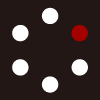
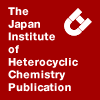
HETEROCYCLES
An International Journal for Reviews and Communications in Heterocyclic ChemistryWeb Edition ISSN: 1881-0942
Published online by The Japan Institute of Heterocyclic Chemistry
e-Journal
Full Text HTML
Received, 4th June, 2013, Accepted, 8th July, 2013, Published online, 11th July, 2013.
DOI: 10.3987/COM-13-S(S)24
■ Synthesis, Properties, and Crystal Structure of DDQ-Adducts of Ethynylated 2H-Cyclohepta[b]furan-2-ones
Taku Shoji,* Mitsuhisa Maruyama, Erika Shimomura, Akifumi Maruyama, Shunji Ito, Masafumi Yasunami, Junya Higashi, Kozo Toyota, and Noboru Morita*
Department of Chemistry, Faculty of Science, Shinshu University, Asahi, Matsumoto 390-8621, Japan
Abstract
Ethynylated 2H-cyclohepta[b]furan-2-ones reacted with 2,3-dichrolo-5,6-dicyano-1,4-benzoquinone (DDQ) in a formal [2+2] cycloaddition reaction to afford the corresponding DDQ-adducts in good yields. The electronic properties of the DDQ-adducts were investigated by UV/Vis spectroscopy. One of the DDQ-adducts was revealed the molecular structure by X-ray crystallographic analysis. The redox behavior of the new compounds was examined by cyclic voltammetry (CV) and differential pulse voltammetry (DPV).INTRODUCTION
2,3-Dichloro-5,6-dicyano-1,4-benzoquinone (DDQ) is well known as an oxidizing reagent for the formal dehydrogenation reaction in organic synthesis.1 Recently, Diederich2 and Trofimov3 have reported that DDQ is reacted with various alkyne derivatives in a formal [2+2] cycloaddition reaction to give the corresponding cycloaddition products. They have also reported the unusual reactivities and properties of the adducts, such as carbon-skeleton rearrangement,2,4 intramolecular charge-transfer absorption, third-order optical nonlinearities,2 and so on. These results show the DQQ-adducts have a potential as a new series of advanced organic materials.
2H-Cyclohepta[b]furan-2-one is known as a heteroazulene, a versatile precursor for azulene derivatives.5 Although its unusual reactivity has already been revealed by many research groups,6 there are few 2H-cyclohepta[b]furan-2-one derivatives for the application to the advanced materials with potentially useful electronic properties. For the extension of this chemistry, we have focused our studies on the synthesis of tetracyanobutadiene (TCBD)7 and dicyanoquinodimethane (DCNQ)8 derivatives of 2H-cyclohepta[b]furan-2-one by the formal [2+2] cycloaddition–cycloreversion reaction of the corresponding alkyne derivatives with tetracyanoethylene (TCNE) and 7,7,8,8-tetracyanoquinodimethane (TCNQ), respectively. The study revealed the TCBD and DCNQ derivatives are featured by a strong ICT absorption in their UV/Vis spectra. Ethynyl-2H-cyclohepta[b]furan-2-one derivatives are also expected to show high reactivity with DDQ toward the [2+2] cycloaddition reaction to afford the novel DDQ-adducts owing to the electron-donating nature of the 2H-cyclohepta[b]furan-2-one functions.
Herein, we describe the synthesis of the DDQ-adducts 8–14 with 2H-cyclohepta[b]furan-2-one substituents by the [2+2] cycloaddition reaction of ethynyl-2H-cyclohepta[b]furan-2-ones 1–7 with DDQ. The electronic properties of the new compounds obtained by this reaction were characterized by CV, DPV, and absorption spectroscopy. In addition one of the DDQ-adducts was revealed the molecular structure by X-ray crystallographic analysis.9
RESULT AND DISCUSSION
SYNTHESIS: Ethynyl-2H-cyclohepta[b]furan-2-ones 1–7 were prepared by Sonogashira–Hagihara reaction, according to the procedure reported by us, recently. For the synthesis of the novel DDQ-adducts, we have examined the [2+2] cycloaddition reaction of the ethynyl-2H-cyclohepta[b]furan-2-ones 1–7 with DDQ, according to the procedure described in the literature. The yield of the products is summarized in Table 1.
