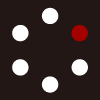
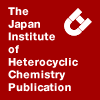
HETEROCYCLES
An International Journal for Reviews and Communications in Heterocyclic ChemistryWeb Edition ISSN: 1881-0942
Published online by The Japan Institute of Heterocyclic Chemistry
e-Journal
Full Text HTML
Received, 13th November, 2012, Accepted, 28th November, 2012, Published online, 6th December, 2012.
DOI: 10.3987/COM-12-12627
■ Cyclocondensation Reaction of Mesoionic 4-Trifluoroacetyl-1,3-oxazolium-5-olates with Hydroxylamine affording 6-Trifluoromethyl-5,6-dihydro-4H-1,2,4-oxadiazin-6-ols
Ryosuke Saijo, Ken-ichi Kurihara, Kazuki Akira, and Masami Kawase*
Faculty of Pharmaceutical Sciences, Matsuyama University, 4-2 Bunkyo-cho, Matsuyama, Ehime 790-8578, Japan
Abstract
Mesoionic 4-trifluoroacetyl-1,3-oxazolium-5-olates (1) undergo tandem addition of hydroxylamine to afford 6-trifluoromethyl-1,2,4-oxadiazin-6-ols (3) in high yields.INTRODUCTION
Trifluoromethyl-substituted heterocyclic compounds continue to receive much attention since many of them sometimes exhibit unique chemical, physiological or physical properties.1 Therefore, development of new efficient methodologies for the preparation of fluorinated heterocycles is strongly required. One of the most attractive methods for the construction of these heterocyles is based on the use of easily available fluorine-containing building blocks.2 Recently, we have focused on mesoionic 4-trifluoroacetyl-1,3-oxazolium-5-olates (1) which were easily prepared from N-acyl-N-alkylglycines (2) in a one step through the cyclodehydration by trifluoroacetic anhydride followed by trifluoroacetylation at C-4 position of an intermediary mesoionic 1,3-oxazolium-5-olate (Eq. 1).3
These trifluoroacetylated mesoionic oxazoles (1) represent a very reactive system owing to the presence of the electrophilic carbon atoms at C-2, C-5 and trifluoroacetyl group. Therefore, the rich reactivity of 1 can be expected to enable a wide variety of transformation, which makes 1 extremely useful synthons for trifluoromethyl-substituted heterocycles such as imidazoles, pyrazoles, triazines, and pyrroles.3 In line with this continuing interest, we report the reaction of the mesoionic oxazoles (1) with hydroxylamine leading to the formation of 6-trifluoromethyl-1,2,4-oxadiazines in excellent yields. Thus, with the nucleophile, the tandem addition to the C-2 position of the mesoionic ring and to the trifluoromethyl ketone yielded 6-trifluoromethyl-1,2,4-oxadiazines (3).
RESULTS AND DISCUSSION
Table 1 shows the results when 4-trifluoroacetyl-1,3-oxazolium-5-olate (1a) was allowed to react with hydroxylamine under various conditions. The best result was obtained by the reaction of 1a (1 mmol) with hydroxylamine hydrochloride (1.5 mmol) in DMF (5 mL) in the presence of sodium acetate (3 mmol) at 80 °C for 3 h: 6-trifluoromethyl-1,2,4-oxadiazine 3a was isolated in 95% yield (entry 1). The effect of the base on the yield of 3a was briefly investigated (entries 1, 4, and 7).
Sodium acetate gave the best yield of 3a in DMF as a solvent (entry 1). The reaction of 1a with hydroxylamine could proceed in other solvents, such as toluene and 1,2-dichloroethane, but the conversion was very low. Sometimes, the side product oxime (4) was isolated in low yields. The oxime (4) was identical with an authentic sample prepared in 74% yield by the reaction of N-methyl-N-(3,3,3-trifluoro-2,2-dihydroxypropyl)benzamide (5),4 which was obtained by the hydrolysis of 1a, with hydroxylamine in pyridine-EtOH (Eq. 2).
With the optimized conditions in hand, the scope of the reaction substrates was investigated. The results are summarized in Table 2. The reaction is efficient in both 3-alkyl- and 3-aryl-substituted mesoionic compounds (entries 1, 2, 4, and 5). However, 2-methyl-substituted mesoionic compounds (1b and 1d) gave slightly lower yields compared to 2-aryl-substituted compounds (1a and 1e). 2-tert-Butyl-substituted mesoionic compounds (1c and 1f) also gave the desired 1,2,4-oxadiazines (entries 3 and 6).
