centrifugation.11 The reaction between 3,5-dibromopyridne 4 and the pure organozinc reagent, protected γ-iodozinc-alkylated L-glycine 7, was then carried out using 10 mol% Pd2(dba)3 with 40 mol% P(2-furyl)312 at 50 °C for 24 h in order to afford the desired dicoupled product 8 in 39% yield (entry 1). When the reaction was run with 20 mol% Pd-PEPPSI-IPr, which was developed by Organ and co-workers,13 the dicoupled product 8 and monocoupled byproduct 9 were obtained in 59% and 30% yield, respectively (entry 2). However, the yield of 8 was decreased in the presence of LiBr and LiCl as an additive in order to activate the organozinc reagents (entries 3 and 4). In the case of 3,5-diiodopyridine 5, the Negishi cross-coupling reaction with 20 mol% Pd-PEPPSI-IPr gave the product 8 in only 32% yield (entry 5). However, the reaction run with 10 mol% Pd2(dba)3 and 40 mol% P(2-furyl)3 afforded 8 in 76% yield (entry 6). An air and moisture stable NHC-catalyst, Pd-PEPPSI-IPr can be useful for sp3-sp3 bond formation, and thus is known as a stronger catalyst than those used under normal Negishi conditions. Therefore, in the case of 3,5-diiodopyridine, the conditions with Pd-PEPPSI-IPr might be hard on the substrate, resulting in decomposition.
Dicoupled product 8 can also be prepared from 3,5-dialkyl-4-bromopyridine 10, which was obtained in our previous synthetic study of desmosine 2.7b Hydrodehalogenation (hydrodebromination) of 10 was thus carried out using zinc in DMF to give 8 in 56% yield (Scheme 1).
Formation of the pyridinium salt of the obtained 8 with protected ω-iodoalkylated L-glycine 11, which can be obtained from commercially available 5-benzoxyl-(S)-4-[(tert-butoxycarbonyl)amino]-5-oxopentanoic acid in seven steps,9 was then conducted in MeNO2 at 80 °C to produce 12 in 79% yield (Scheme 2).14 After reduction of the three benzyl groups with H2 and Pd/C to give the tricarboxylic acid 13 in 89% yield, the three t-butoxycarbonyl protecting groups were successfully removed using TFA to provide the crude product. Purification by reversed-phase HPLC [column: Cosmosil 5C18-Ar-II (10 × 250 mm); mobile phase: MeOH/H2O (1/9, linear gradient); flow rate: 1.5 mL/min; detection: 270 nm; temperature: 40 °C; Rt (1) = 7.3-8.5 min] afforded pure neodesmosine 1 in 79% yield. Spectroscopic data obtained for 1, including that from 1H NMR, MS, and UV analyses, were in good agreement with those reported for natural 1.2
In summary, the total synthesis of neodesmosine 1, a crosslinking pyridinium amino acid of elastin, has been achieved for the first time. The synthesis was conducted in 33% yield over four steps starting from 3,5-dibromopyridine 4 with a Negishi cross-coupling reaction as the key transformation. The synthesis described above is currently being applied to the preparation of other crosslinking amino acids5 in order to elucidate the three dimensional structure of elastin fibers.
EXPERIMENTAL
All non-aqueous reactions were conducted under an atmosphere of nitrogen with magnetic stirring using dry solvents unless otherwise indicated. Dimethylformamide (DMF) was dried by distillation and stored over activated molecular sieves. Trimethylsilyl chloride (TMSCl), diisopropylethylamine (iPr2NEt), and nitromethane (MeNO2) were dried by distillation. Dehydrated methanol (MeOH) for the reactions was purchased from Kanto Chemicals (Tokyo, Japan). All reagents were obtained from commercial suppliers and used without further purification unless otherwise stated. Centrifugation was performed by LMS Mini Centrifuge MCF-2360 (6,600 rpm). Analytical thin layer chromatography (TLC) was performed on Silica gel 60 F254 plates produced by Merck. Column chromatography was performed with acidic Silica gel 60 (spherical, 40-50 µm) or neutral Silica gel 60N (spherical, 40-50 µm) produced by Kanto Chemicals.
