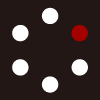
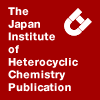
HETEROCYCLES
An International Journal for Reviews and Communications in Heterocyclic ChemistryWeb Edition ISSN: 1881-0942
Published online by The Japan Institute of Heterocyclic Chemistry
e-Journal
Full Text HTML
Received, 9th November, 2012, Accepted, 13th December, 2012, Published online, 21st December, 2012.
DOI: 10.3987/COM-12-12623
■ Role of CH/O and CH/π Interactions in Structural Stability. Effect on Endo Selectivity in 1,3-Dipolar Reaction of Electron-Deficient 4-Oxo-4H-pyrazole 1,2-Dioxide with N-Substituted Maleimides
Koki Yamaguchi, Kazunobu Harano, and Masashi Eto*
Faculty of Pharmaceutical Sciences, Sojo University, 4-22-1, Ikeda, Kumamoto-shi, 860-0082, Japan
Abstract
The molecular structure of the 1:2 cycloadduct derived from 1,3-dipolar reaction of 3,5-bis(methoxycarbonyl)-4-oxo-4H-pyrazole 1,2-dioxide (1a) with N-phenyl maleimide (2a) was established to be an anti-endo-endo form by the single crystal X-ray analysis. The weak attractive CH/O interaction between 1a and 2a is conjectured to play an important role in the preferential formation of the endo transition-state. The formation mechanism of the adduct is discussed on the basis of transition-state structures optimized at the B3LYP/6-31G(d) level of theory.INTRODUCTION
3,5-Disubstituted 4-oxo-4H-pyrazole 1,2-dioxide (1), which contains two nitrone moieties, undergoes 1,3-dipolar cycloaddition with various unsaturated compounds to give 1:1 cycloadducts.1,2 Usually, the remaining nitrone moiety is unreactive towards cycloaddition with excess dienophiles.1b In spite of the existence of an additional nitrone moiety in 1, formation of the 1:2 cycloadducts is yet to be reported. However, preliminary density functional theory (DFT) calculations for the model reaction of 1 with ethylene estimate that the reaction barrier in the secondary reaction is marginally higher than that of the primary reaction.1g This suggests that the possibility of cycloaddition between the remaining 1,3-dipole and ethylene moiety exists.
During the study of the reaction behaviour of 1, we isolated unexpectedly the heterocage compound produced by the sequential 1,3-dipolar reactions (involving an initial intermolecular cycloaddition and the subsequently successive intramolecular cycloaddition) of 3,5-bis(methoxycarbonyl)-4-oxo-4H-pyrazole 1,2-dioxide (1a) containing electron-deficient substituents with 1,3,5-cycloheptatriene (Scheme 1).1g This result prompted us to reinvestigate the reaction behaviour of 1 towards planar cyclic olefins to obtain a corresponding 1:2 cycloadduct. In this paper, we report the reaction behaviour of 1 towards N-substituted maleimides (2) and furnish the first example of the formation of the 1:2 cycloadduct.
RESULTS AND DISCUSSION
A mixture of 1a and N-phenylmaleimide (2a) in benzene was refluxed for 23 h to give a mixture of 1:1 cycloadducts (endo-3aa, 17% and exo-3aa, 10%), 1:2 cycloadduct (4aa, 22%), and isoxazole derivative (5aa, 1%) as shown in Scheme 2 and Table 1. The MS, 1H-NMR and 13C-NMR spectra of 3aa and 4aa were consistent with the 1:1 and 1:2 1,3-dipolar cycloaddition products, respectively. The endo/exo structures of 3aa were determined by comparing the NMR spectra. In exo-3aa, two methine protons (δ4.44 and 5.49) resonate at high-field regions when compared to those of the endo isomer (δ4.79 and 5.90), owing to the ring current effect of the R–C=N→O moiety.1b
Immediately after separation of 3aa by silica gel column chromatography, the endo/exo ratio was approximately 3:2. However, the amount of endo-form increased with time, suggesting that the isomerization from the exo-form to the endo-form gradually occurred in the solution (DMSO, acetone, or CHCl3). Interestingly, the colouration of the solution was observed during the course of the reaction. The spectra showed a maximum absorption in the vicinity of 600 nm. The visible absorption spectrum of the reaction mixture was not merely a cumulative result obtained by the addition of the spectra of the pure samples of the 1a and 2a, indicating the presence of a charge-transfer (CT) complex between 1a and 2a (Figure 1).