The reaction of 1 with DDQ in refluxing ethyl acetate yielded 8 in 83% yield. In the reaction of acetylene derivatives with TCNE, the [2+2] cycloaddition reaction with an alkyne moiety afford the cyclobutene intermediate, following to the ring-opening reaction of the cyclobutene derivative gives the thermodynamically stable TCBDs. Formation of the DDQ-adducts with a cyclobutene substructure in this reaction might be attributable to the structural effect of the fused cyclohexene ring.
To investigate the substituent effect on the alkyne terminal, the reaction of 2−7 with DDQ were examined under the similar reaction conditions. Acetylene derivatives 2 and 3 also reacted readily with DDQ to afford the corresponding DDQ-adducts 9 and 10 in 70% and 84% yields, respectively, as similar to the reaction of 1 with DDQ. The cycloaddition reaction of 4 gave the presumed DDQ-adduct 11 in 15% yield along with a certain amount of an inseparable complex mixture, although the cycloaddition reaction of 5 with an electron-withdrawing function with DDQ afforded the presumed 12 in 74% yield as a sole product. These results indicate the aryl group with highly electron-donating function on the acetylene terminal should be prevented the [2+2] cycloaddition reaction with DDQ in the acetylene moiety. The [2+2] cycloaddition reaction of thiophene-substituted alkyne 6 with DDQ also afforded the corresponding DDQ-adduct 13 in 76% yield, under the similar reaction conditions. The reaction of symmetrically substituted alkyne 7 with DDQ yielded the corresponding cycloadduct 14 in 59% yield.
PROPERTIES: These new compounds 8–14 were fully characterized by the spectral data. Mass spectra of 8–14 ionized by ESI showed the correct molecular ion peaks observed as [M + Na]+ ion peaks. The characteristic stretching vibration band of the C≡N moieties of 8–14 was observed at νmax = 2219–2239 cm-1 on their IR spectra, instead of the characteristic stretching band for C≡C triple bond of the starting acetylene derivatives. Assignment of peaks in the 1H NMR spectra of the compounds was accomplished by decoupling and 2D COSY experiments. These results are consistent with the structure of these products.
Single crystals of 9 suitable for X-ray crystallographic analysis were obtained by recrystallization from CH2Cl2. The DDQ-adduct 9 was crystallized in an orthorhombic cell. The molecular structure of 9 was revealed as shown in Figure 1. The cycloheptafuranone ring in 9 has an approximately orthogonal conformation with the substituted cyclobutene ring. Recently, Diederich et al. have reported the planarity between the dimethylanilino group and the cyclobutene ring of compound 15 by X-ray crystallographic analysis (Figure 2). The structural deformation of 9 might be ascribed to the steric bulkiness of both trimethylsilyl group and isopropyl moiety substituted on the cycloheptafuranone ring. The effect may become much more serious than that of simpler dimethylanilino group in 15.
The UV/Vis spectra of compounds 10–14 showed characteristic absorptions arising from the cycloheptafuranone system in the visible region. The absorption maxima (λmax) and the coefficients (log ε) of the DDQ-adducts 10–14 and the corresponding acetylene derivatives 3−7 are summarized in Table 2. The absorption maxima of the DDQ-adduct 10 showed broad absorption bands at λmax = 418 nm and 451 (sh) nm, respectively. The UV/Vis spectrum of 11, with a p-anilino substituent, exhibited absorption bands at λmax = 376 nm and 439 nm, respectively. The absorption maximum of 12 (λmax = 463 nm) exhibited a bathochromic shift relative to those of 10 and 11. These results reflect to the p-nitrobenzene moiety results in a decrease of the HOMO-LUMO gap of 12, compared to those of 10 and 11. The DDQ-adduct 13 substituted by 2-thienyl group showed a broad absorption at around λmax = 400–600 nm in CH2Cl2. Compound 14 exhibited two absorption bands at λmax = 400 nm and λmax = 507 (sh) nm. Intensity of the absorption band was twice as large as that of 10−13. It should be ascribed to the overlapping of the transition from the two cycloheptafuranone rings in the molecule. The absorption coefficient of the DDQ-adducts 10–14 was decreased compared with that of acetylene derivatives 3−7. These results suggest the less effective conjugation between the cycloheptafuranone and aryl moieties, due to the low planarity as observed by X-ray crystallographic analysis of DDQ-adduct 9.
