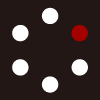
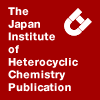
HETEROCYCLES
An International Journal for Reviews and Communications in Heterocyclic ChemistryWeb Edition ISSN: 1881-0942
Published online by The Japan Institute of Heterocyclic Chemistry
e-Journal
Full Text HTML
Received, 12th November, 2012, Accepted, 30th November, 2012, Published online, 6th December, 2012.
DOI: 10.3987/COM-12-12624
■ Kinetic Resolution of Secondary Alcohols by Chiral DMAP Derivatives Prepared by the Ugi Multicomponent Reaction
Hiroki Mandai,* Shunsuke Irie, Masaru Akehi, Kazunobu Yuri, Masaaki Yoden, Koichi Mitsudo, and Seiji Suga*
Division of Chemistry and Biotechnology, Graduate School of Natural Science and Technology, Okayama University, 3-1-1 Tsushima-naka, Kita-ku, Okayama 700-8530, Japan
Abstract
The kinetic resolution of secondary alcohols was examined by new chiral DMAP derivatives, which can readily be prepared by the Ugi multicomponent reaction in a one-pot operation. The initial screening of DMAP derivatives indicated that the catalyst bearing L-valine with an S configuration at the α-position of amide showed the best stereoselectivity factor. After the reaction conditions were optimized with (S,S)-4a in the kinetic resolution of secondary alcohols, various acyclic and cyclic secondary alcohols could be resolved with an s-factor of up to 12.INTRODUCTION
The development of chiral nucleophilic catalysts is an important field of study in synthetic organic chemistry. Various catalysts have been developed to date and used in various asymmetric transformations, such as the kinetic resolution of racemic alcohols or amine, desymmetrization of meso-anhydrides, and carbon-carbon bond-forming reactions.1 In kinetic resolution, the catalyst is evaluated in terms of Kagan's equation, which gives the stereoselectivity factor (s).2 In general, a catalyst with an s-factor of greater than 20 is considered to be synthetically useful.3 However, such chiral catalysts sometimes require a multistep synthesis and/or cumbersome resolution of the racemate of the catalyst (optical resolution or chiral HPLC separation). Furthermore, in most cases, precise modification of the catalysts is not easy. Therefore, a short and practical synthesis of chiral DMAP derivatives, which can efficiently promote asymmetric transformations, is highly desirable.
Recently, we developed a diastereoselective Ugi reaction4 of a 4-(N,N-dimethylamino)pyridine (DMAP)-based aldehyde with various α-amino acids and tert-butyl isocyanide (Eq. 1).5
The reactions of 4-(dimethylamino)-3-pyridinecarboxaldehyde (1) with various α-amino acids gave the 3-substituted chiral DMAP derivatives in moderate yield (18%–63%) with good to high diastereoselectivity (up to 92:8 d.r.). This new synthetic method for accessing 3-substituted chiral DMAP derivatives6 offers several advantages: (1) highly functionalized chiral DMAP derivatives can be easily prepared by a one-pot operation; (2) each component (aldehyde, α-amino acid, and isocyanide) is readily available; and (3) diastereomerically pure DMAP derivatives can be potentially obtained by traditional purification techniques. Our preliminary studies showed that the major diastereomer of the Ugi product7 had a moderate s-factor (s = 2.3) in the kinetic resolution of 1-phenylethyl alcohol under unoptimized reaction conditions.
In this paper, we report the details of the kinetic resolution of various secondary alcohols using diastereomerically pure chiral DMAP derivatives.
