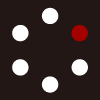
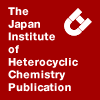
HETEROCYCLES
An International Journal for Reviews and Communications in Heterocyclic ChemistryWeb Edition ISSN: 1881-0942
Published online by The Japan Institute of Heterocyclic Chemistry
e-Journal
Full Text HTML
Received, 29th October, 2012, Accepted, 12th November, 2012, Published online, 16th November, 2012.
DOI: 10.3987/REV-12-757
■ Boron and Phosphorus Complexes of meso-Aryl Expanded Porphyrins
Tomohiro Higashino and Atsuhiro Osuka*
Department of Chemistry,, Graduate School of Science, Kyoto University, Sakyo, Kyoto 606-8502, Japan
Abstract
Our recent efforts in the boron and phosphorus insertion reactions into meso-aryl expanded porphyrins are reviewed. Boron insertion into [28]hexaphyrin(1.1.1.1.1.1) 19 triggered a skeletal rearrangement to bis-boron complex of [28]hexaphyrin(2.1.1.0.1.1) 20. Boron-insertion into quadruply N-fused heptaphyrin 23 with BBr3 and EtNiPr2 followed by treatment with an alcohol gave corresponding boron complex 24-OR. Treatment of non-fused heptaphyrin mono-Cu(II) complex 25 with BBr3 and EtNiPr2 induced a splitting reaction to B(III) subporphyrin 26 and Cu(II) porphyrin 27. Phosphorus insertion to N-fused [24]pentaphyrin 30 led to the formation of multiply fused P(V) complex 31, which was reduced to P(III) complex 33 with BH3·Me2S as a rare P(III) porphyrinoid. Phosphorus insertion to 19 proceeded in a stepwise manner to give mono-P(V) complex 38 and bis-P(V) complex 39, which were, respectively, identified to be Möbius aromatic and antiaromatic molecules. Phosphorus insertion into [34]heptaphyrin 40 gave Möbius antiaromatic P(V) complex 43 and Hückel aromatic P(V) complex 44. Upon heating in acetonitrile, 43 was cleanly converted to 44, indicating that 43 was a kinetically formed Möbius antiaromatic product as the first case. Phosphorus insertion into [36]octaphyrin 5 led to the formation of [38]octaphyrin mono-P(V) complex 47 and [40]octaphyrin bis-P(V) complex 48. The latter complex is a fully reduced octaphyrin, hence representing the first example of expanded isophlorins, but is stable under ambient conditions.CONTENTS
1. Introduction
2. Boron and Phosphorus Complexes of Porphyrins
2-1. Boron Complexes of Porphyrins
2-2. Phosphorus Complexes of Porphyrins
3. Boron Complexes of meso-Aryl Expanded Porphyrins
3-1. Boron Insertion Induced Skeletal Rearrangement to Hexaphyrin(2.1.1.0.1.1)
3-2. Boron Complexes of Heptaphyrin and Splitting Reaction
4. Phosphorus Complexes of meso-Aryl Expanded Porphyrins
4-1. N-Fused Pentaphyrin (NFP5)
4-1-1. General Properties
4-1-2. Phosphorus Complexes of Triply Fused [24]Pentaphyrin
4-2. Hexaphyrin
4-2-1. General Properties
4-2-2. Möbius Aromatic and Antiaromatic Phosphorus Complexes of Hexaphyrins
4-3. Heptaphyrin
4-3-1. General Properties
4-3-2. Hückel Aromatic and Möbius Antiaromatic Phosphorus Complexes of Heptaphyrins
4-4. Octaphyrin
4-4-1. General Properties
4-4-2. Phosphorus Complexes of Octaphyrin: Expanded Isophlorin
5. Conclusion
1. INTRODUCTION
In recent years, there is a growing interest in the chemistry of expanded porphyrins that consist of more than five pyrrolic units.1 Expanded porphyrins are homologues of porphyrins but possess attractive attributes such as multi-metal coordination capabilities,2 versatile redox-states,3 large nonlinear optical properties,4 transannular bridging reactions,5 metamorphosis-like mutual interconversions,6 and effective platforms to realize stable Möbius aromatic and antiaromatic species,7 which are not shared with porphyrins. We entered the chemistry of expanded porphyrins since our serendipitous finding of the effective synthesis of a series of meso-aryl substituted expanded porphyrins in the reaction of pentafluorobenzaldehyde and pyrrole.8 In 1987, Lindsey et al. reported a simple two-step one-flask procedure for the synthesis of porphyrins that was based on the condensation of pyrrole and aromatic aldehyde at 6.7 mM using BF3·OEt2 in CH2Cl2.9 We found that when the condensation reaction of pyrrole and pentafluorobenzaldehyde was conducted at 10 times higher concentrations (ca. 67 mM), a series of meso-aryl expanded porphyrins were produced with acceptable yields.8 This simple and effective synthesis is only applicable to 2,6-substituted electron-deficient aryl aldehydes. The size-selective synthesis of expanded porphyrins has been achieved by using dipyrromethane or tripyrrane as a building block.10,11 This size-selective protocol has advantages of better yields, easier separation, and availability of expanded porphyrins bearing two different meso-aryl substituents (Scheme 2).11
The systematic nomenclature of expanded porphyrins proposed by Franck and Nonn is employed in this account.12 In this nomenclature, the name of expanded porphyrin consists of three parts: 1) the number in the bracket indicates the number of π electrons in the macrocyclic conjugation pathway, 2) the core name that indicates the number of pyrrole subunits, and 3) the numbers in the parentheses indicate the numbers of bridging carbon atoms between the constitutional pyrrole groups.