The 1H-NMR and 13C-NMR spectra of the 1:2 cycloadduct (4aa) indicated a highly symmetrical structure. Six stereoisomers of 4aa are possible with respect to the combination of endo/exo selectivity and syn/anti approach to the 1:1 cycloadduct (Figure 2). In the 1H-NMR spectrum of 4aa, a high-field shifted methyl signal of the methoxycarbonyl group was observed at δ3.14 due to the anisotropy of the N-phenyl group, in comparison with that of the 1:1 cycloadduct of 1a and 2a (δ3.78). This observation suggests that the orientation of the cycloaddition was the anti-endo-endo form. As shown in Figure 3, the structure of 4aa was established to be an anti-endo-endo 1:2 cycloadduct by the single crystal X-ray analysis, in which the N–N bond length and O–N–N–O dihedral angle were found to be 1.502(3) Å and 86.4(1)°, respectively. The S-shaped structure indicates that the cycloadditions at both stages proceeded through endo transition states. The analysis also suggests the presence of the CH/π interaction3 between the methyl hydrogen of the ester group and the phenyl ring, in which the nearest H3C•••C distance was 3.453(5) Å. A similar close contact was found between another ester group and the phenyl ring of the adjacent molecule [with H3C•••C distance of 3.506(4) Å]. To the best of our knowledge, this is the first demonstration of isolation of a 1:2 cycloadduct in the 1,3-dipolar cycloaddition of 1 with dipolarophiles.
The formation of the 1:2 cycloadduct (4aa) is attributed to the effective contribution of the neutral-type FMO interaction according to Sustmann’s classification4 of cycloadditions. The first step of the cycloaddition is controlled by the inverse-type interaction1b (i.e., by the combination of LUMO of 1a and HOMO of 2a). However, the second step is a neutral-type reaction, wherein both the HOMO/LUMO and LUMO/HOMO interactions are operative.
Similarly, 1a reacted with N-methyl maleimide (2b) to produce a 1:1 cycloadduct (endo-3ab, 32%) and a 1:2 cycloadduct (4ab, 20%) with the remnant reactant recovered. The stereochemistry of 4ab was confirmed and the product exhibited an anti-endo-endo form, which was inferred based on the similarity of the methine proton signals of 4aa.
In the reactions of 1a with 2, minor products (5aa or 5ab) were also obtained. The structure of 5aa was determined to be a dihydroisoxazole derivative based on the X-ray structure of 5ab. The derivative is probably produced from the extrusion of the carbonyl group of a 1:2 cycloadduct assisted by the weakened N–N and (O=)C–C(CO2Me) bonds.