RESULTS AND DISCUSSION
We began by examining the kinetic resolution of racemic 1-phenylethyl alcohol (2a) with an array of single diastereomers of DMAP derivatives possessing a chiral side chain at the C-3 position of the DMAP moiety, which could be obtained through a diastereoselective Ugi reaction, followed by a traditional purification technique (flash chromatography on SiO2).5 As illustrated in Table 1, the kinetic resolution of rac-2a was carried out with 0.75 equivalents of acetic anhydride and triethylamine in the presence of 5 mol % catalyst in toluene at 0 °C for 15 h. Catalysts 4a−d having an R configuration at the α-position of the amide with an alkyl-substituted α-amino acid (e.g., L-valine) showed s-factors of 1.9−2.2 (entries 1−4). Catalysts with a heteroatom-substituted α-amino acid resulted in similar s-factors (s = 2.2−2.4, entries 5 and 6). However, a minor diastereomer of the Ugi product,8 which has an S configuration at the α-position of the amide bearing L-valine or L-methionine [(S,S)-catalyst 4a and 4e], showed a slightly better s-factor (s = 3.4) than those obtained with the corresponding (S,R)-catalysts 4a and 4e (s = 2.1 and 2.2). Amide-modified catalysts (S,R)-4g and (S,R)-4h were ineffective (s = 1.8 and 1.0) in the current reaction (entries 9 and 10 vs 1). DMAP derivatives possessing a chiral side chain at the C-2 position of the DMAP moiety, (S,RS)-4i9 did not catalyze the reaction, probably due to the steric hindrance around the nucleophilic site. According to these results, (S,S)-4a was thought to be the best catalyst among those tested, and we decided to use (S,S)-4a for further optimization of the reaction conditions.
To increase the efficiency of resolution, we next examined the solvent effect with (S,S)-4a (Table 2). Solvents such as dichloromethane and diethyl ether decreased the s-factor to 2.0 and 3.1, respectively, compared to that with toluene (entries 2 and 3 vs 1). Polar solvents, such as acetonitrile, t-amyl alcohol, and ethyl acetate, resulted in low s-factors of 1.7−2.7 (entries 4−6). Although we did not find any correlation between the s-factor and the nature of solvent, toluene was selected as the optimal solvent in view of the s-factor.
To clarify the effect of the acylating reagent, sterically hindered anhydrides were used in the kinetic resolution (Table 3). Propionic anhydride and isobutyric anhydride did not improve the s-factor compared to that with acetic anhydride, while showing almost the same reactivity as acetic anhydride (entries 2 and 3 vs 1). Based on these results, acetic anhydride was thought to be the best acylating reagent in the current reaction.
Next, the effect of auxiliary base was investigated to improve the s-factor. The use of Hünig's base (DIPEA) decreased the s-factor (s = 3.5). Cyclic bases including DBU, N-methylmorpholine (NMM), and N-methylpiperidine (NMP) were found to be slightly inferior to Et3N with respect to the s-factor.
We next examined the concentration of substrate and the reaction temperature, since these might be important for achieving high enantioselectivity (Tables 5 and 6). As shown in Table 5, various concentrations
of substrate were tested. The reactions at lower concentrations were slightly better than the control reaction (0.5 M) while retaining satisfactory conversions of 2a (entries 3−5 vs 1). At a lower concentration (0.05 M), the reaction at -78 °C gave the highest s-factor (s =8.1; Table 6; entry 5). 10
With the optimal conditions in hand, we then subjected various secondary alcohols to kinetic resolution with (S,S)-4a, as shown in Figure 1. Naphthyl-based carbinols 2b and 2c could be resolved with s = 9.3 and 9.1, respectively. Phenyl-based carbinol 2d possessing a sterically hindered substituent (t-Bu) could also be used in the kinetic resolution with s =12.4, indicating that the steric environment of the substrate affected on the s-factor. On the other hand, propargylic alcohols 2e and 2f resulted in low s-factors (s = 2.8 and 3.8) compared to 2a−d, and were considered to be unsuitable substrates in the kinetic resolution with (S,S)-4a. The cyclic alcohol 2g with a phenyl substituent at the α-position gave s = 10.4, whereas the reaction of mono-protected cis-1,2-cyclohexanediol 2h proceeded with s = 2.6. Based on these results, aryl-substituted carbinols 2a−d and 2g could be resolved by the new catalyst (S,S)-4a with a satisfactory s-factor. Although we only confirmed the reaction kinetics (s-factor) for various alcohols with (S,S)-4a at a certain reaction time (15 h), for obtaining high enantiopurity of the recovering alcohols, longer reaction time (higher conversion) should be required according to the corresponding s-factor.2
In conclusion, we found that a minor diastereomer of the Ugi product, (S,S)-4a, can catalyze the kinetic resolution of secondary alcohols with s-factors of up to 12. To the best of our knowledge, this is the first example of the kinetic resolution of secondary alcohols with a chiral DMAP derivative that was prepared by the diastereoselective Ugi multicomponent reaction.11 This new approach for accessing chiral DMAP derivatives in a one-pot operation may become an attractive tool for constructing nucleophilic catalyst libraries. Studies on the further optimization of the catalyst structure and the use of a major diastereomer of the Ugi product [(S,R)-catalyst] in other important classes of asymmetric transformations are currently in progress.