The chemistry of main group porphyrin complexes has been actively studied. It is known that elements with the covalent radius (rcov) of 1.22–1.43 Å fit comfortably with a porphyrin cavity. Phosphorus and boron are considerably small (rcov = 1.10 and 0.85 Å, respectively), hence requiring structural distortion or doubly occupancy for their accommodation in porphyrin.13 While various metal complexes of expanded porphyrins have been prepared so far,1i,2 their main group complexes have still remained rather limited. As rare examples, boron complexes of [22]hexaphyrin(1.0.0.1.0.0) (amethyrin) 7 and [32]octaphyrin(1.0.0.0.1.0.0.0) 8 were reported as hydrolytically stable complexes by Sessler and co-workers in 2004 (Figure 1).14 In this account, we summarized our recent advances in the chemistry of boron and phosphorus complexes of expanded porphyrins.
2. BORON AND PHOSPHORUS COMPLEXES OF PORPHYRINS
2-1. Boron Complexes of Porphyrins
In 1970s, an attempt to prepare a boron complex of porphyrin was reported but the data were not sufficient for full characterization.15 The first fully characterized boron complex 9 was reported by Brothers and co-workers in 1994,16 which was accompanied by complex 10 in 1998.17 They prepared these complexes by the reactions of TPP-type porphyrins with BF3 or BCl3 in the presence of a trace amount of water. They also reported that the reduction of dichlorodiboranyl complex 11 with magnesium anthracenide provided isophlorin complex 12, which exhibited strong 20π antiaromaticity.18 In addition, Latos-Grażyński and co-workers prepared boron complex of N-fused porphyrin 13, which represented a rare example of NNN-type, tripyrrolylboron complexes.19
2-2. Phosphorus Complexes of Porphyrins
Phosphorus is small for accommodation at the porphyrin core and hexa-coordination of the phosphorus(V) ion was proposed to result in a ruffled structure.20 The first phosphorus complex was reported by Gouterman et al. in 1976.21 Brothers synthesized a phosphorus(V) porphyrin 14 bearing chloride anions as axial ligands, and she revealed that the chloro groups on phosphorus atom can be replaced by oxygen and nitrogen substituents.22 Akiba et al. reported the synthesis of phosphorus porphyrin complexes that contained a phosphorus-carbon bond by reactions with organoaluminum reagents.23 Phosphorus porphyrin complexes can be used as a structural motif to create oligomeric architectures. For example, the “wheel-and-axle”-type phosphorus(V) porphyrin arrays 15 were synthesized by Shimizu et al.24 A porphyrin-based molecular electronic/optical device can be also constructed by utilizing the axial-bonding capability of phosphorus(V) porphyrin.25
Besides, phosphorus insertion has been recently shown to be also effective in creation of novel macrocycles. The rearrangement of tetraazaporphyrin into triazacorrole 16 was first reported by Hanack,26 and Goldberg has widely used this method in the azacorrole chemistry.27 Latos-Grażyński reported phosphorus complexes of N-fused porphyrin 1728 and N-fused telluraporphyrin 18,29 which are 20π porphyrin, isophlorin analogues. It is notable that phosphorus atom was bound to NNN- or NNC-manner as the P=O moiety, differing from usual porphyrin complex. Another porphyrinoids containing phosphorus atom, phosphaporphyrins have been synthesized by Matano although they are not “phosphorus complexes”.30
As described above, many phosphorus complexes have been synthesized so far. However, the phosphorus atom exists as a phosphorus(V) state in all cases and no phosphorus(III) derivatives has been reported in the literature.
3. BORON COMPLEXES OF MESO-ARYL EXPANDED PORPHYRINS
3-1. Boron Insertion Induced Skeletal Rearrangement to Hexaphyrin(2.1.1.0.1.1)
Treatment of [28]hexaphyrin(1.1.1.1.1.1) 19 with BBr3 in the presence of EtNiPr2 and subsequent aqueous work up, followed by oxidation with MnO2, gave bis-boron complex 20 (Scheme 3).31 The structure of 20 was revealed by single crystal X-ray diffraction analysis to be a bis-boron complex of [28]hexaphyrin(2.1.1.0.1.1) that has a figure-of-eight conformation but has a directly connected bipyrrole unit and an s-trans 1,3-butadienyl linkage at the opposite position (Figure 4). Although the mechanism of skeletal rearrangement is still unclear, the transposition requires the formation of α-α and meso-meso bonds and the subsequent cleavage of two meso-α bonds. The axial ligands can be exchanged by a different alkoxy ligand upon treatment with an alcohol similarly to subporphyrins.
Redox interconversion between 20 and 21 was confirmed as shown in Scheme 4. Complex 21 has been characterized to be a [30]hexaphyrin(2.1.1.0.1.1) with boron atoms in a trigonal coordination environment. The 1H NMR spectrum of 20 exhibits signals of the peripheral β-protons at δ = 8.76, 6.56, 6.23, 5.94, and 5.46 ppm with a signal at δ = 4.09 for the axial hydroxy group. These 1H NMR data indicated weak 28π Hückel antiaromaticity. On the other hand, the 1H NMR spectrum of 21 shows six doublets at δ = 7.66, 7.62, 7.53, 7.24, 7.21, and 5.44 ppm without any signal for axial ligands in line with the assigned weakly aromatic 30π hexaphyrin structure. The reversible interconversion between 20 and 21 is interesting, in that switching between tetrahedral versus trigonal boron coordination occurs upon the change in the number of π electrons in the hexaphyrin(2.1.1.0.1.1) conjugation network. In addition, treatment of 20 with a large excess of MnO2 (300–400 equiv) at room temperature provided free-base [26]hexaphyrin(2.1.1.0.1.1) 22 (Scheme 3).