To clarify the electrochemical properties, the redox behavior of 10–14 was examined by CV and DPV. Measurements were carried out with a standard three-electrode configuration. Tetraethylammonium perchlorate (0.1 M) in benzonitrile was used as the supporting electrolyte with a platinum wire and disk as the auxiliary and working electrodes, respectively. All measurements were carried out under an argon atmosphere, and potentials were related to an Ag/Ag+ reference electrode and Fc/Fc+ as an internal reference, which discharged at +0.15 V. The redox potentials (in V vs. Ag/AgNO3) of DDQ-adducts 10–14 obtained by the DPV analysis are summarized in Table 3.
All DDQ-adducts 10–14 showed irreversible oxidation and reduction waves by CV. Differential pulse voltammograms for the oxidation and reduction of 14 are shown in Figure 3. DDQ-adducts with aryl substituents 10, 11, and 12 displayed an irreversible two- or three-stage reduction wave, depending on the p-substituent on the substituted-benzene ring. The first reduction potential of 12 (−0.54 V) was less negative than that of 10 (−0.59 V) and 11 (−0.92 V). These results reflect that the electron-withdrawing NO2 substituent on the benzene ring directly results in a decrease of the LUMO level of the molecule. The lowest oxidation potential among these series of 11 (+0.65 V) should be attributed to the redox reaction of the substituted highly electron-donating p-anilino group. The electrochemical oxidation and reduction of 13 showed irreversible waves on DPV (E1ox = +1.11 V and E1red = −0.58 V) by the formation of a radical cationic and anionic species, respectively. Compound 14 also exhibited irreversible oxidation and reduction waves. The first redox potentials of 14 were identified as +0.88 V and −0.62 V by DPV. Recently, Diederich et al. have reported the compound 15 and its derivatives exhibit reversible multi-stage oxidation and reduction waves by CV.2 The irreversibility for the redox wave of DDQ-adducts 10–14 should be attributed to the instability of the 2H-cyclohepta[b]furan-2-one ring, compared to N,N-dimethylanilino group, in the electrochemical reaction.
In conclusion, the novel DDQ-adducts with 2H-cyclohepta[b]furan-2-one substituents 8–14 were synthesized by the [2+2] cycloaddition reaction of the ethynyl-2H-cyclohepta[b]furan-2-ones 1–7 with DDQ in good yields, except for the DDQ-adduct with electron-donating p-anilino substituent. The electronic and structural properties of the DDQ-adducts 8–14 were clarified by UV/Vis spectroscopy and X-ray crystallographic analysis. Electrochemical analyses by CV and DPV showed that these compounds 10–14 exhibited irreversible oxidation and reduction waves, whose potentials were depended considerably on the substituted aryl groups.
Azulene derivatives also have attracted much interest due to their characteristic electronic properties.13 As described above, 2H-cyclohepta[b]furan-2-one derivatives are one of versatile precursors for azulene derivatives. Thus, DDQ-adducts 8–14 may become a possible precursor for the DDQ-adducts with azulene functions. To evaluate the reactivity of the novel DDQ-adducts with cycloheptafuranone functions, conversion of the compounds 8–14 into azulene derivatives is currently progress in our laboratory.
EXPERIMENTAL
Melting points were determined with a Yanagimoto MPS3 micro melting apparatus and are uncorrected. Mass spectra were obtained with a Bruker APEX II instrument. IR and UV/Vis spectra were measured with JASCO FT/IR-4100 and Shimadzu UV-2550 spectrophotometers, respectively. 1H and 13C NMR spectra were recorded with a Bruker AVANCE400 spectrometer (at 400 MHz and 100 MHz, respectively), or a JEOL ECA500 spectrometer (at 500 MHz and 125 MHz, respectively). Voltammetry measurements were carried out with a BAS 100B/W electrochemical workstation equipped with Pt working and auxiliary electrodes and a reference electrode formed from Ag/AgNO3 (0.01 M) in acetonitrile containing tetrabutylammonium perchlorate (0.1 M). Elemental analyses were performed at the Research and Analytical Center for Giant Molecules, Graduate School of Science, Tohoku University.