EXPERIMENTAL
All melting points were determined using a Yanaco micro melting point apparatus MP-S3 and are uncorrected. Solvents were generally distilled and dried by standard procedures prior to use.12 The IR spectra were recorded on a JASCO FT/IR-4100 spectrometer. NMR spectra were recorded on a Varian VNMRS-400 spectrometer at the SC-NMR Laboratory (Okayama University), operating at 400 MHz for 1H NMR and 100 MHz for 13C NMR. Chemical shifts in CDCl3 are reported on the δ scale relative to CHCl3 (7.26 ppm) as an internal reference for 1H NMR. For 13C NMR, chemical shifts are reported on the δ scale relative to CHCl3 (77.0 ppm) as an internal reference. Column chromatography was performed with silica gel 60N (spherical, neutral, 40–50 µm) purchased from KANTO CHEMICAL. Optical rotations were measured on a HORIBA Model SEPA-300 High-sensitive polarimeter. High-resolution FAB mass spectra (HRMS) were measured on a JEOL JMS-700 MStation at the Mass Spectrometry Facility (Okayama University). The enantiomeric ratio (er) (= enantiomeric composition) and enantiomeric excess (ee) were determined by HPLC or GC analysis. HPLC was performed on Shimazu HPLC systems consisting of the following: pump, LC-10AD; detector, SPD-10A, 254 nm; column, DAICEL CHIRALCEL OD-H; mobile phase, hexane/i-PrOH. GC was performed on Shimazu chromatograph GC-14B (CP-Cyclodextrin-B-2,3,6-M-19 (0.25 mm, 0.25 µm, 25 m) in comparison with authentic racemic materials.
Preparation of chiral DMAP derivatives
The preparation of and analytical data for the catalysts (S,R)-4a−f and (S,RS)-4j were reported previously.5
(S)-N-(1-(tert-Butylcarbamoyl)-1-(4-(dimethylamino)pyridin-3-yl)methyl)-L-valine methyl ester ((S,S)-4a). colorless solid; mp 135-136 °C; 1H NMR (400 MHz, CDCl3) δ 8.36 (s, 1H), 8.32 (d, J = 5.6 Hz, 1H), 7.74 (br, 1H), 6.86 (d, J = 5.6 Hz, 1H), 4.41 (s, 1H), 3.58 (s, 3H), 3.01 (br, 1H), 2.88 (s, 6H), 2.11 (br, 1H), 1.93−1.85 (m, 1H), 1.39 (s, 9H), 0.98 (d, J = 6.8 Hz, 6H); 13C NMR (100 MHz, CDCl3) δ 174.6, 171.0, 159.1, 149.6, 149.3, 113.9, 67.5, 59.7, 51.6, 50.8, 44.5, 31.6, 28.7, 19.8, 18.6; IR (KBr) υ = 3194, 2963, 1732, 1671 cm−1; HRMS-FAB (m/z): [M+H]+ calcd. for C19H33N4O3 365.2553, found 365.2548; [α]D25 +43.0 (c 0.145, MeOH).