3-2. Boron Complexes of Heptaphyrin and Splitting Reaction
Attempts of boron insertion into the small cavity of quadruply N-fused heptaphyrin 23 failed with BF3·OEt2 but was effected upon refluxing a solution of 23 in CH2Cl2 in the presence of BBr3 and EtNiPr2 (Scheme 5).32 Subsequent aqueous work up provided hydroxyboron complex 24-OH, which was converted into alkoxyboron complex 24-OR by refluxing in the presence of alcohol. The structure of 24-OiPr has been revealed by single crystal X-ray diffraction analysis (Figure 5). The boron atom is bound to the three pyrroles similarly to subphthalocyanines33 and subporphyrins.34 This NNN-type, tripyrrorylboron-like coordination is quite rare.
Later, we found that N-fusion reaction of heptaphyrin 4 was suppressed by its premetalation with Zn(II) or Cu(II) ion at the hemiporphyrin-like tetrapyrrolic segment. Treatment of Zn(II) complex with BBr3 or BCl3 under various conditions gave a complicated mixture containing free-base 4, a B(III)-Zn(II) dinuclear complex, and subporphyrin 26. However, the yield of 26 is quite low (<1%). On the other hand, treatment of Cu(II) complex 25a with BBr3 in the presence of EtNiPr2 at room temperature provided subporphyrin 26a and Cu(II) porphyrin 27a in 36 and 13% yield, respectively (Scheme 6).35 This splitting reaction is synthetically useful because the subporphyrin 26a cannot be obtained by the usual subporphyrin synthesis. Similar extrusion reaction of meso-trifluoromethyl heptaphyrin 25b provided subporphyrin 26b.36
4. PHOSPHORUS COMPLEXES OF MESO-ARYL EXPANDED PORPHYRINS
4-1. N-Fused Pentaphyrin (NFP5)
4-1-1. General Properties
Generally, meso-aryl-substituted pentaphyrin(1.1.1.1.1) 28 was obtained by acid-catalyzed condensation as only N-fused forms 2 in contrast to β-alkyl pentaphyrin reported by Gossaur.37 This N-fused structure was formed via inversion of pyrrole ring followed by oxidation, because of severe steric repulsion (Scheme 7).38 As a rare case, mono-meso-free pentaphyrin 29 was reported quite recently.39 Non-fused 29 possesses an inward-pointing meso-carbon bridge, preventing the inversion of pyrrole rings (Figure 6).
Pentaphyrin has two stable redox states with 22π- and 24π-electronic systems, [22]NFP5 2 and [24]NFP5 30, respectively (Figure 6). Pentaphyrin 2 can easily be reduced to 30 with NaBH4, and 30 is quantitatively oxidized back to 2 by treatment with DDQ. X-Ray crystal structures reveal that both 2 and 30 have a fused tricyclic structure, a dipyrromethene moiety, and an outward-pointing pyrrole (Figure 7). Except for the inverted pyrrole that is tilted significantly, the entire structure of 2 is almost planar so that a strong diatropic ring current effect arising from the 22π aromaticity is observed in the 1H NMR spectrum. On the other hand, 30 exhibits weak antiaromatic character due to its 24π-electron state.
4-1-2. Phosphorus Complexes of Triply Fused [24]Pentaphyrin
The reaction of 30 and POCl3 in the presence of triethylamine provided the P=O complex 31 in 66% yield (Scheme 8).40 The crystal structure of 31 revealed the inserted phosphorus atom bound to two nitrogen atoms and one carbon atom (Figure 8a). At the same time, N-fusion reaction between nitrogen atom N(5) and pentafluorophenyl substituent at C(55), and C–C bond formation between C(13) and C(23) occurred to form a triply fused structure. The 1H NMR spectrum of 31 displays five peaks due to the β-protons in the range of 6.35–4.34 ppm, indicating moderate antiaromaticity derived from 24π-electron state. The antiaromaticity likely originates from its highly rigid planar conformation forced by the phosphorus incorporation and triply fused structure.
The P=O moiety of 31 can be converted to different phosphorus states (Scheme 8). Complex 31 was converted to P=S complex 32 by the reaction with Lawesson’s reagent in 84% yield. Complex 32 has also a highly rigid planar structure and exhibits distinct antiaromaticity. In addition, complex 31 was reduced with BH3·SMe2 to provide phosphine-borane (P–BH3) complex 33 in 54% yield. X-Ray diffraction analysis of 33 revealed a C(7)–C(8) single bond with bond length of 1.544(5) Å, which was distinctly longer than those of C(2)–C(3) and C(17)–C(18) double bonds; 1.390(5) and 1.375(5) Å, respectively (Figure 8c). The 1H NMR spectrum of 33 displays four peaks due to the β-protons of the reduced pyrrole B at 2.65, 2.53, 2.37, and 2.18 ppm, and four peaks due to the β-protons of the pyrroles A and D at 7.22, 6.96, 6.33, and 5.57 ppm. These data indicate nonaromatic character derived from the interruption of macrocyclic conjugation at reduced pyrrole B.