Compound 8
DDQ (28 mg, 0.12 mmol) was added to a solution of 1 (21 mg, 0.10 mmol) in EtOAc (5 mL). The resulting mixture was refluxed for 12 h under an Ar atmosphere. The solvent was removed under reduced pressure. The residue was purified by column chromatography on silica gel with EtOAc as an eluent to give 8 (36 mg, 83%) as reddish brown crystals. Mp 105.0−107.0 °C (decomp.); IR (KBr disk): νmax = 2971 (w), 2220 (w), 1733 (s), 1712 (s), 1701 (s), 1611 (m), 1583 (m), 1564 (m), 1511 (s), 1490 (s), 1346 (w), 1324 (w), 1279 (m), 1251 (w), 1215 (m), 1197 (m), 1143 (m), 1052 (w), 1042 (w), 926 (w), 890 (w), 879 (w), 820 (m), 804 (m), 780 (w), 762 (w), 726 (w), 698 (w), 647 (w) cm-1; 1H NMR (400 MHz, DMSO-d6): δH = 7.89 (d, 1H, J = 1.2 Hz, H-4), 7.65–7.59 (m, 2H, H-7,8), 7.52–7.46 (m, 1H, H-6), 7.20 (s, 1H, H-8’), 3.19 (sept, 1H, J = 6.8 Hz, i-Pr), 1.38 (d, 3H, J = 6.8 Hz, i-Pr), 1.37 (d, 2H, J = 6.8 Hz, i-Pr) ppm; 13C NMR (100 MHz, DMSO-d6): δC = 178.29, 178.01, 166.12, 162.98, 158.38, 147.93, 141.77, 141.61, 140.93, 137.69, 136.40, 129.90, 127.00, 119.89, 115.16, 114.83, 96.90, 57.64, 56.00, 39.98, 24.10, 24.02 ppm; HRMS (ESI) Calcd for C22H12Cl2N2O4 + Na+ [M + Na]+ 461.0066. Found: 461.0065; Anal. Calcd for C22H12Cl2N2O4∙1/2H2O: C, 58.95; H, 2.92; N, 6.25. Found: C, 58.68; H, 3.07; N, 6.16.
Compound 9
The procedure used for the preparation of 8 was adopted here. The reaction of 2 (28 mg, 0.10 mmol) with DDQ (28 mg, 0.12 mmol) in EtOAc (5 mL) at refluxing temperature for 10 h afforded 9 (36 mg, 70%) as reddish brown crystals. Mp 95.0−98.0 °C (decomp.); IR (KBr disk): νmax = 2958 (w), 2219 (w), 1735 (s), 1711 (m), 1698 (m), 1645 (w), 1631 (w), 1595 (m), 1560 (w), 1512 (m), 1497 (m), 1464 (m), 1336 (w), 1314 (w), 1282 (m), 1252 (m), 1202 (w), 1141 (m), 1057 (w), 921 (w), 880 (w), 849 (m), 801 (m), 762 (w), 743 (w) cm-1; 1H NMR (400 MHz, CDCl3): δH = 7.94 (d, 1H, J = 1.2 Hz, H-4), 7.36 (dd, 1H, J = 11.2, 9.2 Hz, H-7), 7.27 (dd, 1H, J = 9.2, 1.2 Hz, H-8), 7.21 (ddd, 1H, J = 11.2, 1.2, 1.2 Hz, H-6), 3.09 (sept, 1H, J = 6.8 Hz, i-Pr), 1.40 (d, 6H, J = 6.8 Hz, i-Pr), 0.27 (s, 9H, SiMe3) ppm; 13C NMR (100 MHz, CDCl3): δC = 177.46, 176.59, 165.95, 162.02, 158.45, 157.69, 150.09, 149.22, 143.04, 142.50, 135.11, 134.80, 126.42, 117.79, 113.38, 113.09, 97.80, 57.27, 53.26, 39.97, 23.47, 23.25, −1.59 ppm; HRMS (ESI) Calcd for C25H20Cl2N2O4Si + Na+ [M + Na]+ 533.0462. Found: 533.0461; Anal. Calcd for C25H20Cl2N2O4Si: C, 58.71; H, 3.94; N, 5.48. Found: C, 58.53; H, 4.18; N, 5.43.
