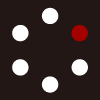
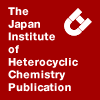
HETEROCYCLES
An International Journal for Reviews and Communications in Heterocyclic ChemistryWeb Edition ISSN: 1881-0942
Published online by The Japan Institute of Heterocyclic Chemistry
e-Journal
Full Text HTML
Received, 26th October, 2012, Accepted, 15th November, 2012, Published online, 22nd November, 2012.
DOI: 10.3987/COM-12-12611
■ The New Convenient Synthesis of Novel γ-Alkylidenebutenolides from 6-Aminopenicillanic Acid
Bin Yu, En Zhang, Yuan Fang, Xiao-nan Sun, Jing-li Ren, De-quan Yu,* and Hong-min Liu*
New Drug Research & Development Center, School of Pharmaceutical Science, Zhengzhou University, Zhengzhou 450001, China
Abstract
Butenolides have good biological activity and serve as the important unit of pharmaceutical intermediates, of which γ-alkylidenebutenolides have gained wide attention over the last past decades particularly. A simple and practical method for the synthesis of butenolide 6 and its 5-substituted analogues 7a-t via vinylogous aldol reaction from 6-aminopenicillanic acid (6-APA) with the ratio of Z/E isomers between 1.1/1.0 and 1.0/0 under mild conditions in 6 steps was first reported. Besides, we also reported the methanolysis behaviors of azetidinone fused oxazoline derivatives. All the synthesized γ-alkylidenebutenolides are new and are currently being evaluated for their biological activities.Due to their large abundance and their biological interest and potential medicinal value, butenolides have been extensively studied. Many natural products, including prominent compounds such as freelingyne,1 rubrolides,2 tetrenolin3 (Figure 1), belong to the pharmacologically important group of γ-alkylidenebutenolides (γ-AIBs), which have gained an increasing amount of attention over the last past decades.4 Most of them exhibit various interesting biological activities, such as antibacterial, anticancer, antibiotic and so on.5 For example, freelingyne displays antibiotic activity6 and rubrolides, which are marine tunicate metabolites, exhibit potent antibiotic activities in vitro.5b Principally, the efficient synthetic strategies towards the γ-AIBs mainly focus on five major respects, which were reviewed comprehensively by Rao,7 Negishi,8 Bruckner,9 and Langer.10 Many efforts have been dedicated to seek highly potent γ-AIBs on their pharmacological activities.
Our previous work indentified that γ-AIBs based derivatives showed moderate to excellent biological activities, such as antitumor activity,11 α-glucosidase inhibitory activity,12 and antagonist of histamine.13 Besides, γ-AIBs could regulate the intercellular Na+ concentration.14 As part of our ongoing effort to indentify new biological agents, we are interested in investigating novel γ-AIBs, expecting to find lead structures for further preclinical research. Herein we report an efficient route to access the novel γ-AIBs with a similar amino acid side chain starting from commercially available 6-aminopenicillanic acid (6-APA) (Scheme 1).
Initially, penicillin sulfoxide 2 was prepared from commercially available 6-APA in one-pot procedure. Acylation of 6-APA, oxidation, followed by protection of carboxyl group to give compound 2 in 89% yield in ice bath, When the reaction temperature increased to room temperature, it gave a complex mixture due to the instability of β-lactam ring. Oxazoline 3 was successfully synthesized from penicillin sulfoxide 2 in 85% yield. As reported by Yamamoto et al.,15 acid was necessary for formation of 3. After screening the acids, such as benzoic acid, formic acid, niacin, and squaric acid, we found that a catalytic amount of squaric acid (0.05 eq) was efficient for the formation of oxazoline 3 due to its low pk1 value and little nucleophilicity. N,N-Dimethylacetamide (DMA), as a co-solvent, was found essential and an optimal amount of DMA was 5-10% of the solvent. Excess amount of DMA caused double-bond isomerization at the butenoate side chain, which was in agreement with the literature report.15 The allylic chlorination of oxazoline 3 by simple addition of chlorine, followed by treatment with aqueous sodium hydrogen carbonate proceeded well and gave allylic chloride 4 in 75% yield via an ene-type reaction mechanism.16
As part of our continuing efforts to find an efficient method for synthesizing the oxacephem derivatives, we found that treatment of 4 with methanol gave an unexpected dipeptide 5 in high yield without any catalyst at room temperature due to the formation of the unstable 4-methoxyl-azetidin-2-one intermediate leading to a non-lactam dipeptide 5 under the same reaction condition, which was similar to Kamata’s report (Scheme 2).17 To the best of our knowledge, this is the first report about the methanolysis of azetidinone fused oxazoline derivatives.
Inspired by this interesting finding, we further explored the effects of the substituents on the methanolysis of azetidinone fused oxazoline derivatives (Table 1). When R1=Ph and R2=H (Table 1, entry 1), the structure remained unchanged in methanol even under reflux. In comparison, when R1=Bn and R2=H (Table 1, entry 2), it caused the cleavage of the C-N bond affording the oxazole derivate 5a at room temperature. To our surprise, when R1=Ph and R2=Cl (Table 1, entry 3), an unexpected dipeptide 5 was efficiently obtained in good yield (75%). The detailed mechanism towards these different behaviors remains unclear.
Base-catalyzed intramolecular cascade sequence afforded the butenolide 6, which involved three cascade reactions: (a) substitution reaction of the chloride with hydroxide anion; (b) the intramolecular transesterification; (c) base promoted isomerization of double bond (Scheme 3).