Melting points were measured by an AS one ATM-01 apparatus. Optical rotations were measured on a JASCO P-2200 digital polarimeter at the sodium lamp (λ = 589 nm) D line and are reported as follows: [α]DT (c g/100 mL, solvent). UV spectra were recorded on a JASCO V-560 UV/VIS spectrophotometer and are reported in wavelengths (nm). Infrared (IR) spectra were recorded on a JASCO FT-IR 4100 spectrometer and are reported in wavenumbers (cm-1). 1H and 13C NMR spectra were recorded on a JEOL JNM-EXC 300 spectrometer (300 MHz) or on a JEOL JNM-ECA 500 spectrometer (500 MHz). 1H NMR data are reported as follows: chemical shift (δ, ppm), integration, multiplicity (s, singlet; d, doublet; t, triplet; q, quartet; m, multiplet), coupling constants (J) in Hz, assignments. 13C NMR data are reported in terms of chemical shift (δ, ppm). EI-MS spectra were recorded on a Shimadzu GCMS QP-5050 instrument. ESI-MS spectra were recorded on a JEOL JMS-T100LC instrument. Mass spectroscopic data were reported in m/z. JASCO HPLC systems PU-2085, MD-2010, and CO-2060 were used for the purification of neodesmosine 1.
The carbon numbering on 1H NMR of all compounds is corresponding with 1.
3,5-Diiodopyridine 5: The mixture of 3,5-dibromopyridine 4 (500 mg, 2.1 mmol), KI (11.5 g, 69.1 mmol) and CuI (1.05 g, 55.1 mmol) was placed in a 100 mL two-necked flask. DMF (45 mL) was added, and the flask was heated at 180 °C under N2. After 1 week, the flask was allowed to cool to room temperature and the solution was poured onto ice water. The precipitate was filtered, and dissolved in CH2Cl2/pyridine (5/1) solution. Saturated Na-EDTA solution was added to remove excess Cu component from the organic layer, and the mixture was stirred overnight. After removal of the organic layer, the aqueous layer was then extracted with ethyl acetate (EtOAc). The combined organic layers were washed with brine, dried over Na2SO4, and concentrated in vacuo to give the crude product as a green solid. Purification by flash column chromatography (hexane/EtOAc = 10/1) afforded the pure 5 (438.3 mg, 1.32 mmol, 63%) as a colorless solid. The remaining colorless solid was recrystallized from benzene to give a colorless solid; Rf 0.57 (hexane/EtOAc = 5/1); mp 165-168 °C; IR (KBr, cm-1) 3067, 2999, 1527, 1398, 1107, 1068, 999, 876, 723, 688, 636; 1H NMR (300 MHz, CDCl3) δ 8.93 (2H, s, H2/6), 8.36 (1H, t, J = 2.1 Hz, H4); 13C NMR (75 MHz, CDCl3) δ 154.3, 151.6; EI-MS (m/z) calcd for C5H3I2 N [M]+ 330.84, found 330.70.
(2S,2’S)-Benzyl 4,4’-(pyridine-3,5-diyl)bis(2-(tert-butoxycarbonylamino)butanoate) 8 and 2S-benzyl-4-(5-bromopyridin-3-yl)-(2-tert-butoxycarbonylamino)butanoate 9: Zinc dust (200 mg, 3.0 mmol) was placed in a nitrogen-purged 1.5 mL Eppendorf microtube. Dry DMF (150 µL) and TMSCl (60.0 μL, 0.47 mmol) were added to the microtube, and the resulting mixture was stirred vigorously for 15 min at room temperature. After stirring, the solution was removed by microsyringe. The remaining solid was dried using a hot air gun at reduced pressure. The activated zinc was then cool to room temperature. A solution of benzyl 2-(S)-((tert-butoxycarbonyl)amino)-3-iodobutanoate 69 (210 mg, 0.5 mmol) in dry DMF (150 µL and washed with 100 µL DMF) was added to the activated zinc. The reaction mixture was stirred at room temperature for 1 h. The insertion of zinc to give the organozinc reagent 7 was monitored by TLC analysis (hexane/EtOAc = 5/1). After completion of the insertion, the zinc solution was allowed to settle using a centrifuge for 1 min at room temperature.