Using these three phosphorus complexes, the realization of free phosphorus(III) species was attempted. However, neither the reduction of 31 with silane reducing reagents nor desulfurization of 32 with electron-rich phosphines or Raney Nickel gave the desired phosphorus(III) compounds. On the other hand, the attempt at removal of borane from 33 provided the chlorin-type P=O complex 34, no free phosphorus(III) compounds was obtained (Scheme 8). Therefore, it is conceivable that a free P(III) species is quite unstable toward oxygenation. In this regard, P–BH3 complex 33 can be regarded as a phosphorus(III) complex, which is rare in the chemistry of phosphorus porphyrinoid.
4-2. Hexaphyrin
4-2-1. General Properties
[26]Hexaphyrin(1.1.1.1.1.1) 3, which was first synthesized by Cavaleiro and co-workers,41 is one of the most studied expanded porphyrins. Hexaphyrin 3 was obtained as a major product in 16–20% yield by modified Rothemund-Lindsey protocol as an oxidized form with 26π-electron system.8 [26]Hexaphyrin 3 is easily reduced to [28]hexaphyrin 19 upon treatment with NaBH4, and 19 can be oxidized back to 3 (Scheme 9). Depending on the substituents, [26]hexaphyrins(1.1.1.1.1.1) take various conformations such as rectangle (3),11,41 dumbbell (35),42 figure-of-eight (36),43 and triangle (37-MSA)44 (Figure 9). Most of meso-aryl [26]hexaphyrins adopt a planar rectangular conformation with two inverted pyrrole rings, which allow its strong 26π aromaticity. On the other hand, [28]hexaphyrin exists as an equilibrium of rapid interconversion between twisted Möbius aromatic conformations and rectangular antiaromatic conformation in solution at ambient temperature (Figure 10).45 These rapid interconversions were frozen at low temperature (–100 °C), so that the 1H NMR spectrum exhibits distinct aromaticity because of the predominant contribution of a single Möbius conformation.
4-2-2. Möbius Aromatic and Antiaromatic Phosphorus Complexes of Hexaphyrin
The reaction of [28]hexaphyrin 19 with POCl3 gave mono-phosphorus complex 38 in 65% yield (Scheme 10).46 The twisted Möbius structure of 38 was revealed by X-ray diffraction analysis, in which the phosphoryl moiety was bound to the two nitrogen atoms of the pyrrole units A and B, and one carbon atom of the pyrrole unit C (Figure 11a). The 31P NMR spectrum of 38 shows a signal at –11.99 ppm, which is consistent with a P=O moiety. The 1H NMR spectrum of 38 displays ten signals of outer β-protons in the range δ = 7.75–6.53 ppm and a doublet signal of inner β-proton of the pyrrole unit C at δ = 2.99 ppm. The difference between the chemical shifts (Δδ) of the most shielded and deshielded β-protons is 4.76 ppm, indicating a diatropic ring current despite the large dihedral angle (65°) along its π conjugation.
Further treatment of 38 with PCl3 provided bis-phosphorus complex 39 in 46% yield (Scheme 10). The structure of bis-phosphorus complex 39 was also determined by X-ray diffraction analysis to be a twisted Möbius conformation (Figure 11b). The additional P=O moiety was bound to the three nitrogen atoms of the pyrrole units D, E, and F. The 31P NMR spectrum of 39 shows two signals at –5.67 and –7.70 ppm. Complex 39 was assigned as a reduced [30]hexaphyrin by high-resolution ESI-TOF-MS and charge consideration. This highly reduced electron state is probably stabilized by the two electron-withdrawing P=O moieties. The 1H NMR spectrum of 39 exhibits a remarkably deshielded signal at δ = 11.14 ppm that corresponds to the inner β-protons of pyrrole unit C, and ten signals in the range δ = 6.72–5.54 ppm that correspond to the outer β-protons. These data indicate a paratropic ring current.
The UV/vis absorption spectrum of 38 exhibits an intense Soret-like band at 588 nm and broad Q-like bands at 876 and 1055 nm. Moreover, 38 exhibits a fluorescence band at 1090 nm as a mirror image of the corresponding absorption spectrum (Figure 12). In contrast, the UV/vis absorption spectrum of 39 shows ill-defined Soret-like bands and broad, weak near-IR bands. Furthermore, 39 shows no fluorescence emission. These absorption and emission behaviors of 39 are characteristic of antiaromatic expanded porphyrins. NICS values at the gravity center of 38 and 39 were calculated to be –8.25 and +3.65 ppm, respectively in line with the assignments of Möbius aromatic and antiaromatic characters. It is worthy to note that 39 is the first structurally well-defined Möbius antiaromatic molecule.
4-3. Heptaphyrin
4-3-1. General Properties
meso-Aryl heptaphyrins(1.1.1.1.1.1.1) are obtained by acid-catalyzed condensation with usually 32π electron state as the most stable form. meso-Pentafluorophenyl [32]heptaphyrin 4 takes a figure-of-eight Hückel antiaromatic conformation in nonpolar solvents such as hexane, toluene, and CH2Cl2 (Figure 13a).47 The conformation of 4 depends on the polarity of the solvent, 4 takes a twisted Möbius aromatic conformation in polar solvents such as acetone (Scheme 11). In contrast to 4, meso-(2,6-dichlorophenyl) [32]heptaphyrin 40 takes a twisted Möbius conformation even in nonpolar solvents such as hexane. Its X-ray crystal structure shows a singly twisted Möbius structure with a smoothly conjugated pathway, which is consistent with its aromaticity confirmed by 1H NMR and UV/vis absorption (Figure 13b).
meso-Pentafluorophenyl [32]heptaphyrin 4 underwent N-fusion reaction quantitatively to form singly N-fused heptaphyrin 41 just on standing in solution.32 Further N-fusion reactions were induced by heating of a toluene solution of 41 at reflux, and then heating of a DMF solution with NaH provided quadruply N-fused heptaphyrin 23 as a rare 34π-electron species (Scheme 12). In addition, the figure-of-eight structure of 23 is rigid enough to separate two enantiomers.