In order to obtain good yield of compound 6, we examined the reaction under various conditions (solvent, base, temperature, and phase transfer catalyst). The results were summarized in Table 2. We found that solvents and bases played an important role in this reaction sequence, while TBAB was not necessary for this reaction. When the reaction was performed in MeCN/H2O (4/1) (Table 2, entry 1), compound 6 was obtained in 78% yield. When the amount of water used decreased, the yield was only 5% and 60% with prolonged time (>12 h), respectively (Table 2, entries 2, 4). When 1.0 eq of TBAB was added, the yield remained almost unchanged (Table 2, entry 3). When the mild base NaHCO3 was used, the yield was 42% (Table 2, entry 7). Compound 5 was completely decomposed due to the strong basicity of NaOH and no product was obtained (Table 2, entry 8). When DMF/H2O (4/1) as the solvent was used, the yield decreased to about 30% (Table 2, entry 5). Particularly, when MeOH/H2O (4/1) was used as the solvent (Table 2, entry 6), it caused the isomerization of double bond; no desired product 6 was observed. Thus, the best condition for this reaction was the use of compound 5 (1.0 eq), Na2CO3 (1.0 eq), MeCN/H2O (4/1) as the solvent under reflux (Table 2, entry 1).
Our group previously developed an efficient method for the construction of γ-AIBs, which was successfully applied to the stereoselective synthesis of andrographolide and digoxin derivatives containing γ-AIB moiety.18 Our strategy involved the investigation of the effects of bases (pyridine, 1,2-ethanediamine, DMAP, NaHCO3, Na2CO3 and K2CO3) and solvents (CHCl3, MeCN, THF and MeOH) in the aldol condensation of butenolide with aldehydes or ketones, revealing that Na2CO3 proved to be more effect in promoting the aldol condensation with most of aldehydes, but to ketones, the catalyst 1,2-ethanediamine was the most effective in methanol. Unfortunately, no desired products were obtained under this protocol when compound 6 was subjected to aromatic ketones and aliphatic aldehydes due to the low reactivity of them. Besides, we also found that treatment 6 with aromatic aldehydes with electron-withdrawing group did not afford the corresponding products, showing that the electronic effect of the substituents on the aromatic ring had a remarkable effect on this reaction.
Having established the optimal conditions, we then applied the protocol to the vinylogous aldol reaction of different aldehydes or ketones with 6, affording a series of novel γ-alkylidenebutenolides (7a-t) in 53%-86% yields (Table 3). Besides, different ratio of Z- or E-isomer was observed depending on the R1 and R2 groups which could be explained by an E1cb mechanism19 whereby the formation of the Z-isomer was favored due to the steric hindrance provided by the β-methyl (Scheme 4). The Z-isomers are generally more stable than their E-counterparts, especially when a β-substituent exists.20 That is why most naturally occurring γ-alkylidenebutenolides have the Z-configuration, although many of them lack a β-substituent, as represented by freelingyne and tetrenolin (Figure 1).
In summary, we have developed a simple method to synthesize a series of novel γ-alkylidenebutenolides from readily available starting material 6-APA. Besides, we first reported the methanolysis behaviors of azetidinone fused oxazoline derivatives under mild condition and the detailed mechanism is under investigation. Butenolide 6 was synthesized via the intramolecular cascade sequence in one-pot under mild condition. This strategy is valuable for the construction of compound libraries and the screening of lead compound. Efforts towards biological evaluation of these new compounds are in progress and will be reported elsewhere.
EXPERIMENTAL
All reagents and solvents used were of analytical grade purchased from commercial sources. Thin-layer chromatography (TLC) was carried out on glass plates coated with silica gel (Qingdao Haiyang Chemical Co., G60F-254) and visualized by UV light (254 nm). The products were purified by column chromatography over silica gel (Qingdao Haiyang Chemical Co., 200–300 mesh). Melting points were determined on a Beijing Keyi XT4A apparatus and are uncorrected. All NMR spectra were recorded with a Bruker DPX 400 MHz spectrometer with TMS as internal standard. Chemical shifts are given as δ ppm values relative to TMS. Mass spectra (MS) were recorded on Esquire3000 mass spectrometer by electrospray ionization (ESI).
Synthesis of compound 4 Compound 4 was prepared from 6-APA according to the literature procedures.15,16 Compound 4 was synthesized from 6-APA by acylation, oxidation and protection of carboxyl group in one pot to give penicillin sulfoxide 2, which was subjected to rearrangement and chlorination with an overall yield of about 56.7%. White solid; 1H NMR (400 MHz, CDCl3, TMS) δ 7.90 (d, J = 7.3 Hz, 2H), 7.59–7.27 (m, 13H), 6.92 (s, 1H,), 6.30 (d, J = 3.3 Hz, 1H), 5.47 (s, 1H), 5.37 (d, J = 3.3 Hz, 1H), 5.28 (s, 1H), 5.12 (s, 1H), 4.13–3.99 (dd, J = 12.0, 16.0 Hz, 2H); 13C NMR (101 MHz, CDCl3, TMS) δ 167.70, 166.80, 166.76, 138.94, 138.88, 138.26, 132.34, 128.62, 128.38, 127.26, 127.02, 126.84, 120.49, 86.25, 82.14, 79.09, 77.38, 77.06, 76.74, 55.09, 45.60.