The structures of 3a-f are supported by spectral and analytical data. The presence of the CF3 group in 3a-f was determined on the basis of long-range 13C-19F coupling. Thus, the carbons of the CF3 group and C-6 appear at around δ 122.5 ppm (quartet, 1JC-F = 287 Hz) and δ 90.6 ppm (quartet, 2JC-F = 32 Hz), respectively. The 1H-NMR spectrum of 3a exhibited the methylene signal of C-5 at δ 3.25 ppm (d, 1H, J = 12 Hz) and 3.37 ppm (d, 1H, J = 12 Hz). These 1H- and 13C-NMR data are similar to the data for the 6-trifluoromethyl-1,4,5,6-tetrahydro-4-methyl-1,3-diphenyl-1,2,4-triazin-6-ol (6),3a 5,5-dimethyl-3-phenyl-1,2,4-oxadiazin-6-ol (7)5 and 6-methyl-3-phenyl-1,2,4-oxadiazine (8)6 as shown in Scheme 1.
A plausible mechanism is described in Scheme 2. Thus, nucleophilic attack of the nitrogen of hydroxylamine on C-2 of 1 gives rise to an adduct (9). The scission of the bond of C-2 and O-1 in 10 gives an open-chain intermediate (11), which extrudes carbon dioxide to provide the ketone (12). Finally, intramolecular cyclization of 12 affords 3.
The 1,2,4-oxadiazine compounds possess interesting pharmacological properties such as diuretic, antiphlogistic, peripheral vasodilative, coronary flow increasing, and hypotensive effects.7 However, the 1,2,4-oxadiazine core belongs to an underutilized class of heterocycles and there are only a handful of methods for the synthesis of 5,6-dihydro-4H -1,2,4-oxadiazines: (a) condensation of arylamidoximes and glyoxal,8 chloroacetyl chloride,9 ethyl γ-bromoacetoacetate,10 or 1,2-diaza-1,3-butadienes,11 (b) reaction of oxazolines and hydroxylamine,5 and (c) acid-catalyzed isomerization of aziridin-1-yl oximes.6,12 In spite of the importance of fluorine molecules, 1,2,4-oxadiazines containing trifluoromethyl substituent have not yet been described.
In summary, we developed the first efficient and regiospecific preparation of 6-trifluoromethyl-5,6-dihydro-4H-1,2,4-oxadiazines. The method appears to be useful and convenient in terms of the ready accessibility of the starting materials, cheap reagents, operational simplicity, and high overall yields.
EXPERIMENTAL
All melting points were determined using a Yanagimoto hot-stage melting point apparatus and are uncorrected. 1H-NMR spectra were measured on Bruker AVANCE500 spectrometer with tetramethylsilane (Me4Si) as an internal reference and CDCl3 as the solvent. 13C-NMR spectra were obtained on a Bruker AVANCE500 spectrometer (at 126 MHz). Both 1H- and 13C-NMR spectral data are reported in parts per million (δ) relative to Me4Si. Infrared (IR) spectra were recorded on a JASCO FT/IR-4100 spectrometer. Low- and high-resolution MS were obtained with a JEOL JMS-GC mate Ⅱ spectrometer with a direct inlet system at 70 eV. Elemental analyses were carried out in the microanalytical laboratory of Ehime University. Standard work-up means that the organic layers were finally dried over Na2SO4, filtered, and concentrated in vacuo below 45 °C using a rotary evaporator.
Materials: The following compounds were prepared by employing the reported method. N-Benzoyl-N-methylglycine. mp 101−104 °C (lit.,13 mp 102−104 °C). N-Acetyl-N-benzylglycine. mp 118−119 °C (lit.,14 mp 118−119 °C). N-Phenyl-N-pivaloylglycine. mp 123−124 °C. 1H NMR (500 MHz, CDCl3) δ 1.05 (s, 9H, CCH3), 4.27 (s, 2H, NCH2), 7.36-7.40 (m, 5H, ArH). 13C NMR (126 MHz, CDCl3) δ 29.2, 40.7, 55.1, 128.6, 129.3, 129.5, 143.8, 174.1, 178.7. N-Acetyl-N-phenylglycine. mp 196−198 °C (mp15 193−195 °C). N-Benzoyl-N-phenylglycine. mp 126−128 °C (lit.,13 mp 127−129 °C). N-Methyl-N-pivaloylglycine. mp 75−76 °C (lit.,4 mp 75−76 °C).
