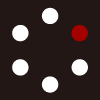
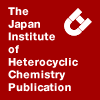
HETEROCYCLES
An International Journal for Reviews and Communications in Heterocyclic ChemistryWeb Edition ISSN: 1881-0942
Published online by The Japan Institute of Heterocyclic Chemistry
e-Journal
Full Text HTML
Received, 27th November, 2012, Accepted, 21st January, 2013, Published online, 31st January, 2013.
■ Novel Synthesis of Thioalkoxy-Substituted Oxa[9]helicenes by the Reaction of Helical Quinones with Thiols
Mohammad Salim, Takao Kimura, and Michinori Karikomi*
Department of Material and Environmental Chemistry, Faculty of Engineering, Utsunomiya University, 7-1-2, Yoto, Utsunomiya, Tochigi 321-8585, Japan
Abstract
The reaction of helical quinone with thiols in the presence of Brønsted acid such as HCl, para-toluene sulfonic acid (pTSA), Yb(OTf)3·3H2O or BF3·OEt2 to form resulted in the formation of thioalkoxy-substituted oxa[9]helicenes in moderate to good yields.Helicenes and other related helical compounds captured the interest of researchers, owing to their potential for use as effective chiral sources in asymmetric synthesis, chiral materials, and optical materials as a result of this potential arises from their inherent asymmetric molecular structures. In the past decade, a large number of helicenes and other related molecules such as aza- and thia-helicenes have been developed because of their extraordinary and unique properties. Thus, the synthesis of helicene-like compounds has become a subject of increasing interest to synthetic organic chemists.1 Among the several synthetic strategy for the helical polycondensed structure, photo cyclization of stilbene derivatives or styrylheterocycles has emerged as one of the most powerful and attractive methodologies. Oxahelicenes are also possible to synthesize according to the same methodology. However, only a few
examples of oxahelicenes have been reported. In this context, the development of new synthetic approaches to synthesize oxygen-containing helical molecules represents an important opportunity to develop an application of these new, potential and useful molecules. We have previously reported the synthesis of the helical quinones 1 derived from 2-hydroxybenzo[c]phenanthrenes via a stereo- and region-selective oxidative coupling using CuCl(OH)–TMEDA.2 Furthermore, we studies the synthetic uses of the quinones 1 for the construction of new helical molecules. Then, we have reported on synthesis of oxa[9]helicenes from the helical quinones 1 with Lawesson’s reagent or phosphorous pentasulfide.3 We also have reported on synthesis of alkoxy substituted oxa[9]helicenes by the reaction of the quinone 1 with several alcohols.4 According to the results, the reaction of the quinone with primary alcohols and secondary alcohols gave alkoxy-substituted oxa[9]helicenes 2 in good yields. However, the cases of reactions involving tertiary alcohol, such as t-BuOH did not give any products.4 Thus, as an extension of these results, we wish to report herein the novel synthesis of thioalkoxy oxa[9]helicenes from the helical quinone with various thiols. We initially examined the reaction of 1 and n-butylthiol as a nucleophile. Table 1 shows the reaction of helical quinone 1 with series of structurally different thiols in the presence of acids (HCl, pTSA, Yb(OTf)3·3H2O). Most of cases a mixture of desired 9-thioalkoxy-11- oxa[9]helicene 2a-h and undesired non-substituted 11-oxa[9]helicene 3 was obtained in good yield.5,6 Similar to the case of n-butylthiol, n-dodecanethiol also gave the corresponding 2b in moderate selectivity (entry 4). The reaction of secondary thiols such as i-PrSH and cyclohexylthiol afforded the corresponding thioalkoxy substituted oxa[9]helicene 2c-d more selectively compare to the cases of primary thiols (entries 5 and 6). Interestingly, the reactions of tertiary thiols such as t-BuSH and tert-tetradecanethiol gave thioalkoxy substituted 2e-f selectively in good yield. In these cases, the formation of 3 was not detected (entries 7 and 8). Aryl thiols were also reacted to give the corresponding aryl thioalkoxy substituted oxa[9]helicene 2g-h with moderate selectivity (entries 9, 10, and 11).
These results would suggest a convincing reaction mechanism for the synthesis of 2 (Scheme 2). The initial conjugate addition and the following cyclization are catalyzed by Brønsted acid as a common process for both 2 and 3. The selectivity for the substituted 2 or non-substituted 3 is realized at the final elimination step; the selectivity is controlled by the steric hindrance of the thiols. The less hindered primary and secondary thiols are possible to form disulfide as an eliminating species (route b). Thus, the product selectivity for 2 (route a) is unsatisfactory. On the other hand, in the cases of tertiary thiols are too hindered to form the corresponding disulfide. Thus, tertiary thioalkoxy substituted oxa[9]helicenes 2e-f were obtained selectively. Perhaps the most important pieces of experimental evidence to support for this reaction mechanism is the fact that disulfide was detected in the reaction mixture. When cyclohexylthiol was used, cyclohexyl disulfide was detected in the reaction mixture by the comparison of its 1H NMR and 13C NMR to the commercially available authentic sample.