(S)-N-(1-(tert-Butylcarbamoyl)-1-(4-(dimethylamino)pyridyn-3-yl)methyl)-L-methionine methyl ester ((S,S)-4e). colorless solid; mp 99-100 °C; 1H NMR (400 MHz, CDCl3) δ 8.36 (s, 1H), 8.33 (d, J = 5.6 Hz, 1H), 7.60 (br, 1H), 6.88 (d, J = 5.6 Hz, 1H), 4.47 (s, 1H), 3.60 (s, 3H), 3.43 (br, 1H), 2.87 (s, 6H), 2.63 (d, J = 7.1 Hz, 1H), 2.61 (d, J = 7.1 Hz, 1H), 2.28 (br, 1H), 2.11 (s, 3H), 2.01−1.92 (m, 1H), 1.88−1.79 (m, 1H), 1.40 (s, 9H); 13C NMR (100 MHz, CDCl3) δ 174.4, 170.8, 159.1, 149.8, 149.6, 129.0, 114.0, 59.9, 59.7, 52.0, 50.9, 44.5, 32.7, 30.6, 28.7, 15.5; IR (KBr) υ = 3309, 2991, 2960, 2382, 1727, 1673 cm−1; HRMS-FAB (m/z): [M+H]+ calcd. for C19H33N4O3S 397.2273, found 397.2279; [α]D25 −4.54 (c 0.125, MeOH).
(R)-N-(1-(1,1,3,3-Tetramethylbutylcarbamoyl)-1-(4-(dimethylamino)pyridin-3-yl)methyl)-L-valine methyl ester ((S,R)-4g). To a suspension of 4-(dimethylamino)-3-pyridinecarboxaldehyde (30.8 mg, 0.21 mmol) and L-valine (26.7 mg, 0.23 mmol) in dry MeOH (0.4 mL) in a screw-cap test tube was added 1,1,3,3-tetramethylbutyl isocyanide (39.6 µL, 0.23 mmol), and the reaction mixture was stirred for 36 h at 50 °C. The solvent was evaporated in vacuo and the resulting residue was purified by column chromatography on SiO2 (EtOAc only) to give (S,R)-4g as a colorless solid (37.8 mg, 45% yield as a single diastereomer); mp 113-114 °C; 1H NMR (400 MHz, CDCl3) δ 8.52 (s, 1H), 8.36 (d, J = 5.6 Hz, 1H), 7.24 (br, 1H), 6.88 (d, J = 5.6 Hz, 1H), 4.57 (s, 1H), 3.69 (s, 3H), 2.83 (s, 6H), 2.78−2.74 (m, 1H), 2.47 (br, 1H), 1.95−1.87 (m, 1H), 1.75 (d, J = 15 Hz, 1H), 1.62 (d, J = 15 Hz, 1H), 1.39 (s, 3H), 1.38 (s, 3H), 0.96 (s, 9H), 0.90 (d, J = 6.7 Hz, 3H), 0.84 (d, J = 6.7 Hz, 3H); 13C NMR (100 MHz, CDCl3) δ 174.6, 170.3, 159.5, 150.5, 149.9, 127.9, 113.7, 64.6, 58.6, 54.8, 52.4, 51.5, 44.3, 31.5, 31.4, 31.3, 28.9, 28.6, 19.2, 18.4; IR (KBr) υ = 3207, 3043, 2966, 1732, 1666 cm−1; HRMS-FAB (m/z): [M+H]+ calcd. for C23H40N4O3 421.3179, found 421.3173; [α]D25 −137 (c 0.735, MeOH).