In contrast to the reaction behaviour of 1a containing electron-deficient substituents, the reactions of the diphenyl derivative (1b) with 2 produced an endo/exo mixture of exclusively the 1:1 cycloadduct (endo-3ba, 36% and exo-3ba, 23%).5
In order to evaluate the differences between the reaction behaviours of 1a and 1b toward 2, DFT6 calculations at the B3LYP/6-31G(d) level were performed on the possible reaction pathways of 1 with 2b (Figure 4). For the primary step of the reaction between 1a and 2b, the GS energy of endo-3ab is found to be much smaller than that of exo-3ab, indicating the lower thermodynamic stability of the exo cycloadduct. In contrast to 1a, the ground-state (GS) energy of the exo adduct derived from the model reaction of the unsubstituted 4-oxo-4H-pyrazole 1,2-dioxide (1c) with 2a is 3.2 kcal/mol more stable than that of the endo product.7 The stability of endo-3ab could be attributed to the C–H/O=C< type interaction8 between the C3b-methine proton and the oxygen atom of the ester group (with the CH•••O distance of 2.304 Å). The transition-state (TS) calculation also suggested the contribution of intermolecular CH/O interaction (with the CH•••O distance of 2.504 Å) to the stabilization of the endo-TS, which is more stable than the exo-TS.9 The energy difference between two TS is 0.8 kcal/mol, which supports the observed endo/exo selectivity. Changing the substituent on nitrogen of maleimide from methyl to phenyl group may increase the steric congestion between the N-substituent and the ester group of 1a in the transition structure, resulting in a decrease in the preferential formation of the endo-TS in the primary reaction step between 1a and 2a.10
The secondary TS energy of the cycloaddition of 1b toward 2b is 23.3 kcal/mol, which is ca. 3 kcal/mol higher than that of the primary reaction step, as shown in Figure 4. For the reaction of 1a with 2b, the reaction barrier of the secondary step seems almost identical to that of the initial step. These calculations strongly suggest that the successive cycloaddition between the remaining 1,3-dipole of 3ab and 2b is possible. The difference in the secondary reaction barriers of endo-3ab and endo-3bb can be accounted on the basis of steric interferences and an attractive CH/O interaction between the substituents of the addends in the TS structures. The distance between the methine hydrogen adjacent to the carbonyl group and the ester oxygen atom on the ester group in the secondary TS structure of 3ab is 2.468Å, which seems to contribute the stabilization of the anti-endo-endo-TS (Figure 4). The TS energy is ca. 1.6 kcal/mol lower than that of the model rotated around the C–CO2Me bond without CH/O interaction.11 These calculations indicate that the CH/O interaction between 1a and 2 could play an important role in the preferential formation of the endo-TS.12
On the other hands, considering that the formation of the exo-3ab adduct, the formation of anti-endo-exo-3ab may be expected. However, the endo approach of 2b against exo-3ab might be unfavourable even if the contribution of the CH/O interaction exists. This is due to the steric interference between N-Me and the flexibility restricted C3a-CO2Me group by the exo-protruded maleimide ring.
In summary, the anti-endo-endo-1:2 cycloadduct was formed by the sequential 1,3-dipolar reactions of 1a containing electron-withdrawing substituents with maleimides (2). In these reactions, the weak attractive CH/O interaction in the TS affected the stereoselectivity of the 1,3-dipolar cycloadditon.
EXPERIMENTAL
Melting points were uncorrected. The IR spectra were taken with a Hitachi 270-30 spectrophotometer. 1H-NMR and 13C-NMR spectra were taken with JEOL JNM-EX 270 (270 MHz) and JNM-A 500 (500 MHz) spectrometers for ca.10% solution with TMS as an internal standard; chemical shifts are expressed as δ values and the coupling constants (J) are expressed in Hz. Mass spectra were obtained using a JEOL JMS-T100LC Accu TOF instrument.
Reaction of 1 with 2 (general procedure) – A solution of 0.6 g (2.5 mmol) of la in 10 mL of benzene and 0.87 g of 2a was heated under reflux for 23 h. The mixture was cooled and the solvent was removed under vacuum. The residue was chromatographed on silica gel. The products separated were crystallized from appropriate solvents.