Synthesis of dipeptide 5 Compound 4 (10 mmol) was placed in a flask and MeOH (20 mL) was added, the reaction mixture was stirred overnight at room temperature. The product was precipitated gradually from the solution, filtered and dried as a white solid; Yield: 75%; mp 136.1-136.6 ºC; 1H NMR (400 MHz, CDCl3, TMS) δ 7.87–7.80 (d, 2H), 7.62 (d, J = 7.5 Hz, 1H), 7.58–7.22 (m, 14H), 6.90 (s, 1H), 5.47 (s, 1H), 5.43 (s, 1H), 5.36 (d, J = 7.5 Hz, 1H), 4.80 (dd, J = 6.0, 2.2 Hz, 1H), 4.77 (d, J = 2.2 Hz, 1H), 4.14 (dd, J = 42.1, 12.4 Hz, 2H), 3.59 (s, 3H), 3.45 (s, 3H); 13C NMR (101 MHz, CDCl3, TMS) δ 168.64, 167.33, 167.22, 140.10, 139.27, 139.23, 133.56, 132.05, 128.73, 128.65, 128.38, 128.31, 127.26, 127.20, 127.15, 119.52, 104.87, 78.96, 57.78, 56.85, 55.74, 55.56, 44.94; HRMS (ESI): m/z calcd for C30H32ClN2O6 (M+H)+, 551.1949; found, 551.1945.
Synthesis of butenolide 6 To a solution of 5 (9 mmol) in MeCN/H2O (4/1, 20 mL) was added Na2CO3 (9 mmol), the resulting mixture was stirred under reflux for about 4 h. The reaction mixture was evaporated in vacuum when completed and then EtOAc and water were added, the aqueous layer was extracted with EtOAc, the combined organic layers were washed with water and brine, dried and concentrated successively. The residue was subjected to recrystallization from EtOH to give 6 as a white solid; Yield: 78%; mp 146.3-147.6 ºC; 1H NMR (400 MHz, CDCl3, TMS) δ 8.28 (s, 1H), 7.94–7.41 (m, 5H), 7.18 (d, J = 6.3 Hz, 1H), 4.93 (dd, J = 6.3, 2.7 Hz, 1H), 4.80 (d, J = 2.7 Hz, 1H), 4.73 (s, 2H), 3.63 (s, 3H), 3.50 (s, 3H), 2.18 (s, 3H); 13C NMR (101 MHz, CDCl3, TMS) δ 170.62, 167.41, 166.42, 145.69, 133.35, 132.10, 128.69, 127.24, 120.90, 104.34, 72.13, 57.57, 56.54, 55.97, 14.07; HRMS (ESI): m/z calcd for C17H20N2NaO6 (M+Na)+, 371.1219; found, 371.1216.
General procedure for preparation of compounds 7a-t To a solution of 6 (1 mmol) in MeOH (10 mL) was added Na2CO3 (1 mmol), the resulting mixture was stirred under reflux for about 3 h, MeOH was removed and water was added. The mixture was extracted with EtOAc. The combined organic layers were washed with water, brine, dried (Na2SO4), concentrated, and then purified by column chromatography on silica gel (Petroleum ether /EtOAc = 2/1).
3-Substituted amino-4-methyl-5-(propan-2-ylidene) furan-2(5H)-one (7a) White solid; Yield: 65%; mp 135.6-136.3 ºC; 1H NMR (400 MHz, CDCl3, TMS) δ 8.13 (s, 1H), 7.87 (d, J = 7.8 Hz, 2H), 7.45-7.55 (m, 3H), 7.20 (d, J = 6.3 Hz, 1H), 4.94 (dd, J = 6.3, 2.5 Hz, 1H), 4.81 (d, J = 2.5 Hz, 1H), 3.63 (s, 3H), 3.50 (s, 3H), 2.25 (s, 3H), 2.07 (s, 3H), 2.03 (s, 3H); 13C NMR (101 MHz, CDCl3, TMS) δ 167.43, 166.67, 165.70, 143.79, 140.63, 133.34, 132.10, 128.69, 127.25, 124.19, 122.86, 104.39, 57.44, 56.65, 56.07, 20.74, 18.91, 15.43. HRMS (ESI): m/z calcd for C20H25N2O6 (M+H)+, 389.1713; found, 389.1718.
3-Substituted amino-5-(butan-2-ylidene)-4-methylfuran-2(5H)-one (7b) White solid; Yield: 60%; mp 97.9-98.7 ºC; 1H NMR (400 MHz, CDCl3) δ 8.15 (s, 1H), 7.90–7.83 (m, 2H), 7.58–7.42 (m, 3H), 7.21 (d, J = 6.4 Hz, 1H), 4.94 (dd, J = 6.4, 2.7 Hz, 1H), 4.81 (d, J = 2.7 Hz, 1H), 3.62 (s, 3H), 3.49 (s, 3H), 2.42 (q, J = 7.5 Hz, 2H), 2.25 (s, 3H), 2.05 and 2.02 (two singlets, 3H), 1.16-1.06 (m, 3H); 13C NMR (101 MHz, CDCl3) δ 167.44, 166.71, 165.71, 143.53, 143.34, 140.83, 140.42, 133.35, 132.09, 130.17, 129.70, 128.68, 127.25, 104.39, 57.40, 56.61, 56.07, 29.71, 27.38, 25.33, 17.93, 16.54, 15.46, 14.89, 13.07, 12.28; HRMS (ESI): m/z calcd for C21H27N2O6 (M+H)+, 403.1869; found, 403.1868.