General Procedure for Preparation of 4-Trifluoroacetyl-1,3-oxazolium-5-olates (1): To a stirred suspension of N-acyl-N-alkylglycine (5.2 mmol) in AcOEt (10 mL) was added TFAA (2.2 mL, 15.6 mmol) at 0 °C, and the solution was stirred at rt for 3 h. To the mixture was added hexane, and the precipitate was collected and recrystallized from hexane/AcOEt to give the product 1.
4-Trifluoroacetyl-3-methyl-2-phenyl-1,3-oxazolium-5-olate (1a). Pale yellow crystals, 87% yield. mp 161−163 °C (lit.,16 mp 162−163 °C).
3-Benzyl-4-trifluoroacetyl-2-methyl-1,3-oxazolium-5-olate (1b).3a White crystals, 67% yield. mp 143−144 °C. HRMS (EI) for C13H10F3NO3 (M+): Calcd, 285.0613. Found, 258.0626.
2-tert-Butyl-4-trifluoroacetyl-3-phenyl-1,3-oxazolium-5-olate (1c). Yellow crystals, 60% yield. mp 174−175 °C. IR (KBr) νmax: 2985, 1880, 1639, 1551, 1357, 1259, 1201, 1148, 831, 779, 734, 705 cm-1. 1H NMR (500 MHz, CDCl3) δ 1.23 (s, 9H, CCH3), 7.35–7.37 (m, 2H, ArH), 7.53–7.62 (m, 3H, ArH). 13C NMR (126 MHz, CDCl3) δ 28.5 (CCH3), 36.8 (CCH3), 97.4, 116.6 (q, 1JC-F = 289.4 Hz, CF3), 126.9, 129.5, 131.1, 134.3, 157.5, 163.3, 166.1 (q, 2JC-F = 37.3 Hz, CCF3). MS m/z: 313 (M+, 66), 58 (100). Anal. Calcd for C15H14F3NO3: C, 57.51; H, 4.50; N, 4.47. Found: C, 57.23; H, 4.62; N, 4.46.
4-Trifluoroacetyl-2-methyl-3-phenyl-1,3-oxazolium-5-olate (1d). White crystals, 90% yield. mp 200−203 °C (lit.,16 mp 211−212 °C).
4-Trifluoroacetyl-2,3-diphenyl-1,3-oxazolium-5-olate (1e). Yellow crystals, 81% yield. mp 194−196 °C (lit.,16 mp 194−196 °C).
2-tert-Butyl-4-trifluoroacetyl-3-methyl-1,3-oxazolium-5-olate (1f). White crystals, 67% yield. mp 120−121 °C (lit.,4 mp 120−121 °C).
General Procedure for Synthesis of 6-Trifluoromethyl-1,2,4-oxadiazin-6-ols (3): A mixture of hydroxylamine hydrochloride (104 mg, 1.50 mmol) and sodium acetate (246 mg, 3.00 mmol) in DMF (5 mL) was stirred at 0 °C for 10 min under atmosphere of argon. To the mixture was added 4-trifluoroacetyl-1,3-oxazolium-5-olate 1 (1.00 mmol), and the whole was stirred at 80 °C for an additional several hours. After workup with 10% aqueous Na2CO3, the mixture was extracted with AcOEt (x 3). The combined organic layers were washed with brine, dried over anhyd Na2SO4, and evaporated. The residue was purified by column chromatography (silica gel, hexane:AcOEt = 2:1) to give the product 3.