(R)-N-(1-(Benzylcarbamoyl)-1-(4-(dimethylamino)pyridin-3-yl)methyl)-L-valine methyl ester ((S,R)-4h). To a suspension of 4-(dimethylamino)-3-pyridinecarboxaldehyde (30.3 mg, 0.20 mmol) and L-valine (26.2 mg, 0.22 mmol) in dry MeOH (0.4 mL) in a screw-cap test tube was added benzyl isocyanide (26.8 µL, 0.22 mmol), and the reaction mixture was stirred for 16 h at 50 °C. The solvent was evaporated in vacuo and the resulting residue was purified by column chromatography on SiO2 (EtOAc only) to give (S,R)-4h as a yellowish oil (18.6 mg, 23% yield as a single diastereomer); 1H NMR (400 MHz, CDCl3) δ 8.54 (s, 1H), 8.37 (d, J = 5.6 Hz, 1H), 7.62 (br, 1H), 7.33−7.20 (m, 5H), 6.87 (d, J = 5.6 Hz, 1H), 4.71 (s, 1H), 4.47 (dd, J = 15, 6.0 Hz, 1H), 4.42 (dd, J = 15, 6.0 Hz, 1H), 3.65 (s, 3H), 2.85−2.79 (m, 1H), 2.75 (s, 6H), 2.50 (br, 1H), 1.97−1.89 (m, 1H), 0.90 (d, J = 6.8 Hz, 3H), 0.84 (d, J = 6.8 Hz, 3H); 13C NMR (100 MHz, CDCl3) δ 174.6, 171.7, 159.5, 150.5, 150.0, 138.1, 128.7, 127.7, 127.6, 127.5, 113.8, 64.9, 58.5, 51.6, 44.2, 43.5, 31.3, 19.2, 18.5; IR (KBr) υ = 3339, 3171, 2955, 1738, 1666 cm−1; HRMS-FAB (m/z): [M+H]+ calcd. for C22H31N4O3 399.2396, found 399.2383; [α]D25 −147 (c 0.465, MeOH).
4.3. Kinetic Resolution of Racemic Secondary Alcohols
General Method: To a solution of chiral DMAP derivative (0.010 mmol), racemic secondary alcohol 2 (0.20 mmol) and triethylamine (21 µL, 0.15 mmol) in toluene (4.0 mL) at – 78 °C was added acetic anhydride (14 µL, 0.15 mmol), and the reaction mixture was stirred at the same temperature for 15 h. The reaction was quenched with MeOH and concentrated in vacuo. The resulting residue was filtered through a short plug of SiO2 (hexane/Et2O = 3:1, v/v) to give the acetate and the unreacted alcohol, which were subjected to HPLC analysis.
(R)-1-Phenylethyl acetate (3a). a colorless oil (17% conversion, 87:13 er); HPLC (DAICEL CHIRALCEL OD-H, hexane/i-PrOH = 19:1, 0.300 mL/min, 30 °C, 254 nm): Rt 15.1 min (major ester), 15.8 min (minor ester); 1H NMR (400 MHz, CDCl3) δ 7.41−7.29 (m, 5H), 5.94 (q, J = 6.6 Hz, 1H), 2.08 (s, 3H), 1.57 (d, J = 6.6 Hz, 1H); 13C NMR (100 MHz, CDCl3) δ 169.9, 141.5, 128,2, 127.6, 125.8, 72.0, 22.0, 21.0; IR (neat) υ = 3064, 3033, 2982, 2934, 1744 cm−1; [α]D20 +53.9 (c 0.105, CHCl3, 89:11 er); lit.,13 [α]D21 +43 (c 2.10, CHCl3 (er >99:<1)).
(R)-1-(2-Naphthyl)ethyl acetate (3b). a colorless oil (17% conversion, 89:11 er); HPLC (DAICEL CHIRALCEL OJ-H, hexane/i-PrOH = 19:1, 0.300 mL/min, 30 °C, 254 nm): Rt 24.3 min (major ester), 30.0 min (minor ester); 1H NMR (400 MHz, CDCl3) δ 7.90−7.86 (m, 4H), 7.55−7.49 (m, 3H), 6.13 (q, J = 6.6 Hz, 1H), 2.15 (s, 3H), 1.68 (d, J = 6.6 Hz, 3H); 13C NMR (100 MHz, CDCl3) δ 170.2, 138.9, 133.1, 132.9, 128.2, 127.9, 127.5, 126.1, 125.9, 124.9, 124.0, 72.3, 22.1, 21.2; IR (neat) υ = 3057, 3024, 2980, 2933, 1738 cm−1; [α]D20 +78.4 (c 0.400, CHCl3, 86:14 er); lit.,14 [α]D22 +122 (c 1.00, CHCl3 (er >99.5:<0.5)).