3-Substituted amino-5-cyclohexylidene-4-methylfuran-2(5H)-one (7c) White solid; Yield: 63%; mp 128.3-129.5 ºC; 1H NMR (400 MHz, CDCl3) δ 8.14 (s, 1H), 7.93–7.82 (m, 2H), 7.45-7.57 (m, 3H), 7.21 (d, J = 6.4 Hz, 1H), 4.94 (dd, J = 6.4, 2.7 Hz, 1H), 4.81 (d, J = 2.7 Hz, 1H), 3.63 (s, 3H), 3.49 (s, 3H), 2.62–2.46 (t, 4H), 2.25 (s, 3H), 1.66 (d, J = 6.3 Hz, 6H); 13C NMR (101 MHz, CDCl3) δ 167.44, 166.74, 165.73, 141.22, 140.77, 133.36, 133.00, 132.09, 128.69, 127.25, 123.46, 104.39, 57.39, 56.63, 56.08, 29.91, 28.07, 27.60, 26.15, 15.59; HRMS (ESI): m/z calcd for C23H29N2O6 (M+H)+, 429.2026; found, 429.2025.
3-Substituted amino-5-(furan-2-ylmethylene)-4-methylfuran-2(5H)-one (7d) Yellow solid; Yield: 75%; mp 82.1-82.8 ºC;1H NMR (400 MHz, CDCl3, TMS) δ 8.45 (s, 1H), 7.89-7.83 (m, 2H), 7.46-7.55 (m, 3H), 7.46 (s, 1H), 7.21 (d, J = 6.4 Hz, 1H), 6.99 (t, J = 3.2 Hz, 1H), 6.53 (d, J = 1.5 Hz, 1H), 6.17 (s, 1H), 4.96 (dd, J = 6.4, 2.5 Hz, 1H), 4.81 (d, J = 2.5 Hz, 1H), 3.65 (s, 3H), 3.51 (s, 3H), 2.24 (s, 3H); 13C NMR (101 MHz, CDCl3, TMS) δ 167.43 , 166.34 , 165.72 , 149.04 , 146.15 , 143.67 , 137.27 , 133.29 , 132.15 , 128.71 , 128.57 , 127.25 , 121.57 , 114.45 , 112.94 , 104.36 , 99.04 , 57.65 , 56.62 , 56.10 , 11.62; HRMS (ESI): m/z calcd for C22H22N2NaO7 (M+Na)+, 449.1325; found, 449.1326.
5-((1H-Pyrrol-2-yl)methylene)-3-substituted amino-4-methylfuran-2(5H)-one (7e) Yellow solid; Yield: 79%; mp 151.3-151.6 ºC; 1H NMR (400 MHz, DMSO-d6) δ 11.10 (s, 1H), 10.03 (s, 1H), 8.64 (t, J = 8.5 Hz, 1H), 7.91 (m, 2H), 7.53 (m, 3H), 7.01 (d, J = 1.2 Hz, 1H), 6.61 (s, 1H), 6.39 (s, 1H), 6.22 (dd, J = 5.6, 2.4 Hz, 1H), 5.02–4.90 (m, 1H), 4.78 (d, J = 7.2 Hz, 1H), 3.38 (s, 3H), 3.37 (s, 3H), 2.04 (s, 3H); 13C NMR (101 MHz, DMSO-d6) δ 168.52, 166.65, 165.75, 143.29, 142.91, 134.21, 132.00, 128.75, 128.06, 126.12, 123.48, 120.66, 114.83, 110.91, 103.20, 102.32, 55.46, 55.39, 53.83, 10.71; HRMS (ESI): m/z calcd for C22H24N3O6 (M+H)+, 426.1665; found, 426.1667.
3-Substituted amino-4-methyl-5-((5-methylfuran-2-yl)methylene)furan-2(5H)-one (7f) Yellow solid; Yield: 86%; mp 151.3-151.6 ºC; 1H NMR (400 MHz, CDCl3) δ 8.39 (s, 1H), 7.86 (m, J = 16.1, 7.6 Hz, 2H), 7.62–7.40 (m, 4H), 7.20 (d, J = 6.3 Hz, 1H), 6.89 (s, 1H), 6.14 (d, J = 2.6 Hz, 1H), 4.96 (dd, J = 6.3, 2.4 Hz, 1H), 4.81 (d, J = 2.4 Hz, 1H), 3.64 (s, 3H), 3.50 (s, 3H), 2.35 (s, 3H), 2.22 (s, 3H); 13C NMR (101 MHz, CDCl3) δ 167.45, 166.33, 165.87, 154.25, 147.59, 145.07, 137.70, 133.33, 132.12, 128.70, 127.25, 120.99, 116.13, 109.60, 104.37, 99.37, 57.59, 56.59, 56.10, 13.84, 11.52; HRMS (ESI): m/z calcd for C23H24N2NaO7 (M+Na)+, 463.1481; found, 463.1487.