endo-3aa: colorless prisms; mp 175–180 °C (from acetone); IR (KBr) 1773, 1728 cm-1; 1H-NMR (500 MHz, DMSO-d6) δ: 3.78 (s, CO2CH3, 3H), 3.91 (s, CO2CH3, 3H), 4.79 (d, J = 8.0, 1H, C3b-H), 5.90 (d, J = 8.0, 1H, C6a-H), 6.97 (d, J = 7.5, 1H, Ar-H), 7.27 (d, J = 6.9, 1H, Ar-H), 7.47–7.56 (m, 3H, Ar-H); 13C-NMR (125 MHz, DMSO-d6) δ: 53.1(C3b), 53.9 (CO2CH3), 54.8 (CO2CH3), 85.8 (C6a), 83.3 (C-COO), 114.6 (C2), 155.0 (COO), 162.7 (COO), 169.6 (NC=O), 169.9 (NC=O), 177.9 (C=O); HRMS (ESI) Calcd. for C18H17N3O10Na (M++CH3OH+Na): 458.08116. Found: 458.08268.
exo-3aa: obtained as an endo/exo mixture; 1H-NMR (500 MHz, DMSO-d6) δ: 3.75 (s, 3H, CO2CH3), 3.84 (s, 3H, CO2CH3), 4.44 (d, J = 7.5, 1H, C3b-H), 5.49 (d, J = 7.5, 1H, C6a-H), 6.97 (d, J = 7.5, 1H, Ar-H), 7.47–7.56 (m, 4H, Ar-H); 13C-NMR (125 MHz, DMSO-d6) δ: 52.5 (CO2CH3), 52.6 (CO2CH3), 54.4 (C3b), 79.1 (C-COO), 83.4 (C6a), 117.1 (C2), 156.0 (COO), 162.2 (COO), 168.1 (NC=O), 170.3 (NC=O), 180.7 (C=O).
anti-endo-endo-4aa: colorless prisms; mp 229–230 °C (from acetone-CHCl3); IR (KBr) 1724 cm-1; 1H-NMR (500 MHz, DMSO-d6) δ: 3.14 (s, 3H, CO2CH3), 4.59 (d, J = 8.0, 1H, >CH-C(=O)), 5.56 (d, J = 8.0, 1H, -OCH-C(=O)), 7.29 (d, J = 7.5, 2H, ArH), 7.45 (dd, J = 7.5, 7.5, 1H, ArH), 7.56 (dd, J = 7.5, 7.5, 2H, ArH); 13C-NMR (125 MHz, DMSO-d6) δ 53.7 (>CH-C(=O)), 54.0 (COOCH3), 81.0 (-OCH-C(=O)), 84.0 (C-COO), 162.2 (COO), 170.4 (NC=O), 172.1 (NC=O), 183.0 (C=O); m/z (FAB): 577 (M++1). Anal. Calcd for C27H20N4O11 + C3H6O (Acetone): C, 56.78; H, 4.13; N, 8.83. Found: C, 56.69; H, 3.89; N, 8.91.
5aa: colorless prisms; mp 224–225 °C (from acetone); IR (KBr) 1726 cm-1; 1H-NMR (500 MHz, DMSO-d6) δ: 3.84 (s, 3H, CO2CH3), 4.93 (d, J = 9.7, 1H, C3a-H), 5.81 (d, J = 9.7, 1H, C6a-H), 7.32 (brd, J = 7.7, 2H, ArH), 7.44–7.52 (m, 3H, ArH); 13C-NMR (125 MHz, DMSO-d6) δ: 52.9 (CO2CH3), 54.4 (C3a), 83.2 (C6a), 148.3 (C3), 158.8 (COO), 169.2 (NC=O), 171.1 (NC=O); HMRS (ESI) Calcd. for C14H14N2O6Na (M++CH3OH+Na): 329.07496. Found: 329.07423. Anal. Calcd for C13H10N2O5: C, 56.94; H, 3.68; N, 10.22. Found: C, 56.58; H, 3.67; N, 10.12.