In conclusion, this paper describes a novel synthetic route for 9-thioalkoxy-substituted 11-oxa[9]helicene derivatives 2 using Brønsted acids; the synthesis is catalyzed by the nucleophilic addition of various thiols and subsequent cyclization and dehydration reactions. Unfortunately, there is a competing side reaction for non-substituted 11-oxa[9]helicene 3. We then revealed that the selectivity is considerably controlled by the steric hindrance of thiols. In the cases of tertiary thiols, the completed selectivity for the thioalkoxy substituted oxa[9]helicenes 2 were obtained was shown. Further studies aimed at obtaining substituted oxa[9]helicenes by various nucleophiles are currently underway in our laboratory.
ACKNOWLEDGEMENTS
The authors wish to thank Mr. M. Roppongi of the Collaboration Center for Research & Development, Utsunomiya University, for MS spectral data.
References
1. For review (a) A. Urbano, Angew. Chem., Int. Ed., 2003, 42, 3986; CrossRef (b) Y. Shen and C.-F. Chen, Chem. Rev., 2012, 112, 1463. CrossRef
2. M. Karikomi, M. Yamada, Y. Ogawa, H. Houjou, K. Seki, K. Hiratani, K. Haga, and T. Uyehara, Tetrahedron Lett., 2005, 46, 5867. CrossRef
3. M. Salim, A. Akutsu, T. Kimura, M. Minabe, and M. Karikomi, Tetrahedron Lett., 2011, 52, 4518. CrossRef
4. M. Salim, H. Ubukata, T. Kimura, and M. Karikomi, Tetrahedron Lett., 2011, 52, 6591. CrossRef
5. General procedure for the synthesis of 2: Quinone 1 (0.05 mmol) was added with thiol (2-3 eq) in chloroform (1 mL) in the presence of conc. HCl (4 eq) at room temperature for 0.5-1 h. The mixture was neutralized by 5% NaOH, washed with brine and extracted with chloroform (3 × 10 mL). The organic layer was dried (Na2SO4), filtered, and concentrated under vacuum. The crude product was purified by column chromatography on silica gel (chloroform) to give a mixture of 2 and 3. The mixture was separated by recrystallization with ethyl acetate.
6. Compound characterization: 2a. 1H NMR (500 MHz, CDCl3, δ/ppm): 8.63 (1H, d, J = 8.5 Hz), 8.35 (1H, s), 8.28 (1H, d, J = 8.5 Hz), 8.23 (1H, d, J = 8.0 Hz), 7.98 (1H, d, J = 8.0 Hz), 7.62 (1H, d, J = 8.5 Hz), 7.59 (1H, d, J = 8.5 Hz), 7.58 (1H, d, J = 8.0 Hz), 7.54 (1H, d, J = 8.5 Hz), 7.40 (1H, d, J = 8.5 Hz), 7.38 (1H, d, J = 8.5 Hz), 7.33 (1H, d, J = 7.5 Hz), 7.28 (1H, d, J = 8.0 Hz), 6.79 (1H, t, J = 7.5 Hz), 6.74 (1H, t, J = 7.0 Hz), 6.22 (1H, d, J = 9.0 Hz), 6.18 (1H, d, J = 8.0 Hz), 5.75 (1H, t, J = 8.0 Hz), 5.72 (1H, t, J = 7.0 Hz), 3.19-3.29 (2H, m), 1.79-1.85 (2H, m), 1.55-1.62 (2H, m), 0.98 (3H, t, J =7.0 Hz), 13C NMR (125 MHz, CDCl3, δ /ppm): 154.04, 153.53, 134.24, 129.94, 129.86, 129.56, 129.52, 129.49, 128.97, 127.59, 127.27, 127.25, 127.19, 126.99, 126.54, 126.51, 126.35, 126.24, 126.05, 125.55, 125.37, 125.33, 125.13, 125.04, 124.63, 124.37, 124.18, 124.15, 124.03, 122.85, 122.31, 122.29, 121, 120.03, 112.24, 110.87, 34.94, 31.21, 22.13, 13.76, HRMS (EI) calcd for C40H28OS [M]+ 556.1861, found 556.1861.