3-Substituted amino-4-methyl-5-(3,4,5-trimethoxybenzylidene)furan-2(5H)-one (7g) Yellow solid; Yield: 81%; mp 153.5-155.8 ºC; 1H NMR (400 MHz, CDCl3, TMS) δ 8.45 (s, 1H), 7.93–7.87 (m, 2H), 7.60–7.41 (m, 3H), 7.21 (d, J = 6.4 Hz), 7.02 (s, 2H), 6.05 (s, 1H), 4.98 (dd, J = 6.4, 2.7 Hz, 1H), 4.82 (d, J = 2.7 Hz, 1H), 3.91 (s, 6H), 3.89 (s, 3H), 3.65 (s, 3H), 3.51 (s, 3H), 2.28 (s, 3H); 13C NMR (101 MHz, CDCl3, TMS) δ 167.46, 166.45, 166.04, 153.27, 147.38, 138.97, 138.53, 133.29, 132.17, 128.72, 128.64, 128.51, 127.25, 121.34, 110.15, 107.67, 104.34, 61.00, 57.66, 56.56, 56.21, 56.13, 11.83; HRMS (ESI): m/z calcd for C26H27N2O6 (M+H)+, 463.1869; found, 463.1866.
3-Substituted amino-4-methyl-5-((E)-3-phenylallylidene)furan-2(5H)-one (7h) Yellow solid; Yield: 70%; mp 124.6-124.8 ºC; 1H NMR (400 MHz, CDCl3, TMS) δ 8.47 (s, 1H), 7.86–7.93 (m, 2H), 7.41–7.60 (m, 5H), 7.26–7.42 (m, 4H), 7.20 (d, J = 6.4 Hz, 1H), 6.79 (d, J = 15.7 Hz, 1H), 6.12 (d, J = 11.3 Hz, 1H), 4.96 (dd, J = 6.4, 2.7 Hz, 1H), 4.81 (d, J = 2.7 Hz, 1H), 3.65 (s, 3H), 3.51 (s, 3H), 2.24 (s, 3H); 13C NMR (101 MHz, CDCl3, TMS) δ 167.38, 166.22, 165.51, 148.36, 148.36, 136.79, 136.50, 136.17, 133.23, 132.10, 128.78, 128.66, 127.19, 127.01, 121.97, 121.40, 111.40, 104.32, 104.32, 57.62, 56.61, 56.05, 11.59; HRMS (ESI): m/z calcd for C26H27N2O6 (M+H)+, 463.1869; found, 463.1866.
3-Substituted amino-5-(4-(dimethylamino)benzylidene)-4-methylfuran-2(5H)-one (7i) Yellow solid; Yield: 84%; mp 85.3-86.8 ºC; 1H NMR (400 MHz, CDCl3) δ 8.27 (s, 1H), 7.95–7.77 (m, 2H), 7.69 (d, J = 8.9 Hz, 1H), 7.57-7.45 (m, 4H), 7.23-7.20 (m, 2H), 6.68 (dd, J = 8.7, 6.0 Hz, 1H), 6.07 (s, 1H), 4.95 (dd, J = 6.4, 2.7 Hz, 1H), 4.82 (dd, J = 5.0, 2.7 Hz, 1H), 3.65 (s, 3H), 3.51 (s, 3H), 3.03 (s, 6H), 2.25 (s, 3H); 13C NMR (101 MHz, CDCl3) δ 167.44, 166.68, 166.32, 150.56, 144.82, 133.40, 132.13, 131.68, 128.69, 127.26, 121.03, 119.56, 111.98, 111.56, 104.41, 57.53, 56.62, 56.07, 40.17, 29.71, 11.65; HRMS (ESI): m/z calcd for C26H30N3O6 (M+H)+, 480.2135; found, 480.2132.
3-Substituted amino-5-(4-methoxybenzylidene)-4-methylfuran-2(5H)-one (7j) Yellow solid; Yield: 78%; mp 159.5-161.8 ºC; 1H NMR (400 MHz, CDCl3) δ 8.41 (s, 1H), 7.88 (d, J = 7.5 Hz, 2H), 7.72 (d, J = 8.6 Hz, 2H), 7.56-7.44 (m, 3H), 7.34–7.18 (m, 1H), 6.91 (d, J = 8.6 Hz, 2H), 6.07 (s, 1H), 4.98 (dd, J = 6.2, 2.3 Hz, 1H), 4.82 (d, J = 2.3 Hz, 1H), 3.84 (s, 3H), 3.63 (s, 3H), 3.50 (s, 3H), 2.25 (s, 3H); 13C NMR (101 MHz, CDCl3) δ 167.48, 166.46, 166.30, 160.14, 146.38, 139.18, 133.35, 132.12, 128.68, 127.27, 125.81, 120.82, 114.33, 110.15, 104.36, 57.51, 56.51, 56.08, 55.34, 11.72; HRMS (ESI): m/z calcd for C25H27N2O7 (M+H)+, 467.1818; found, 467.1819.
3-Substituted amino-5-(4-fluorobenzylidene)-4-methylfuran-2(5H)-one (7k) Yellow solid; Yield: 53%; mp 77.7-78.5 ºC; 1H NMR (400 MHz, CDCl3) δ 8.44 (s, 1H), 7.96–7.79 (m, 3H), 7.75 (dd, J = 8.8, 5.5 Hz, 2H), 7.57-7.45 (m, 4H), 7.24–7.13 (m, 1H), 7.08 (t, J = 8.7 Hz, 2H), 6.08 (s, 1H), 4.97 (dd, J = 6.5, 2.7 Hz, 1H), 4.82 (d, J = 2.7 Hz, 1H), 3.65 (s, 3H), 3.51 (s, 3H), 2.27 (s, 3H); 13C NMR (101 MHz, CDCl3) δ 167.48, 166.44, 166.05, 147.60, 138.32, 133.30, 132.32, 132.24, 132.15, 128.71, 127.25, 121.59, 108.86, 104.38, 57.62, 56.60, 56.15, 11.81; HRMS (ESI): m/z calcd for C24H23FN2NaO6 (M+Na)+, 477.1438; found, 477.1439.