(R)-1-(1-Naphtyl)ethyl acetate (3c). a colorless oil (14% conversion, 89:11 er); HPLC (DAICEL CHIRALCEL OD-H, hexane/i-PrOH = 9:1, 0.300 mL/min, 30 °C, 254 nm): Rt 17.0 min (major ester), 20.7 min (minor ester); 1H NMR (400 MHz, CDCl3) δ 8.14 (d, J = 7.9 Hz, 1H), 7.91 (bd, J = 7.9 Hz, 1H), 7.84 (d, J = 7.9 Hz, 1H), 7.65 (d, J = 7.9 Hz, 1H), 7.60-7.49 (m, 3H), 6.71 (q, J = 6.6 Hz, 1H), 2.16 (s, 3H), 1.75 (d, J = 6.6 Hz, 3H); 13C NMR (100 MHz, CDCl3) δ 170.3, 137.3, 133.7, 130.2, 128.8, 128.4, 126.2, 125.6, 125.3, 123.1, 123.1, 69.3, 21.6, 21.3; IR (neat) υ = 3051, 2982, 2933, 1739 cm−1; [α]D20 +26.9 (c 0.375, CHCl3, 86:14 er); lit.,14 [α]D22 +52 (c 1.00, CHCl3 (er >99.5:<0.5)).
(R)-2,2-Dimethyl-1-phenylpropyl acetate (3d). a colorless oil (8% conversion, 92:8 er); GC (chiral capillary column CP-CYCLODEX β-236M, 100 °C for 5 min, rate of temperature increase 1.0 °C/min): Rt 26.2 min (minor ester), 26.7 min (major ester) ; 1H NMR (400 MHz, CDCl3) δ 7.34−7.24 (m, 5H), 5.51 (s, 1H), 2.10 (s, 3H), 0.95 (s, 9H); 13C NMR (100 MHz, CDCl3) δ 170.1, 138.4, 127.7, 127.5, 127.4, 82.7, 34.9, 26.0, 21.1; IR (neat) υ = 3033, 2971, 2907, 2871, 1742 cm−1; [α]D19 −87.2 (c 1.00×10-2, CHCl3, 93:7 er).
(S)-1-Phenyl-2-propyn-1-yl acetate (3e). a colorless oil (18% conversion, 72:28 er); GC (chiral capillary column CP-CYCLODEX β-236M, 100 °C for 5 min, rate of temperature increase 1.0 °C/min): Rt 24.1 min (major ester), 25.3 min (minor ester); 1H NMR (400 MHz, CDCl3) δ 7.56−7.53 (m, 2H), 7.43−7.35 (m, 3H), 6.46 (d, J = 2.3 Hz, 1H), 2.67 (d, J = 2.3 Hz, 1H), 2.12 (s, 1H); 13C NMR (100 MHz, CDCl3) δ 169.6, 136.4, 129.0, 128.6, 127.6, 80.2, 75.4, 65.2, 21.0; IR (neat) υ = 3288, 3066, 3035, 2937, 2126, 1742 cm−1; [α]D19 −4.5 (c 0.04, CHCl3, 62:38 er); lit.,15 [α]D25 -4.8 (c 0.25, CHCl3 (er >99.5:<0.5)).
(R)-4-Phenyl-3-butyn-2-yl acetate (3f). a colorless oil (19% conversion, 77:23 er); GC (chiral capillary column CP-CYCLODEX β-236M, 100 °C for 5 min, rate of temperature increase 1.0 °C/min): Rt 38.8 min (minor ester), 39.2 min (major ester); 1H NMR (400 MHz, CDCl3) δ 7.47−7.41 (m, 2H), 7.35−7.27 (m, 3H), 5.69 (q, J = 6.7 Hz, 1H), 2.11 (s, 3H), 1.58 (d, J = 6.7 Hz, 3H); 13C NMR (100 MHz, CDCl3) δ 170.0, 131.9, 128.6, 128.2, 122.2, 87.4, 84.5, 60.8, 21.5, 21.1; IR (neat) υ = 3058, 2989, 2936, 1743 cm−1; [α]D20 +87.4 (c 0.455, CHCl3, 77:23 er); lit.,16 [α]D20 +188 (c 1.10, CHCl3 (er 99.5:0.5)).