4-3-2. Hückel Aromatic and Möbius Antiaromatic Phosphorus Complexes of Heptaphyrin
Treatment of meso-pentafluorophenyl [32]heptaphyrin 4 with PCl3 gave phosphorus complex 42 in 46% yield (Scheme 13).48 The crystal structure of 42 revealed a figure-of-eight conformation with penta-coordinated phosphorus atom bound to the four nitrogen atoms of the pyrrole rings A, B, C and D, and one ortho-carbon atom of a pentafluorophenyl group in a trigonal bipyramidal manner (Figure 14a). The 31P NMR spectrum of 42 shows a signal at –82.41 ppm, which is consistent with the penta-coordination geometry.49 Complex 42 is regarded as a weakly 34π aromatic molecule from its 1H NMR and UV/vis absorption spectra.
On the other hand, treatment of meso-(2,6-dichlorophenyl) [32]heptaphyrin 40 with PCl3 provided two phosphorus complexes 43 and 44 in 49 and 35% yield, respectively (Scheme 13). The ratio of two complexes is dependent on reaction temperature. While complex 43 is a major product at low temperature (43/44 = 1.9/1 at 0 °C), complex 44 is a major product at high temperature (43/44 = 1/4.1 at 60 °C). These results indicated that 43 is a kinetic product. This has been more clearly shown that heating of 43 in acetonitrile led to quantitative conversion to 44.
These two phosphorus complexes 43 and 44 are also assigned as [34]heptaphyrin by high resolution ESI-TOF-MS. The crystal structure of 43 revealed a twisted Möbius structure, in which the phosphorus atom was bound to the three nitrogen atoms of the pyrrole rings A, B and C as a P=O moiety (Figure 14b). The UV/vis absorption spectrum of 43 has an ill-defined Soret-like band, and weak and broad bands in near-IR region, which are characteristic of antiaromatic expanded porphyrins. Moreover, the 1H NMR spectrum of 43 displays signals due to the inner pyrrolic β-protons in the pyrrole rings A and D at 7.96 and 7.60 ppm, which are distinctly deshielded from the other outer β-protons that appear in the range of 6.51–5.51 ppm, indicating a weak paratropic ring current. From these spectroscopic studies, complex 43 is characterized as a Möbius antiaromatic molecule.
The other complex 44 was determined to take a figure-of-eight Hückel structure on the basis of X-ray diffraction analysis (Figure 14c). The P=O moiety was bound to the two nitrogen atoms of the pyrrole rings B and C, and one β-carbon atom of the pyrrole ring D. The complex 44 was assigned as a 34π Hückel aromatic molecule. The 1H NMR spectrum is very broad at room temperature, probably due to conformational flexibility, but became sharpened at –60 °C, exhibiting a doublet at 1.98 ppm due to the inner β-proton in the pyrrole D and twelve signals due to other β-protons in a range of 6.91–3.80 ppm. The difference of chemical shifts (Δδ) is relatively large (4.93 ppm), indicating the presence of a diatropic ring current.
4-4. Octaphyrin
4-4-1. General Properties
Since meso-aryl octaphyrin(1.1.1.1.1.1.1.1) has a large conjugation network, it possesses three different redox states with 34π-, 36π-, and 38π-electrons (Scheme 14). In the crystal structure, [36]octaphyrin 5 that is the most stable form, adopts a figure-of-eight conformation, consisting of two porphyrin-like tetrapyrrolic “hemi-macrocycles”.8 However, the 1H NMR spectrum of 5 indicates its nonaromatic nature. [36]Octaphyrin 5 is easily converted to [34]octaphyrin 45 by the oxidation with DDQ and to [38]octaphyrin 46 by the reduction with NaBH4 quantitatively. The structure of 45 revealed to be a figure-of-eight conformation with two inverted pyrrole rings (Figure 15). Contrary to 5, the 1H NMR spectrum of 45 displays a diatropic ring current effect, indicating a distinct 34π aromaticity. While [38]octaphyrin 46 also exhibits a 38π-aromatic character, the structure of 46 has not been determined yet.
4-4-2. Phosphorus Complexes of Octaphyrin: Expanded Isophlorin
The reaction of [36]octaphyrin 5 with PCl3 in the presence of triethylamine gave mono-phosphorus complex 47 and bis-phosphorus complex 48 in 43 and 9% yield, respectively (Scheme 15).50 In the presence of an additional small amount of water and by heating at 50 °C, bis-phosphorus complex 48 was obtained as a major product in 35% yield along with 47.
The structure of 47 was revealed by X-ray diffraction analysis to be a figure-of-eight structure with penta-coordinated phosphorus atom, which was bound to the three nitrogen atoms of the pyrroles F, G, H and two β-carbon atoms of the pyrroles A and E in a trigonal bipyramidal manner (Figure 16a). The 31P NMR spectrum shows a signal at –85.59 ppm, which is consistent with penta-coordination.49 The UV/vis absorption spectrum shows a Q-like band derived from 38π aromaticity at 1620 nm. Complex 47 can be reduced with NaBH4 to corresponding 40π-electron species 49, which is smoothly oxidized to 47 (Scheme 16). However, [40]octaphyrin 49 is unstable and readily oxidized back to 47, even when it is stored in the solid-state because of its highly reduced electron state.