The solution of organozinc reagent 7 was removed via microsyringe with 200 µL DMF and added to a 10 mL flask containing Pd-PEPPSI-IPr (13.6 mg, 20 mol%) and the 3,5-dibromopyridine 4 (23.9 mg, 0.10 mmol, 1.0 eq). After stirring for 24 h at 50 °C, the reaction mixture was diluted with EtOAc and quenched with a saturated aqueous NH4Cl solution. The aqueous layer was then extracted with EtOAc. The combined organic layers were washed with brine, dried over Na2SO4, and concentrated in vacuo to give the crude product as a yellow oil. Purification by flash column chromatography (hexane/EtOAc = 1/1 → 3/1) afforded the pure dicoupled product 8 (39.4 mg, 59.5 µmol, 59%) as a yellow oil and byproduct monocoupled 9 (13.5 mg, 30.0 µmol, 30%) as a yellow powder, respectively. 8: Rf 0.52 (hexane/EtOAc = 1/2); [α]D20 +10.3 (c 0.1, CHCl3); IR (neat, cm-1) 3354, 2976, 2932, 1713, 1500, 1455, 1366, 1252, 1166, 1050, 1026, 916, 865, 751, 699, 604, 461; 1H NMR (300 MHz, CDCl3) δ 8.34 (2H, s, H2/6), 7.35 (10H, s, Bn), 5.23-5.10 (6H, m, Bn, NH), 4.36 (2H, d, J =5.4 Hz, H15/15’), 2.66-2.58 (4H, m, H13/13’), 2.17-2.07 (2H, m, H14/14’), 1.94-1.86 (2H, m, H14/14’), 1.44 (18H, s, tBu); 13C NMR (75 MHz, CDCl3) δ 172.3, 155.4, 147.7, 136.2, 135.4, 135.3, 128.8, 128.7, 128.6, 80.2, 67.3, 53.3, 34.2, 28.7, 28.4; ESI-HRMS (m/z) calcd for C37H47N3NaO8 [M+Na]+ 684.3260, found 684.3256. 9: Rf 0.39 (hexane/EtOAc = 2/1); 1H NMR (300 MHz, CDCl3) δ 8.51 (1H, s, H2/6), 8.27 (1H, s, H2/6), 7.56 (1H, s, H4), 7.37 (5H, s, Bn), 5.25-5.10 (3H, m, Bn, NH), 4.40 (1H, m, H15), 2.68-2.47 (2H, m, H13), 2.13 (1H, m, H14), 1.92 (1H, m, H14), 1.45 (18H, s, tBu); 13C NMR (75 MHz, CDCl3) δ 172.1, 148.9, 148.1, 138.6, 135.3, 128.9, 80.4, 67.5, 53.2, 34.1, 28.4; EI-MS (m/z) calcd for C13H18BrN2O2 [M-CO2Bn]+ 313.06, found 313.05, calcd for C13H18BrN2O2 [M-CO2Bn]+ 313.06, found 313.05; C17H16BrN2O3 [M-tBu]+ 375.03, found 375.05.
(2S,2’S)-Benzyl 4,4’-(pyridine-3,5-diyl)bis(2-(tert-butoxycarbonylamino)butanoate) 8: Zinc dust (200 mg, 3.0 mmol) was placed in a 10 mL flask. Dry DMF (150 µL) and TMSCl (60.0 μL, 0.47 mmol) were added to the flask, and the mixture was stirred vigorously for 15 min at room temperature. After stirring, the solution was removed by microsyringe. A solution of (2S,2’S)-benzyl 4,4’-(4-bromopyridine-3,5-diyl)bis(2-(tert-butoxycarbonylamino)butanoate) 107b (21.1 mg, 28.5 µmol) in dry DMF (100 µL and washed with 100 µL DMF) was added to the activated zinc. After stirring for 24 h at 50 °C, the reaction mixture was diluted with EtOAc and quenched with a saturated aqueous NH4Cl solution. The aqueous layer was then extracted with EtOAc. The combined organic layers were washed with brine, dried over Na2SO4, and concentrated in vacuo to give the crude product as a yellow oil. Purification by flash column chromatography (hexane/EtOAc = 1/1 → 3/1) afforded the pure dicoupled product 8 (10.6 mg, 16.0 µmol, 56%) as a yellow oil.
