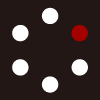
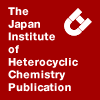
HETEROCYCLES
An International Journal for Reviews and Communications in Heterocyclic ChemistryWeb Edition ISSN: 1881-0942
Published online by The Japan Institute of Heterocyclic Chemistry
e-Journal
Full Text HTML
Received, 17th January, 2013, Accepted, 17th April, 2013, Published online, 2nd May, 2013.
DOI: 10.3987/COM-13-12669
■ Dimethyldioxirane Oxidation of N-Substituted-2-methylindoles to Indoxyls and Bisindoxyls
Alberto Aristeo-Dominguez, Myriam Meléndez-Rodríguez,* Oscar R. Suárez Castillo,* Yaneth M. A. Contreras-Martínez, Lizbeth Suárez-Ramírez, Nayely Trejo-Carbajal, Martha S. Morales-Ríos, and Pedro Joseph-Nathan
Área Académica de Química, Universidad Autónoma del Estado de Hidalgo, Mineral de la Reforma, Hidalgo, 42184, Mexico
Abstract
Oxidation of 2-methylindoles 12a-f with dimethyldioxirane (DMD) revealed that N-unprotected 12b,f and protected N-carbamate 2-methylindole 12c afforded indoxyls 16b,c and o-(N-propionyl)aminobenzoic acid 17f as the main products, while N-alkyl- or N-aryl-2-methylindoles 12a,d,e gave 1,5’-diphenyl-4’,5’-dihydro-3’H-spiro[indole-2,2’-pyrano[3,2-b]indol]-3(1H)-one (10), 2,3'-bisindolin-3-ones 13, dispiro[indole-2,2’-furan-5’,2”-indole]diones 14 or 2-[(3-oxoindolin-2-yl)methyl]-2-hydroxyindolin-3-ones 15. The structure of dimers 10, 13a,d,e, 14a,d and 15a,d followed from NMR measurements, X-ray diffraction analysis confirmed those structures of 13a,d,e, 14a,d and 15d, and some mechanistic implications are discussed.INTRODUCTION
The spiro[2H-indol]-3(1H)-one (spiro-indoxyl) moeity is found in the natural occurring mycotoxins brevianamide A (1) and B (2), isolated from Penicillium species, which have shown antifeedant and insecticidal effects.1 Other spiro-indoxyl molecules include rupicoline (3) and iboluteine (4) isolated from Tabernaemontana rupicola Benth2 and Tabernanthe iboga,3 respectively, austamide (5)4 isolated from Aspergillus ustus, and the phytoaliexin erucalexin (6)5 isolated from Erucastrum gallicum. In turn, spiro indoxyl (7) has been prepared as an example of a conformationally restricted isoindolylpiperidine analogue, which inhibits the microsomal triglyceride-transfer protein function.6 Structurally interesting indole dimers containing one or two indoxyl moieties such as 8, 9 and 10 have been synthesized. Thus, compounds like 8 are the major products isolated after treatment of 2-alkyl- or 2-arylindole with a variety of oxidizing agents,7 while spiroindole dimers 9 and 10 have only been obtained as by-products in 0.8% and 1%, respectively, when 3-hydroxy-3-methyl-1-phenylquinoline-2,4(1H,3H)-dione was treated with an aqueous KOH/benzene mixture.8 Spiro-indoxyl 10 is structurally related to spiropyran derivative 11, which belongs to a class of organic photochromic compounds that have extensively been studied for application in optical switches, memories9 and as anion receptors10 (Figure 1).
A number of reagents for the oxidation of the indole C2-C3 double bond to indoxyls have been reported,7 including NaIO4,7d N-chlorobenzotriazole,7g OsO4,11 oxodiperoxomolibdenum complexes,7f,12 bis(acetylacetonate)oxovanadium,13 dimethyldioxirane (DMD),12,14 singlet oxygen,7e m-CPBA,7h,15 H2O2,7h,16 HCl/MeOH17 and Pd(OAc)2.18 Besides, it has been shown that the oxidation products depend on the nature of the substituens at the N1, C2, and C3 positions of the indole nucleus.
We have already reported on the oxidation of N-protected-3-alkylindole derivatives with DMD to obtain exclusively 3-hydroxyoxindoles.19 In continuation with our studies aimed to shade light on the oxidation of indoles with this reagent, we report herein an approach to indoxyl dimers based on oxidation of 2-methylindole derivatives.
RESULTS AND DISCUSSION
Starting 2-methylindole derivatives 12a,b,d-f (Scheme 1) were obtained according to describe methodologies,20 while indole 12c was obtained in 76% yield when 2-methylindole (12b) was reacted with Me2CO3/DBU.19a
With compounds 12a-f in hand, we firstly treated 1,2-dimethylindole 12a with DMD/acetone (Scheme 1) giving rise to a mixture of five products identified as dimers 13a, 14a and 15a in 34%, 26% and 22% yield, respectively, together with indoxyl 16a and o-(N-acetyl)amino aldehyde (17a) in 0.7% and 5% yield, respectively. Compounds 13a-17a were separated by column chromatography and characterized by detailed analysis of their 1H and 13C NMR spectra, including gHSQC and gHMBC experiments, and mass spectra.