endo-3ab: pale yellow prisms; mp 110–114 °C (from CHCl3); IR (KBr) 1732 cm-1; 1H-NMR (500 MHz, DMSO-d6) δ: 2.73 (s, 3H, CH3), 3.80 (s, 3H, CO2CH3), 3.88 (s, 3H, CO2CH3), 4.65 (d, J = 8.0, 1H, C3b-H), 5.71 (d, J = 8.0, 1H, C6a-H); 13C-NMR (125 MHz, DMSO-d6) δ: 25.2 (CH3) 52.1 (C3b), 53.1 (CO2CH3), 54.8 (CO2CH3), 82.9 (C-COO), 85.6 (C6a), 155.0 (COO), 162.9 (COO), 170.5 (NC=O), 170.7 (NC=O), 178.0 (C=O); HMRS (ESI) Calcd. for C12H10N3O9 (M+–H): 340.04170. Found: 340.03828.
4ab: colorless prisms; mp 210–211 °C (from acetone); IR (KBr) 1712 cm-1; 1H NMR (500 MHz, DMSO-d6) δ 2.82 (s, 3H, CH3), 3.76 (s, 3H, CO2CH3), 4.38 (d, J = 8.0, 1H, >CH-C(=O)), 5.33 (d, J = 8.0, 1H, -OCH-C(=O)); 13C NMR (125 MHz, DMSO-d6) δ 25.3 (CΗ3), 53.9 (>CH-C(=O)), 54.8 (CO2CH3), 80.5 (-OCH-C(=O)), 83.7 (C-COO), 162.2 (COO), 171.3 (NC=O), 173.3 (NC=O), 183.0 (C=O); Anal. Calcd for C17H16N4O11: C, 45.14; H, 3.57; N, 12.39. Found: C, 45.40; H, 3.81; N, 12.25.
5ab: colorless prisms; mp 205–208 °C (from acetone-CHCl3); IR (KBr) 1726 cm-1; 1H-NMR (500 MHz, DMSO-d6) δ: 2.85 (s, CH3, 3H), 3.83 (s, CO2CH3, 3H), 4.77 (d, J = 9.7, 1H, C3a-H), 5.65 (d, J = 9.7, 1H, C6a-H); 13C-NMR (125 MHz, DMSO-d6) δ: 25.1 (CH3), 52.9 (C3a), 54.2 (CO2CH3), 83.0 (C6a), 148.2 (C3), 158.8 (COO), 170.2 (NC=O), 171.9 (NC=O); HMRS (ESI) Calcd. for C9H12N2O6Na (M++CH3OH+Na): 267.05931. Found: 267.05428.
endo-3ba: colorless prisms; mp 249–252 °C (from acetone); IR (KBr) 1736 cm-1; 1H-NMR (500 MHz, DMSO-d6) δ: 4.64 (d, J = 8.0, 1H, C3b-H), 5.90 (d, J = 8.0, 1H, C6a-H), 6.76 (d, J = 7.7, 2H, Ar-H), 7.26 (dd, J = 7.7, 7.5, 2H, Ar-H), 7.33 (dd, J = 7.5, 6.9, 1H, Ar-H), 7.52–7.60 (m, 6H, Ar-H), 7.72 (d, J = 7.7, 2H, Ar-H), 8.11 (dd, J = 6.9, 1.7, 2H, Ar-H); 13C-NMR (125 MHz, DMSO-d6) δ: 57.3 (C3b), 83.2 (C3a), 85.0 (C6a), 170.7 (NC=O), 171.6 (NC=O), 185.5 (C=O); Anal. Calcd for C25H17N3O5: C, 68.33; H, 3.90; N, 9.56. Found: C, 68.32; H, 3.76; N, 9.40.