3,5-Bis((S)-4-(benzyloxy)-3-(tert-butoxycarbonylamino)-4-oxobutyl)-1-((S)-6-(benzyloxy)-5-(tert-butoxycarbonylamino)-6-oxohexyl)pyridinium 12: A mixture of 8 (41.9 mg, 63.3 µmol) and benzyl 2-(S)-((tert-butoxycarbonyl)amino)-6-iodohexanoate 119 (56.6 mg, 126.6 µmol) in MeNO2 (1.5 mL) was heated at 80 °C for 24 h. The reaction mixture was concentrated in vacuo. Purification on silica gel column chromatography (hexane/EtOAc = 1/1 → CH2Cl2/MeOH = 10/1) afforded 12 (55.6 mg, 50.1 µmol, 79%) as a yellow solid; Rf 0.58 (CH2Cl2/MeOH = 10/1); [α]D20 +3.2 (c 0.1, CHCl3); IR (KBr, cm-1) 3371, 2976, 2932, 1710, 1499, 1455, 1366, 1256, 1166, 1051, 1026, 864, 752, 699, 581; 1H NMR (300 MHz, CDCl3) δ 8.93 (2H, s, H2/6), 7.48-7.30 (20H, m, Bn), 6.05 (1H, s, 16NH), 5.63 (2H, m, 20NH/20’NH), 5.24-5.12 (4H, m, Bn), 4.72-4.70 (2H, m, H7), 4.58 (1H, m, H16), 4.35-4.27 (3H, m, H11/20/20’), 3.16 (2H, t, J = 5.1 Hz, H15), 2.87 (4H, m, H18/18’), 2.22-2.02 (6H, m, H8/19/19’), 1.76-1.62 (4H, m, H9/10), 1.42-1.45 (36H, s, tBu); 13C NMR (75 MHz, CDCl3) δ 172.3, 171.7, 155.7, 145.6, 142.1, 142.0, 135.4, 135.2, 128.7, 128.6, 128.5, 80.3, 67.5, 67.2, 61.5, 52.6, 32.8, 31.9, 30.8, 28.8, 28.4, 22.2; ESI-HRMS (m/z) calcd for C55H73N4O12 [M]+ 981.5225, found 981.5218.
3,5-Bis((S)-3-(tert-butoxycarbonylamino)-3-carboxypropyl)-1-((S)-5-(tert-butoxycarbonylamino)-5-carboxypentyl)pyridinium 13: A solution of 12 (50.1 mg, 45.2 µmol) in MeOH (1.0 mL) was treated with 10% Pd/C (271.1 mg, 254.7 µmol) and hydrogenated at balloon pressure at room temperature. After stirring for 24 h at room temperature, the insoluble was separated by filtration through a Celite pad on neutral silica gel eluting with MeOH. The filtrate was then concentrated in vacuo. Concentration of the filtrate yielded tetracarboxylic acid 13 (33.7 mg, 40.2 µmol, 89%) as a yellow solid. The product was used to the next reaction without further purification; 1H NMR (300 MHz, CD3OD) δ 8.73 (2H, s, H2/6), 8.37 (1H, s, H4), 4.56 (2H, m, H7), 3.93 (3H, m, H11/15/15’), 2.90 (4H, m, H13/13’), 2.16-2.05 (6H, m, H8/14/14’), 1.77 (2H, m, H10), 1.41 (38H, m, tBu/H9); ESI-HRMS (m/z) calcd for C34H55N4O12 [M]+ 711.3816, found 711.3816.
(+)-Neodesmosine 1; 3,5-bis((S)-3-amino-3-carboxypropyl)-1-((S)-5-amino-5-carboxypentyl)pyridinium: A mixture of trifluoroacetic acid (TFA) and distilled water (3.0 mL, 95/5 ratio) was added to the crude tricarboxylic acid 13 (14.7 mg 17.5 µmol) at room temperature and stirred for 2 h. The solvent was concentrated in vacuo. Purification on C18 silica gel column chromatography (0.1% TFA in distilled water) afforded the crude product as a yellow oil (27.7 mg). The crude product was then purified by reversed phase HPLC system. The conditions were as follows: column, Cosmosil 5C18-AR-II (10 × 250 mm, Nacalai tesque, Kyoto); solvent, linear gradient of 10% MeOH and 90% H2O; flow rate, 1.5 mL/min; detection, 270 nm; temperature, 40 °C; Rt = 7.3-8.5 min (neodesmosine 1). As a result, 7.3 mg of pure neodesmosine 1 was obtained