Compound 10
The procedure used for the preparation of 8 was adopted here. The reaction of 3 (30 mg, 0.10 mmol) with DDQ (28 mg, 0.12 mmol) in EtOAc (5 mL) at refluxing temperature for 14 h afforded 10 (45 mg, 84%) as reddish brown crystals. Mp 111.5−113.0 °C (decomp.); IR (KBr disk): νmax = 2965 (w), 2934 (w), 2872 (w), 2239 (w), 1736 (s), 1713 (m), 1701 (m), 1622 (w), 1588 (m), 1570 (m), 1514 (m), 1489 (s), 1464 (m), 1447 (w), 1404 (w), 1348 (w), 1320 (w), 1281 (m), 1269 (m), 1242 (m), 1204 (w), 1136 (w), 1046 (w), 1017 (w), 916 (w), 895 (w), 814 (w), 791 (w), 770 (w), 762 (w), 750 (w), 718 (w), 698 (w), 689 (w), 652 (w), 639 (w), 419 (w) cm-1; UV-Vis (CH2Cl2): λmax (log ε) = 260 (4.62), 280 sh (4.39), 306 sh (4.01), 418 (4.15), 451 sh (4.11) nm; 1H NMR (400 MHz, CDCl3): δH = 7.60 (dd, 1H, J = 11.2, 9.2 Hz, H-7), 7.55–7.46 (m, 5H, H-Ph), 7.47 (dd, 1H, J = 9.2, 0.8 Hz, H-8), 7.35 (ddd, 1H, J = 11.2, 1.2, 0.8 Hz, H-6), 6.90 (d, 1H, J = 1.2 Hz, H-4), 2.72 (sept, 1H, J = 6.8 Hz, i-Pr), 0.97 (d, 3H, J = 6.8 Hz, i-Pr), 0.96 (d, 3H, J = 6.8 Hz, i-Pr); 13C NMR (100 MHz, CDCl3): δC = 178.84, 177.47, 166.08, 162.21, 159.48, 149.33, 143.37, 142.54, 140.14, 136.66, 135.48, 133.66, 132.12, 130.18, 130.16, 128.66, 126.28, 118.23, 114.17, 113.70, 97.62, 56.99, 55.96, 39.57, 22.84, 22.74 ppm; HRMS (ESI): Calcd for C28H16Cl2N2O4 + Na+ [M + Na]+ 537.0379. Found: 537.0377; Anal. Calcd for C28H16Cl2N2O4∙2/5H2O: C, 64.36; H, 3.24; N, 5.36. Found: C, 64.37; H, 3.28; N 5.38.
Compound 11
The procedure used for the preparation of 8 was adopted here. The reaction of 4 (303 mg, 1.00 mmol) with DDQ (272 mg, 1.20 mmol) in EtOAc (30 mL) at refluxing temperature for 5 min afforded 11 (80 mg, 15%) as reddish brown crystals. Mp 224.0−227.0 °C (decomp.); IR (KBr disk): νmax = 3413 (w), 2968 (w), 2239 (w), 1768 (s), 1737 (s), 1621 (s), 1603 (m), 1525 (m), 1510 (m), 1492 (m), 1432 (w), 1401 (w), 1345 (w), 1316 (w), 1348 (m), 1284 (m), 1267 (m), 1239 (w), 1209 (m), 1175 (m), 1092 (w), 1059 (w), 1033 (w), 971 (w), 897 (m), 835 (m), 815 (m), 792 (m), 765 (m), 732 (w), 711 (w), 667 (w) cm-1; UV-Vis (CH2Cl2): λmax (log ε) = 242 sh (4.47), 265 (4.51), 310 sh (4.20), 324 (4.25), 376 (4.01), 439 (4.10) nm; 1H NMR (500 MHz, CDCl3): δH = 7.28 (d, 2H, J = 8.0 Hz, H-3’,5’), 7.20 (d, 2H, J = 11.2 Hz, H-7), 7.14 (d, 2H, J = 11.2 Hz, H-8), 6.97 (d, 2H, J = 11.2 Hz, H-6), 6.92 (s, 1H, H-4), 6.61 (d, 2H, J = 8.0 Hz, H-2’,6’), 4.07 (s, 2H, NH2), 2.64 (sept, 2H, J = 6.5 Hz, i-Pr), 1.07 (d, 3H, J = 6.5 Hz, i-Pr), 1.05 (d, 3H, J = 6.5 Hz, i-Pr) ppm; HRMS (ESI): Calcd for C28H17Cl2N3O4 + Na [M + Na]+ 552.0494. Found: 552.0490; Anal. Calcd for C28H17Cl2N3O4: C, 63.41; H, 3.23; N, 7.92. Found: C, 63.20; H, 3.44; N 7.80. Low solubility hampered the measurement of 13C NMR.