exo-3ba: colorless prisms; mp 215–216 °C (from acetone); IR (KBr) 1714 cm-1; 1H-NMR (500 MHz, DMSO-d6) δ: 4.83 (d, J = 8.0, 1H, C3b-H), 5.68 (d, J = 8.0, 1H, C6a-H), 6.66 (dd, J = 7.5, 1.7, 2H, Ar-H), 7.39 (d, J = 7.5, 3H, Ar-H), 7.48 (d, J = 7.5, 3H, Ar-H), 7.79 (d, J = 7.5, 2H, Ar-H), 7.59 (d, J = 8.6, 3H, Ar-H), 8.30 (dd, J = 8.6, 1.7, 2H, Ar-H); 13C-NMR (125 MHz, DMSO-d6) δ: 56.2 (C3b), 78.5 (C6a), 84.2 (C3a), 169.2 (NC=O), 169.4 (NC=O), 188.6 (C=O); HMRS (ESI) Calcd. for C26H21N3O6Na (M++CH3OH+Na): 494.13280. Found: 494.13262.
endo-3bb: colorless prisms; mp 249–252 °C (from n-hexane-AcOEt); IR (KBr) 1736 cm-1; 1H-NMR (500 MHz, DMSO-d6) δ 2.58 (s, 3H, CH3), 4.49 (d, J = 8.0, 1H, C3b-H), 5.71 (d, J = 8.0, 1H, C6a-H), 7.50–7.57 (m, 6H, Ar-H), 7.66 (d, J = 7.5, 2H, Ar-H), 7.99–8.01 (m, 2H, Ar-H); 13C-NMR (125 MHz, DMSO-d6) δ 24.8 (CH3), 57.0 (C3b), 82.9 (C3a), 84.8 (C6a), 171.4 (NC=O), 171.5 (NC=O), 185.2 (C=O); HMRS (ESI) Calcd. for C20H15N3O5Na (M++Na): 400.09094. Found: 400.08871.
exo-3bb: colorless prisms; mp 210–211 °C (from acetone); IR (KBr) 1720 cm-1; 1H-NMR (500 MHz, DMSO-d6) δ: 2.59 (s, 3H, CH3), 4.62 (d, J = 8.0, 1H, C3b-H), 5.48 (d, J = 8.0, 1H, C6a-H), 7.39–7.43 (m, 3H, Ar-H), 7.57–7.58 (m, 3H, Ar-H), 7.68–7.70 (m, 2H, Ar-H), 8.27 (dd, J = 7.7, 1.7, 2H, Ar-H); 13C-NMR (125 MHz, DMSO-d6): δ 24.7 (CH3), 56.2 (C3b), 78.7 (C3a), 83.4 (C6a), 169.8 (NC=O), 170.0 (NC=O), 189.3 (C=O); Anal. Calcd for C20H15N3O5: C, 63.66; H, 4.01; N, 11.14. Found: C, 63.73; H, 4.28; N, 10.87.
Crystal structure analysis – The single crystals for inclusion complexes were prepared by slow evaporation of solution at room temperature. All measurements were performed on RAXIS RAPID imaging plate area detector with graphite-monochromated Mo-Kα radiation (λ=0.7107 Å). The data were collected at a temperature of 23 ± 1 °C to a maximum 2θ value of 55°. The structure was solved by direct method (SIR9213a), and all hydrogen atoms were located at calculated positions. The structure was refined by a full-matrix least-squares technique using anisotropic thermal parameters for non-hydrogen atoms and a riding model for hydrogen atoms. All calculations were performed using the crystallographic software package Crystal Structure.13b,c These X-ray crystallographic data have been deposited at the Cambridge Crystallographic Data Centre (CCDC). Crystal data of anti-endo-endo-4aa: C27H20N4O11•C3H6O (4aa+acetone), M=634.55, monoclinic, Space group P21/c, a=9.635 (1), b=11.409 (2), c=26.545 (1) Å, β=94.297 (8) °, V=2909.7 (6) Å3, Dc=1.448 g cm-3, Z=4, R=0.057, Rw=0.100. CCDC ref. No. 695559; 5ab: C8H8N2O5, M=212.16, orthorhombic, Space group Pbca, a=14.402 (4), b=13.171 (4), c=9.650 (4) Å, V=1830 (1) Å3, Dc=1.583 g cm-3, Z=8, R=0.042, Rw=0.044, CCDC ref. No. 696187; endo-3bb: C20H15N3O5, M=377.36, monoclinic, Space group P21/n, a=10.193 (2), b=10.496 (2), c=16.406 (3) Å, β=92.247 (6) °, V=1750.4 (5) Å3, Dc=1.432 g cm-3, Z=4, R=0.090, Rw=0.135, CCDC ref. No. 908115.