The crystal structure of bis-phosphorus complex 48 was also a figure-of-eight structure, in which the additional P=O moiety was bound to the two nitrogen atoms of the pyrroles A, B and one β-carbon atom of the pyrrole C. The 31P NMR spectrum shows a new signal due to the additional P=O moiety at –9.80 ppm. From 1H NMR, MS and crystal structure, 48 is characterized as a [40]octaphyrin bis-phosphorus complex. The oxygen source of the P=O moiety of 48 is a small amount of water, which has been confirmed by synthesis of 18O-labelled 48 using H218O. Similar to the redox behaviors of 47 and 49, the oxidation of 48 gave aromatic [38]octaphyrin complex 50, and it is reduced back to 48 with NaBH4. In contrast to 49, bis-phosphorus complex 48 is stable under ambient condition and can be stored over several months without any oxidation in the solid state. The increase of stability is derived from electron-withdrawing effect of the P=O moiety. Importantly, this bis-phosphorus complex 48 is the first stable [40]octaphyrin, in other words, expanded isophlorin.
5. CONCLUSION
The boron insertion into meso-aryl expanded porphyrins triggered novel skeletal rearrangements such as the methine carbon transposition from [28]hexaphyrin(1.1.1.1.1.1) to [28]hexaphyrin(2.1.1.0.1.1) and the splitting reaction of a putative B(III)-Cu(II) complex of [34]heptaphyrin into subporphyrin and Cu(II) porphyrin. Similarly, the phosphorus insertion into N-fused pentaphyrin induced the multiple more fusion reactions. Interestingly, the phosphorus complexes can take fully reduced electron states, such as [24]pentaphyrin, [30]hexaphyrin, [34]heptaphyrin and [40]octaphyrin as “expanded isophlorins”, since the incorporated phosphorus atoms exist as strongly electron-withdrawing phosphorus(V) states, hence stabilizing the reduced states by their electron-withdrawing effects. Most importantly, the phosphorus insertions into [30]hexaphyrin and [34]heptaphyrin allowed the formation of the stable Möbius antiaromatic molecules as the first examples of structurally well-defined species.
ACKNOWLEDGEMENTS
This work was supported by Grants-in-Aid (No. 22245006(A) and 20108001 “pi-space”) for Scientific Research from MEXT. T. H. acknowledges a JSPS Fellowship for Young Scientists.
References
1. a) 'The Porphyrin Handbook,' Vol. 1–10, ed. by K. M. Kadish, K. M. Smith, and R. Guilard, Academic Press, San Diego, 2000; b) A. Jasat and D. Dolphin, Chem. Rev., 1997, 97, 2267; CrossRef c) T. D. Lash, Angew. Chem. Int. Ed., 2000, 39, 1763; CrossRef d) H. Furuta, H. Maeda, and A. Osuka, Chem. Commun., 2002, 1795; CrossRef e) J. L. Sessler and D. Seidel, Angew. Chem. Int. Ed., 2003, 42, 5134; CrossRef f) K. Chandrashekar and S. Venkatraman, Acc. Chem. Res., 2003, 36, 676; CrossRef g) A. Ghosh, Angew. Chem. Int. Ed., 2004, 43, 1918; CrossRef h) M. Stępień, N. Sprutta, and L. Latos-Grażyński, Angew. Chem. Int. Ed., 2011, 50, 4288; CrossRef i) S. Saito and A. Osuka, Angew. Chem. Int. Ed., 2011, 50, 4342; CrossRef j) A. Osuka and S. Saito, Chem. Commun., 2011, 47, 4330. CrossRef
2. a) S. Shimizu and A. Osuka, Eur. J. Inorg. Chem., 2006, 1319; CrossRef b) J. L. Sessler and E. Tomat, Acc. Chem. Res., 2007, 40, 371. CrossRef
3. a) S. Mori, J.-Y. Shin, S. Shimizu, F. Ishikawa, H. Furuta, and A. Osuka, Chem. Eur. J., 2005, 11, 2417; CrossRef b) S. Mori and A. Osuka, J. Am. Chem. Soc., 2005, 127, 8030. CrossRef
4. a) T. K. Ahn, J. H. Kwon, D. Y. Kim, D. W. Cho, D. H. Jeong, S. K. Kim, M. Suzuki, S. Shimizu, A. Osuka, and D. Kim, J. Am. Chem. Soc., 2005, 127, 12856; CrossRef b) Z. S. Yoon, J. H. Kwon, M.-C. Yoon, M. K. Koh, S. B. Noh, J. L. Sessler, J. T. Lee, D. Seidel, A. Aguilar, S. Shimizu, M. Suzuki, A. Osuka, and D. Kim, J. Am. Chem. Soc., 2006, 128, 14128; CrossRef c) J. M. Lim, Z. S. Yoon, J.-Y. Shin, K. S. Kim, M.-C. Yoon, and D. Kim, Chem. Commun., 2009, 261. CrossRef