Compound 12
The procedure used for the preparation of 8 was adopted here. The reaction of 5 (160 mg, 0.48 mmol) with DDQ (172 mg, 0.76 mmol) in EtOAc (10 mL) at refluxing temperature for 18 h afforded 12 (199 mg, 74%) as reddish brown crystals. Mp 268.0−270.0 °C (decomp.); IR (KBr disk): νmax = 2960 (w), 2931 (w), 2219 (w), 1729 (s), 1700 (s), 1617 (w), 1588 (m), 1565 (w), 1560 (w), 1522 (m), 1515 (m), 1501 (w), 1487 (s), 1459 (w), 1340 (s), 1324 (m), 1286 (m), 1273 (w), 1247 (w), 1140 (w), 853 (w), 813 (w), 764 (w), 713 (w) cm-1; UV-Vis (CH2Cl2): λmax (log ε) = 257 (4.58), 274 sh (4.45), 322 sh (3.94), 352 sh (3.94), 368 (4.00), 463 (4.21) nm; 1H NMR (400 MHz, DMSO-d6): δH = 8.39 (ddd, 2H, J = 9.2, 2.4, 2.0 Hz, Ph-3,5), 7.76 (ddd, 2H, J = 9.2, 2.4, 2.0 Hz, Ph-2,6), 7.71 (dd, 1H, J = 11.2, 9.2 Hz, H-7), 7.58 (dd, 1H, J = 9.2, 0.8 Hz, H-8), 7.46 (ddd, 1H, J = 11.2, 1.2, 0.8 Hz, H-6), 7.04 (d, 1H, J = 1.2 Hz, H-4), 2.75 (sept, 1H, J = 6.8 Hz, i-Pr), 1.03 (d, 3H, J = 6.8 Hz, i-Pr), 1.01 (d, 3H, J = 6.8 Hz, i-Pr) ppm; 13C NMR (100 MHz, DMSO-d6): δC = 177.06, 165.76, 163.14, 159.21, 149.04, 148.81, 141.58, 140.41, 138.75, 138.21, 136.88, 136.63, 135.91, 129.87, 127.10, 125.30, 120.12, 114.35, 102.30, 96.66, 57.14, 56.36, 39.27, 23.50, 23.39 ppm; HRMS (ESI): Calcd for C28H15Cl2N3O6 + Na+ [M + Na]+ 582.0230. Found: 582.0228; Anal. Calcd for C28H15Cl2N3O6∙3/5H2O: C, 58.88; H, 2.86; N, 7.36. Found: C, 58.96; H, 3.00; N 7.48.
Compound 13
The procedure used for the preparation of 8 was adopted here. The reaction of 6 (178 mg, 0.60 mmol) with DDQ (169 mg, 0.77 mmol) in EtOAc (10 mL) at refluxing temperature for 7 h afforded 13 (239 mg, 76%) as reddish brown crystals. Mp 228.0−230 °C (decomp.); IR (KBr disk): νmax = 2967 (w), 2873 (w), 2253 (w), 2233 (w), 1735 (w), 1697 (m), 1685 (s), 1676 (s), 1636 (w), 1589 (m), 1555 (s), 1526 (m), 1517 (w), 1491 (m), 1453 (s), 1420 (m), 1356 (w), 1340 (w), 1269 (s), 1196 (m), 1175 (s), 1075 (m), 1053 (w), 1010 (w), 998 (m), 897 (m), 887 (m), 801 (m), 775 (w), 765 (w), 745 (w), 723 (m), 606 (w) cm-1; UV-Vis (CH2Cl2): λmax (log ε) = 271 (4.36), 284 sh (4.31), 347 (3.83), 406 (3.54), 486 sh (3.18) nm; 1H NMR (400 MHz, DMSO-d6): δH = 8.03 (dd, 1H, J = 4.8, 0.8 Hz, H-5’), 7.64−7.58 (m, 2H, H-8,7), 7.44–7.41(m, 1H, H-6), 7.32 (dd, 1H, J = 4.8, 4.0 Hz, H-4’), 7.28 (dd, 1H, J = 4.0, 0.8 Hz, H-3’), 7.19–7.16 (m, 1H, H-6), 7.12 (d, 1H, J = 0.8 Hz, H-4), 2.84 (sept, 1H, J = 6.8 Hz, i-Pr), 0.98 (d, 6H, J = 6.8 Hz, i-Pr) ppm; 13C NMR (100 MHz, DMSO-d6): δC = 177.93, 177.07, 165.83, 162.07, 158.63, 149.00, 141.50, 139.82, 138.18, 137.22, 136.45, 134.46, 132.27, 130.68, 129.76, 128.56, 125.68, 119.23, 114.36, 96.25, 57.43, 56.31, 55.87, 38.92, 23.33, 23.30 ppm; HRMS (ESI): Calcd for C26H14Cl2N2O4S + Na+ [M + Na]+ 542.9944. Found: 542.9940; Anal. Calcd for C26H14Cl2N2O4S: C, 59.90; H, 2.71; N, 5.37. Found: C, 59.61; H, 2.91; N, 5.22.