(1R, 2S)-trans-2-Phenylcyclohexyl acetate (3g). a colorless oil (14% conversion, 91:9 er); The product was hydrolyzed and the enantiomeric ratio was determined by HPLC analysis of alcohol 2g. (DAICEL CHIRALCEL OD-H, 0.46 cm φ × 25 cm, hexane/i-PrOH = 199:1, 1.00 mL/min, 30 °C, 220 nm): Rt 16.2 min (minor alcohol), 17.9 min (major alcohol); 1H NMR (400 MHz, CDCl3) δ 7.38−7.34 (m, 2H), 7.29−7.24 (m, 3H), 5.07 (ddd, J = 4.5, 4.5, 4.5 Hz, 1H), 2.78−2.71 (m, 1H), 2.23−2.20 (m, 1H), 2.05−1.85 (m, 3H), 1.84 (s, 3H), 1.71−1.36 (m, 4H); 13C NMR (100 MHz, CDCl3) δ 170.2, 143.0, 128.1, 127.4, 126.3, 75.7, 49.6, 33.7, 32.2, 25.7, 24.6, 20.8; IR (neat) υ = 3062, 3029, 2935, 2859, 1738 cm−1; [α]D20 −33.1 (c 0.270, CHCl3, 90:10 er).
(1S, 2R)-cis-2-Acetoxycyclohexyl benzoate (3h). a colorless oil (34% conversion, 68:32 er); GC (chiral capillary column CP-CYCLODEX β-236M, 100 °C for 5 min, rate of temperature increase 1.0 °C/min): Rt 89.2 min (minor ester), 89.8 min (major ester); 1H NMR (400 MHz, CDCl3) δ 8.06−8.03 (m, 2H), 7.58−7.54 (m, 1H), 7.47−7.43 (m, 2H), 5.34−5.32 (m, 1H), 5.10-5.06 (m, 1H), 2.03 (s, 3H), 2.01−1.90 (m, 2H), 1.78−1.65 (m, 4H), 1.57−1.43 (m, 2H); 13C NMR (100 MHz, CDCl3) δ 170.4, 165.7, 132.9, 130.5, 129.6, 128.3, 71.5, 71.2, 28.1, 27.4, 22.2, 21.2, 21.1; IR (neat) υ = 2942, 2865, 1719, 1602 cm−1; [α]D19 +16 (c 0.31, CHCl3, 68:32 er).
ACKNOWLEDGEMENTS
Financial support was provided by Grant-in-Aid for Young Scientists (Start-up, No. 21850020) from the Japan Society for the Promotion of Science (JSPS), Okayama Foundation for Science and Technology, Wesco Scientific Promotion, and Okayama University. We are grateful to the SC-NMR Laboratory of Okayama University for the NMR spectra.
References
1. V. P. Krasnov, D. A. Gruzdev, and G. L. Levit, Eur. J. Org. Chem., 2012, 1471; CrossRef (b) H. Pellissier, Adv. Synth. Catal., 2011, 353, 1613; CrossRef (c) M. D. Diaz de Villegas, J. A. Galvez, P. Etayo, R. Badorrey, and P. Lopez-Ram-de-Viu, Chem. Soc. Rev., 2011, 40, 5564; CrossRef (d) L.-W. Ye, J. Zhou, and Y. Tang, Chem. Soc. Rev., 2008, 37, 1140; CrossRef (e) R. P. Wurz, Chem. Rev., 2007, 107, 5570; CrossRef (f) E. Vedejs and M. Jure, Angew. Chem. Int. Ed., 2005, 44, 3974; CrossRef (g) J. L. Methot and W. R. Roush, Adv. Synth. Catal., 2004, 346, 1035; CrossRef (h) G. C. Fu, Acc. Chem. Res., 2004, 37, 542; CrossRef (i) C. E. Müller and P. R. Schreiner, Angew. Chem. Int. Ed., 2011, 50, 6012. CrossRef
2. H. B. Kagan and J. C. Fiaud, Top. Stereochem., 1988, 249. CrossRef