5. a) M. Suzuki and A. Osuka, J. Am. Chem. Soc., 2007, 129, 464; CrossRef b) M. Suzuki and A. Osuka, Angew. Chem. Int. Ed., 2007, 46, 5171. CrossRef
6. a) Y. Tanaka, W. Hoshino, S. Shimizu, K. Youfu, N. Aratani, N. Maruyama, S. Fujita, and A. Osuka, J. Am. Chem. Soc., 2004, 126, 3046; CrossRef b) Y. Tanaka, H. Shinokubo, Y. Yoshimura, and A. Osuka, Chem. Eur. J., 2009, 15, 5674; CrossRef c) Y. Tanaka, H. Mori, T. Koide, H. Yorimitsu, N. Aratani, and A. Osuka, Angew. Chem. Int. Ed., 2011, 50, 11460; CrossRef d) H. Mori, N. Aratani, and A. Osuka, Chem. Asian J., 2012, 7, 1340. CrossRef
7. a) M. Stępień, L. Latos-Grażyński, N. Sprutta, P. Chawalisz, and L. Szterenberg, Angew. Chem. Int. Ed., 2007, 46, 7869; CrossRef b) Y. Tanaka, S. Saito, S. Mori, N. Aratani, H. Shinokubo, N. Shibata, Y. Higuchi, Z. S. Yoon, K. S. Kim, S. B. Noh, J. K. Park, D. Kim, and A. Osuka, Angew. Chem. Int. Ed., 2008, 47, 681; CrossRef c) J. K. Park, Z. S. Yoon, M.-C. Yoon, K. S. Kim, S. Mori, J.-Y. Shin, A. Osuka, and D. Kim, J. Am. Chem. Soc., 2008, 130, 1824; CrossRef d) M. Inoue, K. S. Kim, M. Suzuki, J. M. Lim, J.-Y. Shin, D. Kim, and A. Osuka, Angew. Chem. Int. Ed., 2009, 48, 6687; CrossRef e) J. M. Lim, J.-Y. Shin, Y. Tanaka, S. Saito, A. Osuka, and D. Kim, J. Am. Chem. Soc., 2010, 132, 3105; CrossRef f) M. Inoue, T. Yoneda, K. Youfu, N. Aratani, and A. Osuka, Chem. Eur. J., 2011, 17, 9028. CrossRef
8. J.-Y. Shin, H. Furuta, K. Yoza, S. Igarashi, and A. Osuka, J. Am. Chem. Soc., 2001, 123, 7190. CrossRef
9. a) J. S. Lindsey, I. C. Schreiman, H. C. Hsu, P. C. Kearney, and A. M. Marguerettaz, J. Org. Chem., 1987, 52, 827; CrossRef b) R. W. Wagner, D. S. Lawrence, and J. S. Lindsey, Tetrahedron Lett., 1987, 28, 3069. CrossRef
10. a) R. Taniguchi, S. Shimizu, M. Suzuki, J.-Y. Shin, H. Furuta, and A. Osuka, Tetrahedron Lett., 2003, 44, 2505; CrossRef b) Y. Kamimura, S. Shimizu, and A. Osuka, Chem. Eur. J., 2007, 13, 1620; CrossRef c) Y. Tanaka, J.-Y. Shin, and A. Osuka, Eur. J. Org. Chem., 2008, 1341. CrossRef
11. M. Suzuki and A. Osuka, Org. Lett., 2003, 5, 3943. CrossRef
12. B. Frank and A. Nonn, Angew. Chem. Int. Ed. Engl., 1995, 34, 1795. CrossRef
13. a) P. J. Brothers, J. Porphyrins Phthalocyanines, 2002, 6, 259; CrossRef b) P. J. Brothers, Chem. Commun., 2008, 2090. CrossRef
14. T. Köhler, M. C. Hodgson, D. Seidel, J. M. Veauthier, S. Meyer, V. Lynch, P. D. W. Boyd, P. J. Brothers, and J. L. Sessler, Chem. Commun., 2004, 1060. CrossRef
15. C. J. Carrano and M. Tsutsui, J. Coord. Chem., 1977, 7, 125. CrossRef
16. W. J. Belcher, P. D. W. Boyd, P. J. Brothers, M. J. Liddell, and C. E. F. Rickard, J. Am. Chem. Soc., 1994, 116, 8416. CrossRef
17. W. J. Belcher, M. Breede, P. J. Brothers, and C. E. F. Rickard, Angew. Chem. Int. Ed., 1998, 37, 1112. CrossRef
18. a) A. Weiss, H. Pritzkow, P. J. Brothers, and W. Siebert, Angew. Chem. Int. Ed., 2001, 40, 4182; CrossRef b) A. Weiss, M. C. Hodgson, P. D. W. Boyd, W. Siebert, and P. J. Brothers, Chem. Eur. J., 2007, 13, 5982. CrossRef
19. A. Młodzianowska, L. Latos-Grażyński, L. Szterenberg, and M. Stȩpień, Inorg. Chem., 2007, 46, 6950. CrossRef
20. J. K. Sanders, N. Bampos, Z. Clyde-Watson, S. L. Darling, J. C. Hawley, H.-J. Kim, C. C. Mak, and S. J. Webb, 'The Porphyrin Handbook: Axial Coordination Chemistry of Metalloporphyrins,' Vol. 3, ed. by K. M. Kadish, K. M. Smith, and R. Guilard, Academic Press, San Diego, 2000, pp. 1–48.