Compound 14
The procedure used for the preparation of 8 was adopted here. The reaction of 6 (107 mg, 0.27 mmol) with DDQ (96 mg, 0.42 mmol) in EtOAc (10 mL) at refluxing temperaturre for 1 h afforded 13 (99 mg, 59%) as reddish brown crystals. Mp 250.0−253.0 °C (decomp.); IR (KBr disk): νmax = 2963 (w), 2928 (w), 2909 (w), 2869 (w), 2222 (w), 1756 (s), 1741 (s), 1724 (s), 1712 (s), 1624 (w), 1588 (s), 1561 (w), 1519 (s), 1498 (s), 1484 (s), 1435 (w), 1427 (m), 1411 (w), 1384 (w), 1378 (w), 1365 (w), 1347 (w), 1304 (m), 1274 (s), 1258 (m), 1245 (m), 1213 (m), 1194 (w), 1151 (m), 1077 (w), 1061 (w), 1046 (w), 1014 (w), 924 (w), 911 (w), 899 (w), 803 (m), 797 (w), 795 (w), 784 (w), 764 (w), 757 (w), 717 (w), 707 (w), 648 (w), 626 (w) cm-1; UV-Vis (CH2Cl2): λmax (log ε) = 266 (4.64), 400 (4.40), 507 sh (3.97) nm; 1H NMR (400 MHz, CDCl3): δH = 7.31 (dd, 2H, J = 10.8, 9.2 Hz, H-7), 7.29 (br. s, 2H, H-4), 7.23 (d, 2H, J = 9.2 Hz, H-8), 7.06 (d, 2H, J = 10.8 Hz, H-6), 2.77 (sept, 2H, J = 6.8 Hz, i-Pr), 1.11 (d, 6H, J = 6.8 Hz, i-Pr), 1.09 (d, 6H, J = 6.8 Hz, i-Pr) ppm; HRMS (ESI): Calcd for C34H22Cl2N2O6 + Na+ [M + Na]+ 647.0747. Found: 647.0745; Anal. Calcd for C34H22Cl2N2O6∙1/2H2O: C, 64.36; H, 3.65; N, 4.42. Found: C, 64.33; H, 3.69; N 4.37; Low solubility hampered the measurement of 13C NMR.
ACKNOWLEDGEMENTS
This work was partially supported by a Grant-in-Aid for Research (Grant 22850007 and 25810019 to T.S.) from the Ministry of Education, Culture, Sports, Science, and Technology, Japan.