Measurement of visible absorption spectra – Solutions of 1a (2.9 mmol/L), 2a (3.8 mmol/L), and 3a (3.7 mmol/L) in CHCl3 were used for the measurement at 25 °C.
Molecular orbital (MO) calculations – The MO calculations were performed by density functional theory (DFT) based ab initio method at the B3LYP/6-31G(d) level using Gaussian09 program package.6 Vibrational frequencies were used to characterize the nature of the stationary points. Zero point energy (ZPE) corrections were scaled by 0.9804.14
ACKNOWLEDGEMENTS
We thank Ms. R. Haraguchi and Mr. K. Egami for experimental assistance.
References
1. a) M. Eto, K. Harano, Y. Yoshitake, and T. Hisano, J. Heterocycl. Chem., 1993, 30, 1557; CrossRef b) M. Eto, Y. Yoshitake, K. Harano, and T. Hisano, J. Chem. Soc., Perkin Trans. 2, 1994, 1337; CrossRef c) Y. Yoshitake, M. Eto, and K. Harano, Heterocycles, 1997, 45, 1873; CrossRef d) Y. Yoshitake, M. Eto, and K. Harano, Tetrahedron Lett., 1998, 39, 2761; CrossRef e) Y. Yoshitake, M. Eto, and K. Harano, Chem. Pharm. Bull., 1999, 47, 601; CrossRef f) K. Yamaguchi, Y. Yoshitake, and K. Harano, Heterocycles, 2009, 78, 2777; CrossRef g) K. Yamaguchi, M. Eto, Y. Yoshitake, and K. Harano, J. Phys. Org. Chem., 2013, in press.
2. a) J. P. Freeman, J. J. Gannon, and D. L. Surbey, J. Org. Chem., 1969, 34, 187; CrossRef b) J. P. Freeman and M. L. Hoare, J. Org. Chem., 1971, 36, 19; CrossRef c) A. Kotali and V. P. Papageorgiou, Org. Prep. Proced. Int., 1991, 23, 611; d) A. Kotali and P. G. Tsoungas, Heterocycles, 1989, 29, 1615. CrossRef
3. a) M. Nishio, M. Hirota, and Y. Umezawa, The CH/π interaction; Evidence Nature and Consequences, Wiley, New York, 1998; b) O. Takahashi, Y. Kohno, and M. Nishio, Chem. Rev., 2010, 110, 6049; CrossRef c) M. Nishio, Phys. Chem. Chem. Phys., 2011, 13, 13873. CrossRef
4. R. Sustmann, Tetrahedron Lett., 1971, 12, 2717; CrossRef 2721. CrossRef
5. The endo/exo stereochemistries of 3ba and 3bb were determined by analysing the NOE (nuclear Overhauser effect) data. In the case of endo-3bb, which was subjected to single crystal X-ray analysis, the protons of the phenyl group attached to the C3a position correlated to the C3b methine proton. However, such a correlation was not observed in the case of exo-3bb.