6-Trifluoromethyl-5,6-dihydro-4-methyl-3-phenyl-4H-1,2,4-oxadiazin-6-ol (3a). White crystals, 95% yield. mp 163−164 °C (CHCl3-hexane). IR (KBr) νmax: 3032, 1602, 1577, 1413, 1368, 1335, 1272, 1200, 1051, 1024, 979, 770, 704 cm-1. 1H NMR (500 MHz, CDCl3) δ 2.86 (s, 3H, NCH3), 3.37 (d, J = 11.8 Hz, 1H, NCH), 3.52 (d, J = 11.7 Hz, 1H, NCH), 4.85 (br s, 1H, OH), 7.41-7.48 (m, 5H, ArH) ppm. 13C NMR (126 MHz, DMSO-d6) δ 39.9 (NCH3), 48.4 (NCH2), 90.6 (q, 2JC-F = 31.7 Hz, CF3C), 122.5 (q, 1JC-F = 287.3 Hz, CF3), 128.4, 128.7, 129.8, 131.5, 154.7 (CN) ppm. MS m/z: 260 (M+, 100). Anal. Calcd for C11H11F3N2O2: C, 50.77; H, 4.26; N, 10.77. Found: C, 50.63; H, 4.01; N, 10.70.
4-Benzyl-6-trifluoromethyl-5,6-dihydro-3-methyl-4H-1,2,4-oxadiazin-6-ol (3b). White crystals, 68% yield. mp 187−189 °C (CHCl3-hexane). IR (KBr) νmax: 3040, 1614, 1207, 1190 cm-1. 1H NMR (500 MHz, CDCl3) δ 2.08 (s, 3H, CH3), 3.20 (d, J = 11.4 Hz, 1H, NCH), 3.39 (d, J = 11.5 Hz, 1H, NCH), 4.37 (d, J = 16.5 Hz, 1H, PhCH), 4.62 (d, J = 16.6 Hz, 1H, PhCH), 6.04 (br s, 1H, OH), 7.26-7.27 (m, 2H, ArH), 7.32-7.34 (m, 1H, ArH), 7.38-7.41 (m, 2H, ArH) ppm. 13C NMR (126 MHz, CDCl3) δ 16.6 (CH3), 47.0, 54.4, 90.9 (q, 2JC-F = 32.7 Hz, CCF3), 122.1 (q, 1JC-F = 284.9 Hz, CF3), 126.6, 128.0, 129.2, 135.5, 152.4 (CN) ppm. MS m/z: 274 (M+, 100). Anal. Calcd for C12H13F3N2O2: C, 52.56; H, 4.78; N, 10.21. Found: C, 52.22; H, 4.52; N, 10.05.
3-tert-Butyl-6-trifluoromethyl-5,6-dihydro-4-phenyl-4H-1,2,4-oxadiazin-6-ol (3c). White crystals, 97% yield. mp 156−158 °C (CHCl3-hexane). IR (KBr) νmax: 3161, 2993, 2962, 1562, 1214, 1181, 1145 cm-1. 1H NMR (500 MHz, CDCl3) δ 1.12 (s, 9H, CCH3), 3.33 (br s, 1H, OH), 3.54 (d, J = 12.5 Hz, 1H, NCH), 3.58 (d, J = 12.4 Hz, 1H, NCH), 7.27-7.30 (m, 3H, ArH), 7.35-7.38 (m, 2H, ArH) ppm. 13C NMR (126 MHz, CDCl3) δ 30.2 (CCH3), 38.7 (CCH3), 51.6 (NCH3), 92.2 (q, 2JC-F = 32.8 Hz, CCF3), 121.8 (q, 1JC-F = 286.4 Hz, CF3), 127.5, 128.8, 129.2, 146.2, 160.3 (CN) ppm. MS m/z: 302 (M+, 12), 252 (100). Anal. Calcd for C14H17F3N2O2: C, 55.62; H, 5.67; N, 9.27. Found: C, 55.32; H, 5.74; N, 9.26.
6-Trifluoromethyl-5,6-dihydro-3-methyl-4-phenyl-4H-1,2,4-oxadiazin-6-ol (3d). White crystals, 54% yield. mp 169−170 °C (CHCl3-hexane). IR (KBr) νmax: 3071, 3043, 3025, 1616, 1593, 1200, 1179, 1158 cm-1. 1H NMR (500 MHz, CDCl3) δ 1.81 (s, 3H, CH3), 3.58 (d, J =11.6 Hz, 1H, NCH) , 3.76 (d, J = 11.6 Hz, 1H, NCH), 6.25 (br s, 1H, OH), 7.26-7.29 (m, 2H, ArH), 7.35 (m, 1H, ArH), 7.41-7.43 (m, 2H, ArH) ppm. 13C NMR (126 MHz, CDCl3) δ 17.5 (CH3), 50.8 (NCH2), 90.6 (q, 2JC-F = 32.7 Hz, CCF3), 122.2 (q, 1JC-F = 286.2 Hz, CF3), 127.9, 128.2, 129.9, 142.2, 151.7 (CN) ppm. MS m/z: 260 (M+, 100). Anal. Calcd for C11H11F3N2O2: C, 50.77; H, 4.26; N, 10.77. Found: C, 50.61; H, 4.18; N, 10.74.