21. P. Sayer, M. Gouterman, and C. R. Connell, J. Am. Chem. Soc., 1977, 99, 1082. CrossRef
22. T. Barbour, W. J. Belcher, P. J. Brothers, C. E. F. Rickard, and D. C. Ware, Inorg. Chem., 1992, 31, 746. CrossRef
23. Y. Yamamoto, R. Nadano, M. Itagaki, and K. Akiba, J. Am. Chem. Soc., 1995, 117, 8287. CrossRef
24. H. Segawa, K. Kunimoto, K. Susumu, M. Taniguchi, and T. Shimizu, J. Am. Chem. Soc., 1994, 116, 11193. CrossRef
25. D. R. Reddy and B. G. Maiya, Chem. Commun., 2001, 117. CrossRef
26. J. Li, L. R. Subramanian, and M. Hanack, Eur. J. Org. Chem., 1998, 2759. CrossRef
27. B. Ramdhanie, C. L. Stern, and D. P. Goldberg, J. Am. Chem. Soc., 2001, 123, 9447. CrossRef
28. A. Młodzianowska, L. Latos-Grażyński, and L. Szterenberg, Inorg. Chem., 2008, 47, 6364. CrossRef
29. E. Pacholska-Dudziak, F. Ulatowski, Z. Ciunik, and L. Latos-Grażyński, Chem. Eur. J., 2009, 15, 10924. CrossRef
30. Y. Matano and H. Imahori, Acc. Chem. Res., 2009, 42, 1193. CrossRef
31. K. Moriya, S. Saito, and A. Osuka, Angew. Chem. Int. Ed., 2010, 49, 4297. CrossRef
32. S. Saito and A. Osuka, Chem. Eur. J., 2006, 12, 9095. CrossRef
33. C. G. Claessens, D. González-Rodríguez, and T. Torres, Chem. Rev., 2002, 102, 835. CrossRef
34. a) Y. Inokuma and A. Osuka, Dalton. Trans., 2008, 2517; CrossRef b) A. Osuka, E. Tsurumaki, and T. Tanaka, Bull. Chem. Soc. Jpn., 2011, 84, 679. CrossRef
35. S. Saito, K. S. Kim, Z. S. Yoon, G. Kim, and A. Osuka, Angew. Chem. Int. Ed., 2007, 46, 5591. CrossRef
36. R. Sakamoto, S. Saito, S. Shimizu, Y. Inokuma, N. Aratani, and A. Osuka, Chem. Lett., 2010, 39, 439. CrossRef
37. H. Rexhausen and A. Gossauer, J. Chem. Soc., Chem. Commun., 1983, 275. CrossRef
38. a) J.-Y. Shin, H. Furuta, and A. Osuka, Angew. Chem. Int. Ed., 2001, 40, 619; CrossRef b) S. Mori, J.-Y. Shin, S. Shimizu, F. Ishikawa, H. Furuta, and A. Osuka, Chem. Eur. J., 2005, 11, 2417. CrossRef
39. T. Yoneda, H. Mori, B. S. Lee, M.-C. Yoon, D. Kim, and A. Osuka, Chem. Commun., 2012, 48, 6785. CrossRef
40. T. Higashino and A. Osuka, Chem. Sci., 2012, 3, 103. CrossRef
41. M. G. P. M. S. Neves, R. M. Martins, A. C. Tomé, A. J. D. Silvestre, A. M. S. Silva, V. Félix, M. G. B. Drew, and J. A. S. Cavaleiro, Chem. Commun., 1999, 385. CrossRef
42. a) M. Suzuki and A. Osuka, Chem. Eur. J., 2007, 13, 196; CrossRef b) T. Koide, G. Kashiwazaki, M. Suzuki, K. Frukawa, M.-C. Yoon, S. Cho, D. Kim, and A. Osuka, Angew. Chem. Int. Ed., 2008, 47, 9661. CrossRef
43. a) S. Shimizu, J.-Y. Shin, H. Furuta, R. Ismael, and A. Osuka, Angew. Chem. Int. Ed., 2003, 42, 78; CrossRef b) S. Shimizu, N. Aratani, and A. Osuka, Chem. Eur. J., 2006, 12, 4909; CrossRef c) T. Koide, K. Youfu, S. Saito, and A. Osuka, Chem. Commun., 2009, 6047. CrossRef
44. Y.-S. Xie, K. Yamaguchi, M. Toganoh, H. Uno, M. Suzuki, S. Mori, S. Saito, A. Osuka, and H. Furuta, Angew. Chem. Int. Ed., 2009, 48, 5496. CrossRef
45. J. Sankar, S. Mori, S. Saito, H. Rath, M. Suzuki, Y. Inokuma, H. Shinokubo, K. S. Kim, Z. S. Yoon, J.-Y. Shin, J. M. Lim, Y. Matsuzaki, O. Matsushita, A. Muranaka, N. Kobayashi, D. Kim, and A. Osuka, J. Am. Chem. Soc., 2008, 130, 13568. CrossRef
46. T. Higashino, J. M. Lim, T. Miura, S. Saito, J.-Y. Shin, D. Kim, and A. Osuka, Angew. Chem. Int. Ed., 2010, 49, 4950. CrossRef
47. S. Saito, J.-Y. Shin, J. M. Lim, K. S. Kim, D. Kim, and A. Osuka, Angew. Chem. Int. Ed., 2008, 47, 9657. CrossRef
48. T. Higashino, B. S. Lee, J. M. Lim, D. Kim, and A. Osuka, Angew. Chem. Int. Ed., doi:10.1002/anie.201208147. CrossRef
49. D. B. Chesnut and L. D. Quin, Tetrahedron, 2005, 61, 12343. CrossRef
50. T. Miura, T. Higashino, S. Saito, and A. Osuka, Chem. Eur. J., 2010, 16, 55. CrossRef