6. Gaussian 09, Revision A.1, M. J. Frisch, G. W. Trucks, H. B. Schlegel, G. E. Scuseria, M. A. Robb, J. R. Cheeseman, G. Scalmani, V. Barone, B. Mennucci, G. A. Petersson, H. Nakatsuji, M. Caricato, X. Li, H. P. Hratchian, A. F. Izmaylov, J. Bloino, G. Zheng, J. L. Sonnenberg, M. Hada, M. Ehara, K. Toyota, R. Fukuda, J. Hasegawa, M. Ishida, T. Nakajima, Y. Honda, O. Kitao, H. Nakai, T. Vreven, J. A. Montgomery, Jr., J. E. Peralta, F. Ogliaro, M. Bearpark, J. J. Heyd, E. Brothers, K. N. Kudin, V. N. Staroverov, R. Kobayashi, J. Normand, K. Raghavachari, A. Rendell, J. C. Burant, S. S. Iyengar, J. Tomasi, M. Cossi, N. Rega, J. M. Millam, M. Klene, J. E. Knox, J. B. Cross, V. Bakken, C. Adamo, J. Jaramillo, R. Gomperts, R. E. Stratmann, O. Yazyev, A. J. Austin, R. Cammi, C. Pomelli, J. W. Ochterski, R. L. Martin, K. Morokuma, V. G. Zakrzewski, G. A. Voth, P. Salvador, J. J. Dannenberg, S. Dapprich, A. D. Daniels, Ö. Farkas, J. B. Foresman, J. V. Ortiz, J. Cioslowski, and D. J. Fox, Gaussian, Inc., Wallingford CT, 2009. The calculation data (atomic coordinates) are available upon request (e-mail address: meto@ph.sojo-u.ac.jp).
7. exo-3ca: E=–849.3030 (EZPE=–849.1309) hartree; endo-3ca: –849.2979 (EZPE=–849.1258) hartree.
8. a) R. Taylor and O. Kennard, J. Am. Chem. Soc., 1982, 104, 5063; CrossRef b) G. R. Desiraju, Acc. Chem. Res., 1991, 24, 290; CrossRef c) G. R. Desiraju, Acc. Chem. Res., 1996, 29, 441; CrossRef d) T. Steiner, J. A. Kanters, and J. Kroon, J. Chem. Soc., Chem. Commun., 1996, 1277; e) T. Steiner, Cryst. Rev., 1996, 6, 1; CrossRef f) T. Steiner, Angew. Chem. Int. Ed., 2002, 41, 48. CrossRef
9. In the TS structures from 1b and 2b without CH/O interaction, there is almost no energy difference between the exo-TS and endo-TS [exo-TS : E=–1311.3626 (EZPE=–1311.0252) hartree; endo-TS : see Figure 4].
10. Calculations of the endo-TS for the reaction of 1a with 2a by B3LYP/6-31G(d) suggested the presence of steric interference between the ester and the phenyl groups. In comparison to the TS for the reaction of 1a with 2b, the distance between the nitrogen of maleimide and the nitrogen of >C=N→O moiety is separated by ca. 0.14 Å.
11. The B3LYP/6-31G(d) calculation for the rotation model without CH/O interaction also gave stationary point [E=–1703.7515 (EZPE=–1703.3830) hartree].
12. Similar interactions are also observed in Diels-Alder reactions. The B3LYP/6-31G(d) calculated TS structures for the cycloaddition of 2,5-bis(methoxycarbonyl)-3,4-diphenylcyclopentadienone with 2b indicate that the endo-TS is stabilized by the O/H-C interactions not only between the ester carbonyl oxygen and the 2'-hydrogen of the phenyl group (Ph-H•••O= 2.473Å) but also between the proton of 2b and the ester ether oxygen (CH•••O< 2.409Å). The endo-TS energy was ca. 2.1 kcal/mol more stable than that of exo-TS. Detailed study is in progress.
13. a) SIR2008: M. C. Burla, R. Caliandro, M. Camalli, B. Carrozzini, G. Cascarano, L. De Caro, C. Giacovazzo, G. Polidori, D. Siliqi, and R. Spagna, J. Appl. Cryst., 2007, 40, 609; CrossRef b) CrystalStructure 4.0: Crystal Structure Analysis Package, Rigaku Corporation (2000–2010). Tokyo 196-8666, Japan.
14. A. P. Scott and L. Radom, J. Phys. Chem., 1996, 100, 10502.