References
1. D. Walker and J. D. Hiebert, Chem. Rev., 1967, 67, 153. CrossRef
2. S. Kato, M. T. R. Beels, P. La Porta, W. B. Schweizer, C. Boudon, J.-P. Gisselbrecht, I. Biaggio, and F. Diederich, Angew. Chem. Int. Ed., 2010, 49, 6207. CrossRef
3. B. A. Trofimov, L. N. Sobenina, Z. V. Stepanova, I. A. Ushakov, L. M. Sinegovskaya, T. I. Vakul’skaya, and A. I. Mikhaleva, Synthesis, 2010, 470. CrossRef
4. (a) B. A. Trofimov, L. N. Sobenina, Z. V. Stepanova, I. A. Ushakov, A. I. Mikhaleva, D. N. Tomilin, O. N. Kazheva, G. G. Alexandrov, A. N. Chekhlov, and O. A. Dyachenko, Tetrahedron Lett., 2010, 51, 5028; CrossRef (b) L. N. Sobenina, Z. V. Stepanova, I. A. Ushakov, A. I. Mikhaleva, D. N. Tomilin, O. N. Kazheva, G. G. Alexandrov, O. A. Dyachenko, and B. A. Trofimov, Tetrahedron, 2011, 67, 4832. CrossRef
5. (a) K. Takase and M. Yasunami, J. Syn. Org. Chem. Jpn., 1981, 39, 1172; CrossRef (b) N. Morita, K. Toyota, and S. Ito, Heterocycles, 2009, 78, 1917; CrossRef (c) T. Shoji, S. Ito, K. Toyota, M. Yasunami, and N. Morita, Chem. Eur. J., 2008, 14, 8398. CrossRef
6. (a) K. Takase, T. Nakazawa, and T. Nozoe, Heterocycles, 1981, 15, 839; CrossRef (b) A. Mori, Y. Nukii, H. Takeshita, and T. Nozoe, Heterocycles, 1993, 35, 863; CrossRef (c) N. Morita, M. Kudo, R. Yokoyama, and S. Ito, Heterocycles, 2001, 54, 679; CrossRef (d) N. Morita, T. Matsuki, M. Nakashima, T. Shoji, K. Toyota, S. Kikuchi, and S. Ito, Heterocycles, 2006, 69, 119; CrossRef (e) N. Morita, J. Higashi, K. Okada, T. Shoji, K. Toyota, M. Watanabe, M. Yasunami, S. Kikuchi, and S. Ito, Heterocycles, 2007, 73, 237; CrossRef (f) J. Higashi, K Okada, T. Shoji, K. Toyota, M. Watanabe, M. Yasunami, S. Kikuchi, S. Ito, and N. Morita, Heterocycles, 2008, 76, 759; CrossRef (g) T. Shoji, J. Higashi, S. Ito, M. Oda, M. Yasunami, and N. Morita, Heterocycles, 2012, 86, 305. CrossRef
7. T. Shoji, J. Higashi, S. Ito, T. Okujima, M. Yasunami, and N. Morita, Chem. Eur. J., 2011, 17, 5116. CrossRef
8. T. Shoji, J. Higashi, S. Ito, T. Okujima, M. Yasunami, and N. Morita, Org. Biomol. Chem., 2012, 10, 2431. CrossRef
9. A synthetic part of this study was reported previously as a preliminary form: T. Shoji, J. Higashi, S. Ito, M. Yasunami, and N. Morita, Heterocycles, 2011, 83, 2271. CrossRef
10. Crystallographic data for the X-ray structural analysis have been deposited with the Cambridge Crystallographic Data Centre, CCDC no. 891965 for compound 13a. Copies of this information may be obtained free of charge from The Director, CCDC, 12 Union Road, Cambridge CB2 IEZ, UK (Fax: +44-1223-336033, e-mail: deposit@ccdc.cam.ac.uk or http://www.ccdc.cam.ac.uk). Selectee crystal data for 8: Pbca, orthorhombic, a = 20.0005(7) Å, b = 20.9837(5) Å, c = 12.0204(3) Å, α = 90º, β = 90º, γ = 90º, V = 5044.8(2) Å3, Z = 8, Dcalc = 1.347 g cm3, μ (MoKa) = 3.38 cm−1, R = 0.134, Rw = 0.123, R1 = 0.048.
11. Redox potentials were measured by CV and DPV [V vs. Ag/AgNO3, 1 mM in benzonitrile containing Et4NClO4 (0.1 M), Pt electrode (internal diameter: 1.6 mm), scan rate = 100 mVs−1, and Fc/Fc+ = +0.15 V].
12. The E4red was observed at −1.72 V on DPV.
13. (a) K.-P. Zeller, Azulene in Houben-Weyl: Methoden der Organischen Chemie, 4th ed., Georg Thieme, Stuttgart, Germany, 1985, Vol. V, Part 2c, p. 127; (b) S. Ito and N. Morita, Eur. J. Org. Chem., 2009, 4567; CrossRef (c) S. Ito, T. Shoji, and N. Morita, Synlett, 2011, 16, 2279. CrossRef