3-Substituted amino-5-benzylidene-4-methylfuran-2(5H)-one (7l) Yellow solid; Yield: 65%; mp 141.8-142.6 ºC; 1H NMR (400 MHz, CDCl3) δ 8.44 (s, 1H), 7.97–7.80 (m, 3H), 7.76 (d, J = 7.4 Hz, 2H), 7.60–7.14 (m, 6H), 6.12 (s, 1H), 4.97 (dd, J = 6.5, 2.7 Hz, 1H), 4.82 (d, J = 2.8 Hz, 1H), 3.65 (s, 3H), 3.51 (s, 3H), 2.28 (s, 3H); 13C NMR (101 MHz, CDCl3) δ 169.79, 167.42, 166.27, 147.95, 138.35, 133.33, 132.96, 132.14, 131.84, 130.44, 128.80, 128.71, 128.57, 127.25, 121.65, 110.12, 104.38, 103.88, 57.62, 56.60, 56.15, 11.84; HRMS (ESI): m/z calcd for C24H25N2O6 (M+H)+, 437.1713; found, 437.1711.
3-Substituted amino-5-(4-isopropylbenzylidene)-4-methylfuran-2(5H)-one (7m) Yellow solid; Yield: 61%; mp 95.3-94.5 ºC; 1H NMR (400 MHz, CDCl3) δ 8.43 (s, 1H), 7.95–7.79 (m, 2H), 7.69 (d, J = 8.3 Hz, 2H), 7.63–7.41 (m, 4H), 7.31–7.18 (m, 2H), 6.11 (s, 1H), 4.98 (dd, J = 6.5, 2.8 Hz, 1H), 4.82 (d, J = 2.8 Hz, 1H), 3.64 (s, 3H), 3.50 (s, 3H), 2.92 (m, 1H), 2.27 (s, 3H), 1.26 (d, J = 6.9 Hz, 6H); 13C NMR (101 MHz, CDCl3) δ 150.04, 147.37, 138.72, 133.36, 132.11, 130.57, 128.69, 128.56, 127.25, 126.94, 121.32, 110.29, 104.36, 103.87, 57.54, 56.53, 56.12, 34.03, 23.77, 11.79; HRMS (ESI): m/z calcd for C27H30N2NaO6 (M+Na)+, 501.2002; found, 501.2007.
3-Substituted amino-5-(2-hydroxybenzylidene)-4-methylfuran-2(5H)-one (7n) Yellow solid; Yield: 78%; mp 110.4-111.5 ºC; 1H NMR (400 MHz, acetone-d6) δ 8.98 (s, 1H), 8.03 (dd, J = 7.8, 1.4 Hz, 1H), 8.00–7.90 (m, 2H), 7.76 (d, J = 7.9 Hz, 1H), 7.62–7.44 (m, 3H), 7.27–7.10 (m, 1H), 6.96-6.92 (m, 2H), 6.70 (s, 1H), 5.15–4.99 (m, 1H), 4.85 (d, J = 5.1 Hz, 1H), 3.50 (s, 3H), 3.49 (s, 3H), 2.22 (s, 3H); 13C NMR (101 MHz, acetone-d6) δ 168.40, 167.74, 166.56, 156.70, 148.46, 135.23, 132.66, 131.96, 131.15, 129.47, 128.49, 123.09, 121.58, 121.14, 116.54, 104.85, 104.21, 56.82, 56.63, 55.13, 11.63; HRMS (ESI): m/z calcd for C24H24N2NaO7 (M+Na)+, 475.1481; found, 475.1483.
3-Substituted amino-4-methyl-5-(4-morpholinobenzylidene)furan-2(5H)-one (7o) Yellow solid; Yield: 72%; mp 164.8-166.2 ºC; 1H NMR (400 MHz, CDCl3) δ 8.36 (s, 1H), 7.88 (d, J = 7.4 Hz, 2H), 7.70 (d, J = 8.8 Hz, 2H), 7.63–7.39 (m, 3H), 7.22 (d, J = 6.2 Hz, 1H), 6.88 (d, J = 8.8 Hz, 2H), 6.06 (s, 1H), 4.97 (dd, J = 6.4, 2.6 Hz, 1H), 4.82 (d, J = 2.6 Hz, 1H), 3.96 – 3.78 (m, 4H), 3.64 (s, 3H), 3.51 (s, 3H), 3.35–3.18 (m, 4H), 2.25 (s, 3H); 13C NMR (101 MHz, CDCl3) δ 167.45, 166.40, 151.18, 145.93, 139.36, 133.37, 132.10, 131.94, 128.69, 127.26, 124.18, 120.38, 114.74, 110.56, 104.38, 66.70, 48.09, 11.71; HRMS (ESI): m/z calcd for C28H32N3O7 (M+H)+, 522.2240; found, 522.2243.