6-Trifluoromethyl-5,6-dihydro-3,4-diphenyl-4H-1,2,4-oxadiazin-6-ol (3e). White crystals, 88% yield. mp 186−187 °C (CHCl3-hexane). IR (KBr) νmax: 3054, 1590, 1552, 1494, 1359, 1304, 1233, 1203, 1189, 1146, 1088, 1065, 985, 768, 694 cm-1. 1H NMR (500 MHz, CDCl3) δ 3.88 (d, J = 12.0 Hz, 1H, NCH), 3.96 (d, J = 12.0 Hz, 1H, NCH), 4.09 (br s, 1H, OH), 6.94 (d, J = 8.0 Hz, 2H, ArH), 7.06 (t, J = 7.4 Hz, 1H, ArH), 7.15-7.29 (m, 5H, ArH), 7.38 (d, J = 8.5 Hz, 2H, ArH) ppm. 13C NMR (126 MHz, CDCl3) δ 49.8 (NCH2), 92.4 (q, 2JC-F = 33.0 Hz, CF3C), 121.8 (q, 1JC-F = 286.5 Hz, CF3), 125.5, 128.2, 129.1, 129.9, 131.1, 144.8, 153.6 (CN) ppm. MS m/z: 322 (M+, 100). Anal. Calcd for C16H13F3N2O2: C, 59.63; H, 4.07; N, 8.69. Found: C, 59.59; H, 3.84; N, 8.72.
3-tert-Butyl-6-trifluoromethyl-5,6-tetrahydro-4-methyl-4H-1,2,4-oxadiazin-6-ol (3f). White crystals, 74% yield. mp 99−101 °C (CHCl3-hexane). IR (KBr) νmax: 3088, 2996, 1583, 1323, 1185, 1162, 1126, 991 cm-1. 1H NMR (500 MHz, CDCl3) δ 1.32 (s, 9H, CCH3), 3.16 (s, 3H, NCH3), 3.19 (d, J = 11.9 Hz, 1H, NCH), 3.36 (d, J = 11.9 Hz, 1H, NCH), 3.79 (br s, 1H, OH) ppm. 13C NMR (126 MHz, CDCl3) δ 28.8 (CCH3), 36.8 (CCH3), 41.5 (NCH3), 50.7 (NCH2), 90.9 (q, 2JC-F = 32.7 Hz, CCF3), 122.1 (q, 1JC-F = 286.3 Hz, CF3), 160.2 (CN) ppm. MS m/z: 313 (M+, 66), 58 (100). Anal. Calcd for C9H15F3N2O2: C, 45.00; H, 6.29; N, 11.66. Found: C, 44.71; H, 6.19; N, 11.79.
N-[3,3,3-Trifluoro-2-(hydroxyimino)propyl]-N-methylbenzamide (4). Pale yellow crystals. mp 139−141 °C (CHCl3-hexane). IR (KBr) νmax: 2878, 2806, 1598, 1569, 1486, 1409, 1357, 1278, 1189, 1132, 1075, 1007, 736, 708 cm-1. 1H NMR (500 MHz, DMSO-d6) δ 2.86 (s, 3H, CH3), 4.40 (br s, 1H, NCH), 4.60 (br s, 1H, NCH), 7.43-7.46 (m, 5H, ArH), 12.89 (br s, 1H, OH) ppm. 13C NMR (126 MHz, CDCl3) δ 37.5 (NCH3), 121.1 (q, 1JC-F = 273.3 Hz, CF3), 126.6, 128.4, 129.6, 135.6, 143.5 (q, 2JC-F = 29.8 Hz, CCF3), 170.3 (CO) ppm. MS m/z: 260 (M+, 32), 105 (100). Anal. Calcd for C11H11F3N2O2: C, 55.62; H, 5.67; N, 9.27. Found: C, 55.32; H, 5.74; N, 9.26.