as a colorless oil in 79%. Rf 0.32 [MeOH (0.1% TFA)/H2O (0.1% TFA) = 1/9]; [α]D20 +8.7 (c 0.10, H2O); UV λmax: 270 nm in H2O (lit. value: λmax: 270 nm in 0.1 M HCl)3; 1H NMR (500 MHz, D2O) δ 8.65 (2H, s, H2/6), 8.37 (1H, s, H4), 4.58 (2H, t, J = 7.0 Hz, H7), 3.78 (2H, t, J = 6.0 Hz, H15/15’), 3.73 (2H, t, J = 6.0 Hz, H11/11’), 2.97 (4H, m, H13/13’), 2.23 (4H, m, H14/14’), 2.06 (2H, t, J = 7.4 Hz, H8), 1.93-1.88 (2H, m, H10), 1.52-1.36 (2H, m, H9); 13C NMR (75 MHz, D2O) δ 175.2, 174.8 (C12/16), 146.2 (C4), 142.5 (C2/6 or 3/5), 142.2 (C2/6 or 3/5), 61.8 (C7), 55.0 (C11), 54.7 (C15), 31.7 (C14/14’), 30.7 (C8 or 10), 30.4 (C8 or 10), 28.4 (C13/13’), 21.7 (C9); ESI-LRMS (m/z) calcd for C19H31N4O6 [M]+ 411.22, found 411.24. ESI-HRMS (m/z) calcd for C19H31N4O6 [M]+ 411.2244, found 411.2244. These data were good agreement with natural 1.2b
ACKNOWLEDGEMENTS
This work was supported by a Grant-in-Aid for Young Scientists (B) from the Ministry of Education, Culture, Sports, Science and Technology (MEXT) of Japan (KAKENHI Grant Number 22710224), the Shimadzu Science Foundation, the SEI Group CSR Foundation, and the Naito Science Foundation.
References
1. (a) J. Rosenbloom, W. R. Abrams, and R. Mecham, FASEB J., 1993, 7, 1208; (b) L. Debelle and A. M. Tamburro, Int. J. Biochem. Cell Biol., 1999, 31, 261. CrossRef
2. (a) Y. Nagai, Conn. Tissue, 1983, 14, 112; (b) F. Nakamura and K. Suyama, Agric. Biol. Chem., 1991, 55, 547. CrossRef
3. (a) S. Ma, S. Lieberman, G. M. Turino, and Y. Y. Lin, Proc. Natl. Acad. Sci., U.S.A., 2003, 100, 12941; CrossRef (b) S. Ma, Y. Y. Lin, and G. M. Turino, Chest, 2007, 131, 1363; CrossRef (c) S. Ma, G. M. Turino, and Y. Y. Lin, J. Chromatogr. B, 2011, 879, 1893. CrossRef
4. (a) S. M. Partridge, D. F. Elsden, and J. Thomas, Nature, 1963, 197, 1297; CrossRef (b) J. Thomas, D. F. Elsden, and S. M. Partridge, Nature, 1963, 200, 651. CrossRef
5. M. Akagawa and K. Suyama, Connect. Tissue Res., 2000, 41, 131. CrossRef
6. P. Brown-Augsburger, C. Tisdale, T. Broekelmann, C. Sloan, and R. P. Mecham, J. Biol. Chem., 1995, 270, 17778. CrossRef
7. (a) T. Usuki, H. Yamada, T. Hayashi, H. Yanuma, Y. Koseki, N. Suzuki, Y. Masuyama, and Y. Y. Lin, Chem. Commun., 2012, 48, 3233; CrossRef (b) H. Yanuma and T. Usuki, Tetrahedron Lett., 2012, 53, 5920. CrossRef
8. E.-i. Negishi, Acc. Chem. Res., 1982, 15, 340. CrossRef
9. Y. Koseki, H. Yamada, and T. Usuki, Tetrahedron: Asymmetry, 2011, 22, 580. CrossRef
10. Y. Kosaka, H. M. Yamamoto, A. Nakao, M. Tamura, and R. Kato, J. Am. Chem. Soc., 2007, 129, 3054. CrossRef
11. T. Usuki, H. Yanuma, T. Hayashi, H. Yamada, N. Suzuki, and Y. Masuyama, J. Heterocycl. Chem., DOI: 10.1002/jhet.1807. CrossRef
12. V. Farina and B. Krishnan, J. Am. Chem. Soc., 1991, 113, 9585. CrossRef
13. C. Valente, M. E. Belowich, N. Hadei, and M. G. Organ, Eur. J. Org. Chem., 2010, 23, 4343.
14. (a) R. X.-F. Ren, N. Sakai, and K. Nakanishi, J. Am. Chem. Soc., 1997, 119, 3619; CrossRef (b) C. Sicre and M. M. Cid, Org. Lett., 2005, 7, 5737. CrossRef