3-Substituted amino-5-((1-benzyl-1H-indol-3-yl)methylene)-4-methylfuran-2(5H)-one (7p) Yellow solid; Yield: 74%; mp 154.3-155.7 ºC; 1H NMR (400 MHz, CDCl3) δ 8.27 (s, 1H), 8.03 (s, 1H), 7.89 (d, J = 7.2 Hz, 2H), 7.79 (dd, J = 6.4, 2.6 Hz, 1H), 7.57-7.53 (m, 1H), 7.49-7.46 (m, 2H), 7.39–7.19 (m, 8H), 7.14 (d, J = 6.8 Hz, 2H), 6.52 (s, 1H), 5.39 (s, 2H), 4.96 (dd, J = 6.4, 2.6 Hz, 1H), 4.83 (d, J = 2.6 Hz, 1H), 3.65 (s, 3H), 3.52 (s, 3H), 2.32 (s, 3H); 13C NMR (101 MHz, CDCl3) δ 167.44, 166.31, 145.17, 139.32, 136.55, 136.17, 133.41, 132.27, 132.09, 128.90, 128.69, 127.89, 127.61, 127.26, 126.69, 122.88, 120.94, 119.72, 118.46, 110.46, 109.33, 104.44, 102.77, 57.56, 56.64, 56.09, 50.74, 11.62; HRMS (ESI): m/z calcd for C33H31N3NaO6 (M+Na)+, 588.2111; found, 588.2110.
3-Substituted amino-5-((1-(4-chlorobenzyl)-1H-indol-3-yl)methylene)-4-methylfuran-2(5H)-one (7q) Yellow solid; Yield: 72%; mp 185.7-186.5 ºC; 1H NMR (400 MHz, CDCl3) δ 8.29 (s, 1H), 8.00 (d, J = 4.2 Hz, 1H), 7.88 (s, 2H), 7.80-7.76 (m, 1H), 7.67–7.38 (m, 3H), 7.24 (dd, J = 12.6, 4.4 Hz, 6H), 7.08-7.06 (m, 2H), 6.50 (s, 1H), 5.35 (s, 2H), 4.96 (s, 1H), 4.83 (s, 1H), 3.65 (s, 3H), 3.51 (s, 3H), 2.32 (s, 3H); 13C NMR (101 MHz, CDCl3) δ 167.46, 166.35, 166.28, 145.36, 139.60, 136.00, 135.08, 132.11, 129.10, 128.70, 128.02, 127.27, 123.02, 121.08, 120.33, 118.57, 110.31, 104.45, 102.55, 99.99, 57.56, 56.62, 56.10, 50.10, 11.62; HRMS (ESI): m/z calcd for C33H30ClN3NaO6 (M+Na)+, 622.1721; found, 622.1723.
3-Substituted amino-5-((1-(4-fluorobenzyl)-1H-indol-3-yl)methylene)-4-methylfuran-2(5H)-one (7r) Yellow solid; Yield: 68%; mp 201.2-202.7 ºC; 1H NMR (400 MHz, CDCl3) δ 8.31 (s, 1H), 8.03 (s, 1H), 7.92-7.89 (m, 2H), 7.82 (dd, J = 6.2, 2.4 Hz, 1H), 7.67-7.56 (m, 1H), 7.39–7.22 (m, 2H), 7.22–7.09 (m, 7H), 7.06-6.99 (m, 2H), 6.54 (s, 1H), 5.38 (s, 2H), 4.98 (dd, J = 7.3, 2.7 Hz, 1H), 4.86 (d, J = 2.7 Hz, 1H), 3.68 (s, 3H), 3.54 (s, 3H), 2.35 (s, 3H); 13C NMR (101 MHz, CDCl3) δ 167.45, 166.34, 166.28, 145.29, 139.31, 136.00, 133.40, 132.33, 132.10, 132.01, 128.70, 128.43, 128.35, 127.64, 127.26, 122.97, 121.03, 119.82, 118.54, 115.96, 115.74, 110.33, 109.48, 104.44, 102.61, 57.57, 56.63, 56.09, 50.05, 11.63; HRMS (ESI): m/z calcd for C33H30FN3NaO6 (M+Na)+, 606.2016; found, 606.2014.
3-Substituted amino-4-methyl-5-((1-(3,4,5-trimethoxybenzyl)-1H-indol-3-yl) methyl-ene) furan-2(5H)-one (7s) Yellow solid; Yield: 80%; mp 198.5-199.9 ºC; 1H NMR (400 MHz, CDCl3) δ 8.29 (s, 1H), 8.03 (s, 1H), 7.96–7.83 (m, 3H), 7.86–7.76 (m, 1H), 7.68–7.41 (m, 4H), 7.40–7.14 (m, 5H), 6.53 (s, 1H), 6.38 (s, 2H), 4.97 (dd, J = 6.3, 2.7 Hz, 1H), 4.84 (d, J = 2.7 Hz, 1H), 3.82 (s, 3H), 3.76 (s, 6H), 3.66 (s, 3H), 3.52 (s, 3H), 2.33 (s, 3H); 13C NMR (101 MHz, CDCl3) δ 167.46, 166.36, 166.31, 153.64, 145.21, 139.45, 136.25, 132.15, 128.70, 127.60, 127.24, 122.92, 120.95, 119.79, 119.50, 118.50, 110.43, 110.12, 109.38, 108.01, 104.45, 104.08, 103.90, 102.77, 60.85, 57.57, 56.63, 56.17, 56.14, 56.09, 50.96, 11.61; HRMS (ESI): m/z calcd for C36H37N3NaO9 (M+Na)+, 678.2427; found, 678.2430.