The authentic 4 was prepared by the following method: A mixture of N-methyl-N-(3,3,3-trifluoro-2,2-dihydroxypropyl)benzamide4 (263 mg, 1 mmol), hydroxylamine hydrochloride (104 mg, 1.50 mmol) and pyridine (0.25 mL) in EtOH (5 mL) was stirred at 0 °C for 10 min under atmosphere of argon. Then, the whole was stirred at 90 °C for 3 h. After workup with 10% aqueous Na2CO3, the mixture was extracted with AcOEt (x 3). The combined organic layers were washed with brine, dried over anhyd Na2SO4, and evaporated. The residue was purified by column chromatography (silica gel, hexane:AcOEt=1:2) to give N-methyl-N-(3,3,3-trifluoro-2-(hydroxyimino)- propyl)benzamide (4) (195.0 mg, 74%).
References
1. V. A. Petrov, ‘Fluorinated Heterocyclic Compounds-Synthesis, Chemistry, and Applications,’ John Wiley & Sons, Inc., Hoboken, New Jersey, 2009. CrossRef
2. T. Hiyama, ‘Organofluorine Building Blocks. In Organofluorine Compounds: Chemistry and Applications,’ Springer-Verlag, Berlin, 2000, pp. 77-118; M. Schlosser, Angew. Chem. Int. Ed., 2006, 45, 2; CrossRef J.-P. Begue and D. Bonnet-Delpon, ‘Bioorganic and Medicinal Chemistry of Fluorine,’ John Wiley & Sons, Inc., Hoboken, New Jersey, 2008. CrossRef
3. (a) M. Kawase and H. Koiwai, Chem. Pharm. Bull., 2008, 56, 433; CrossRef (b) R. Saijo, Y. Hagimoto, and M. Kawase, Org. Lett., 2010, 12, 4776; CrossRef (c) R. Saijo and M. Kawase, Tetrahedron Lett., 2012, 53, 2782. CrossRef
4. M. Kawase and S. Saito, Chem. Pharm. Bull., 2000, 48, 410. CrossRef
5. A. Bigot, J. Blythe, C. Pandya, T. Wagner, and O. Loiseleur, Org. Lett., 2011, 13, 192. CrossRef
6. S. Y. Cho, S. K. Kang, J. H. Ahn, J. D. Ha, and J.-K. Choi, Tetrahedron Lett., 2006, 47, 9029. CrossRef
7. J. Mezei, S. Kuttel, L. Szemere, and I. Racz, Pharmazie, 1984, 39, 399; J. Mezei, S. Kuttel, and I. Racz, Polish J. Pharmacol. Pharm., 1984, 36, 397; K. Takacs and K. Harsanyi, Chem. Ber., 1970, 103, 2330; CrossRef K. Takacs, K. Harsanyi, P. Kolonits, and K. I. Ajzert, Chem. Ber., 1975, 108, 1911; CrossRef A. J. Crovetti, A. M. VonEsch, and R. J. Thill, J. Heterocycl. Chem., 1972, 9, 435. CrossRef
8. R. M. Srivastava, L. P. F. Morais, S. C. Melo Souto, G. B. Carpenter, and L. T. Carvalho, Tetrahedron Lett., 2006, 47, 3173. CrossRef
9. A. Q. Hussein, Heterocycles, 1987, 26, 163. CrossRef
10. K. Tabei, E. Kawashima, T. Takada, and T. Kato, Chem. Pharm. Bull., 1982, 30, 3987. CrossRef
11. O. A. Attanasi, L. Cotarca, G. Favi, P. Filippone, F. R. Perrulli, and S. Santeusanio, Synlett, 2009, 10, 1583. CrossRef
12. P. Rajagopalan and C. N. Talaty, J. Am. Chem. Soc., 1966, 88, 5048. CrossRef
13. J. Deruiter, B. E. Swearingen, V. Wandreker, and C. A. Mayfield, J. Med. Chem., 1991, 34, 2120. CrossRef
14. M. Kawase, S. Saito, and T. Kurihara, Chem. Pharm. Bull., 2001, 49, 461. CrossRef
15. J. DeRuiter, B. E. Swearingen, V. Wandrekar, and C. A. Mayfield, J. Med. Chem., 1989, 32, 1033. CrossRef
16. C. V. Greco, R. P. Gray, and V. G. Grosso, J. Org. Chem., 1967, 32, 4101. CrossRef