3. For selected examples of the kinetic resolution of secondary alcohols by a non-enzymatic catalyst with s > 20, see: (a) H. Mandai, K. Murota, K. Mitsudo, and S. Suga, Org. Lett., 2012, 14, 3486; CrossRef (b) X. Li, H. Jiang, E. W. Uffman, L. Guo, Y. Zhang, X. Yang, and V. B. Birman, J. Org. Chem., 2012, 77, 1722; CrossRef (c) V. B. Birman and X. Li, Org. Lett., 2008, 10, 1115; CrossRef (d) V. B. Birman and X. Li, Org. Lett., 2006, 8, 1351; CrossRef (e) S. J. Miller, Acc. Chem. Res., 2004, 37, 601; CrossRef (f) K. Ishihara, Y. Kosugi, and M. Akakura, J. Am. Chem. Soc., 2004, 126, 12212; CrossRef (g) S. Bellemin-Laponnaz, J. Tweddell, J. C. Ruble, F. M. Breitling, and G. C. Fu, Chem. Commun., 2000, 1009; CrossRef (h) T. Sano, K. Imai, K. Ohashi, and T. Oriyama, Chem. Lett., 1999, 28, 265; CrossRef (i) J. C. Ruble, H. A. Latham, and G. C. Fu, J. Am. Chem. Soc., 1997, 119, 1492; CrossRef (j) E. Vedejs and X. Chen, J. Am. Chem. Soc., 1996, 118, 1809. CrossRef
4. D. J. Ramón and M. Yus, Angew. Chem. Int. Ed., 2005, 44, 1602. CrossRef
5. H. Mandai, S. Irie, K. Mitsudo, and S. Suga, Molecules, 2011, 16, 8815 and references cited therein. CrossRef
6. (a) K.-S. Jeong, S.-H. Kim, H.-J. Park, K.-J. Chang, and K. S. Kim, Chem. Lett., 2002, 31, 1114; CrossRef (b) S. Yamada, T. Misono, and Y. Iwai, Tetrahedron Lett., 2005, 46, 2239; CrossRef (c) C. O. Dalaigh, S. J. Hynes, D. J. Maher, and S. J. Connon, Org. Biomol. Chem., 2005, 3, 981; CrossRef (d) S. A. Shaw, P. Aleman, and E. Vedejs, J. Am. Chem. Soc., 2003, 125, 13368; CrossRef (e) T. A. Duffey, S. A. Shaw, and E. Vedejs, J. Am. Chem. Soc., 2008, 131, 14; CrossRef (f) A. C. Spivey, T. Fekner, S. E. Spey, and H. Adams, J. Org. Chem., 1999, 64, 9430; CrossRef (g) A. C. Spivey, T. Fekner, and H. Adams, Tetrahedron Lett., 1998, 39, 8919. CrossRef
7. The major diastereomer of Ugi product has a R configuration at the newly formed stereogenic center.
8. (S,S)-Catalyst 4a was obtained by purification of a mixture of deastereomers 4a (92:8 dr) by column chromatography on SiO2 (EtOAc/toluene = 4/1, v/v).
9. A mixture of diastereomers (63:37 dr) was used in the reaction because diastereometically pure isomer could not be obtained by column chromatography on SiO2.
10. When the reaction was carried out with the catalyst (S,R)-4a under identical conditions, the s-factor was 2.1. Therefore, (S,S)-4a was a more effective catalyst than (S,R)-4a.
11. For an example of a chiral ligand prepared by the diastereoselective Ugi reaction, see: G. Dyker, K. Breitenstein, and G. Henkel, Tetrahedron: Asymmetry, 2002, 13, 1929. CrossRef
12. W. L. F. Armarego and C. L. L. Chai, Purification of Laboratory Chemicals, fifth ed.; Butterworth Heinemann, 2003.
13. R. Chênevert, N. Pelchat, and P. Morin, Tetrahedron: Asymmetry, 2009, 20, 1191. CrossRef
14. M. Päiviö, D. Mavrynsky, R. Leino, and L. T. Kanerva, Eur. J. Org. Chem., 2011, 1452. CrossRef
15. H. Kim, Y. K. Choi, J. Lee, E. Lee, J. Park, and M.-J. Kim, Angew. Chem. Int. Ed., 2011, 50, 10944. CrossRef
16. U. Kazmaier and F. L. Zumpe, Eur. J. Org. Chem., 2001, 4067. CrossRef