3-Substituted amino-5-((1-(benzo[d][1,3]dioxol-5-ylmethyl)-1H-indol-3-yl)methylene)-4-methylfuran-2(5H)-one (7t) Yellow solid; Yield: 82%; mp 123.8-124.5 ºC; 1H NMR (400 MHz, CDCl3) δ 8.28 (s, 1H), 7.99 (s, 1H), 7.88 (t, J = 6.8 Hz, 2H), 7.82-7.74 (m, 1H), 7.61–7.15 (m, 7H), 6.74 (d, J = 7.9 Hz, 1H), 6.67 (d, J = 8.0 Hz, 1H), 6.60 (d, J = 1.4 Hz, 1H), 6.51 (s, 1H), 5.91 (s, 2H), 5.27 (s, 2H), 4.96 (dd, J = 6.4, 2.7 Hz, 1H), 4.83 (d, J = 2.7 Hz, 1H), 3.65 (s, 3H), 3.52 (s, 3H), 2.32 (s, 3H); 13C NMR (101 MHz, CDCl3) δ 167.48, 166.36, 148.23, 147.37, 145.18, 139.42, 136.10, 133.44, 132.12, 130.38, 128.72, 127.68, 127.29, 122.91, 120.99, 120.28, 119.75, 118.51, 110.47, 109.36, 108.50, 107.34, 104.47, 102.80, 101.22, 57.57, 56.64, 56.11, 50.61, 50.61, 29.73, 11.64; HRMS (ESI): m/z calcd for C34H31N3NaO8 (M+Na)+, 632.2009; found, 632.2006.
ACKNOWLEDGEMENTS
This work was supported by the National Natural Sciences Foundations of China (No. 8112937, U1204206), China Postdoctoral Science Foundation (No. 20100480857, 201104402) and New Teachers’ Fund for Doctor Stations, Ministry of Education (No. 20114101120013).
References
1. F. von der Ohe and R. Bruckner, New J. Chem., 2000, 24, 659. CrossRef
2. F. Bellina, C. Anselmi, and R. Rossi, Tetrahedron Lett., 2002, 43, 2023. CrossRef
3. F. C. Görth, A. UmLand, and R. Brückner, Eur. J. Org. Chem., 1998, 1055. CrossRef
4. Z. Ahmed and P. Langer, J. Org. Chem., 2004, 69, 3753; CrossRef S. Rousset, M. Abarbri, J. Thibonnet, J. L. Parrain, and A. Duchêne, Tetrahedron Lett., 2003, 44, 7633. CrossRef
5. S. Ma, Z. Shi, and Z. Yu, Tetrahedron, 1999, 55, 12137; CrossRef T. K. M. Shing, H. C. Tsui, and Z. H. Zhou, J. Org. Chem., 1995, 60, 3121. CrossRef
6. S. Rousset, M. Abarbri, J. Thibonnet, A. Duchêne, and J. L. Parrain, Org. Lett., 1999, 1, 701. CrossRef
7. Y. S. Rao, Chem. Rev., 1964, 64, 353; CrossRef Y. S. Rao, Chem. Rev., 1976, 76, 625. CrossRef
8. E. i. Negishi and M. Kotora, Tetrahedron, 1997, 53, 6707. CrossRef
9. R. Bruckner, Curr. Org. Chem., 2001, 5, 679. CrossRef
10. P. Langer, Synlett, 2006, 3369. CrossRef
11. X. J. Wang, H. W. Xu, L. L. Guo, J. X. Zheng, B. Xu, X. Guo, C. X. Zheng, and H.-M. Liu, Bioorg. Med. Chem. Lett., 2011, 21, 3074. CrossRef
12. G. F. Dai, H. W. Xu, J. F. Wang, F. W. Liu, and H. M. Liu, Bioorg. Med. Chem. Lett., 2006, 16, 2710; CrossRef H. W. Xu, G. F. Dai, G. Z. Liu, J. F. Wang, and H. M. Liu, Bioorg. Med. Chem., 2007, 15, 4247. CrossRef
13. G. Z. Liu, H. W. Xu, G. W. Chen, P. Wang, Y. N. Wang, H. M. Liu, and D. Q. Yu, Bioorg. Med. Chem., 2010, 18, 1626. CrossRef
14. T. Staroske, L. Hennig, P. Welzel, H. J. Hofmann, D. Müller, T. Häusler, W. S. Sheldrick, S. Zillikens, B. Gretzer, H. Pusch, and H. G. Glitsch, Tetrahedron, 1996, 52, 12723. CrossRef
15. S. Yamamoto, S. Kamata, N. Haga, Y. Hamashima, and W. Nagata, Tetrahedron Lett., 1981, 22, 3089. CrossRef
16. T. Aoki, M. Yoshioka, Y. Sendo, and W. Nagata, Tetrahedron Lett., 1979, 20, 4327; CrossRef W. Nagata, Phil. Trans. R. Soc. Lond. B, 1980, 289, 225; CrossRef M. Yoshioka, T. Tsuji, S. Uyeo, S. Yamamoto, T. Aoki, Y. Nishitani, S. Mori, H. Satoh, Y. Hamada, H. Ishitobi, and W. Nagata, Tetrahedron Lett., 1980, 21, 351. CrossRef
17. S. Kamata, S. Yamamoto, N. Haga, and W. Nagata, J. Chem. Soc., Chem. Commun., 1979, 1106. CrossRef
18. H. W. Xu, J. F. Wang, G. Z. Liu, G. F. Hong, and H. M. Liu, Org. Biomol. Chem., 2007, 5, 1247. CrossRef
19. J. Boukouvalas, F. Maltais, and N. Lachance, Tetrahedron Lett., 1994, 35, 7897.
20. J. Boukouvalas, P. P. Beltrán, N. Lachance, S. Côté, F. Maltais, and M. Pouliot, Synlett, 2007, 219. CrossRef