Formation of dimers 13a-15a and 2-hydroxyindoxyl 16a may be rationalized as shown in Scheme 2. Thus, oxidation of 12a with DMD afforded 2-hydroxyindoxyl 16a through indoxyl 19. At this respect, it is known that oxidation of 1,2-dialkyl indoles with DMD at low temperature affords oxindoles as the major products instead of indoxyls,14a but under our protocol indoxyl 16a should preferentially be formed in order to obtain the iminium ion 22 which could then be attacked by a second indole moiety 12a to afford the electrophilic aromatic substitution dimer 13a.7c The iminium ion 22 could also rearrange to 2-methyleneindolinone 23 which is further oxidized with DMD to epoxide 24 with subsequent nucleophilic attack of a second molecule of indoxyl 19 to give dimer 15a, or by 2-methyleneindolinone 23 to initially provide the zwitterionic intermediate 25, followed by intramolecular nucleophilic addition of the alkoxide to the electron deficient carbon atom of the iminium bond21 to afford the ring-closed dimer 14a. Although dimer 13a has been obtained using oxidating agents like singlet oxygen,7b MoO5·O=P(n-Pr)3H2O,7c m-CPBA7e,15 and H2O2,7e no such a compound has been reported in the oxidation of indoles with DMD. Besides, to the best of our knowledge, this is the first time dimers 14a and 15a are obtained by oxidation of indole derivatives.
As oxidation products of indole derivatives depend on the nature of the substituents at the N1, C2, and C3 positions,7 we decided to oxidize 2-methylindole (12b) under the same protocol, which afforded indoxyl 16b and o-(N-acetyl)aminobenzoic acid (17b) in 12% and 41% yield, respectively. It is worth noting that no dimerization products were observed when 12b was oxidized with DMD. Similarly, oxidation of N-carbomethoxy-2-methylindole (12c) gave indoxyl 16c in 76%, and again dimerization products were absent. These results suggest iminium ion 19 is formed when the 2-methylindole is N-substituted with an electron rich group since a hydrogen atom or an electron attracting group (-CO2Me) attenuate the internal nucleophilicity of the indole nitrogen atom11 and consequently dimer products are not promoted.
In order to explore the scope and limitations of this reaction, the reactivity profiles of 1-ethyl-2-methylindole (12d), 1-phenyl-2-methylindole (12e) and 2-ethylindole (12f) were studied. When 12d was reacted under the above reaction conditions, only dimers 13d, 14d and 15d were obtained in 32%, 15% and 11% yield, respectively, while 1-phenyl-2-methylindole (12e) gave 10, 13e and 16e in 23%, 30% and 5% yield, respectively. Oxidation of 12f afforded o-(N-propionyl)aminobenzoic acid 17f as the main product in 32% yield. Formation of compound 10 occurs through a hetero-Diels-Alder reaction between two molecules of 2-methyleneindolinone 2622 as is shown in Scheme 3.
Formation of adduct 10 could corroborate an indolinone intermediate like 23 shown in Scheme 2. Compounds 13a,d,e, 14a,d and 15d provided single crystals suitable for X-ray diffraction analysis, the corresponding structures are shown in Figure 2 and the pertinent data are summarized in Table 1.
Finally, acid catalyzed tetrahydrofuran ring opening of dimers 14a,d in an HCl/CHCl3 solution afforded vinylogous N,N’-dialkylindigos trans-trans-27a,d in quantitative yields (Scheme 4).
The trans-trans geometry for 27a,d follows from the chemical shift of the vinylic protons23 of 27a (δ = 8.04, s, 2H) and 27d (δ = 8.15, s, 2H).
While the room temperature 1H NMR spectrum of 14a shows only four proton signals for the aromatic rings and a strongly coupled AA’BB’ spin-spin system for the ethylene residue,8 the 1H NMR spectra of 13a and 15a evidenced eight signals for the aromatic ring protons, and dimer 13a further exhibited line broadening for the aromatic H4’ signal (broad doublet at 7.44 ppm, J = 7.7 Hz, W1/2 = 4.5 Hz) and the C2’-Me’ group (broad singlet at 2.27 ppm, W1/2 = 2.0 Hz). This broadening suggests the presence of conformational isomers arising from slow rotation around the C2-C3’ single bond (Scheme 5), a situation further evidenced when 1H NMR spectra of 13a were measured at temperatures other than the room temperature (20 °C) (Figure 3). When heating a solution of 13a to 35 °C in CDCl3 or to 70 °C in CDCl2CDCl2, the broad H4’ and C2’-Me’ resonances collapsed into sharp signals, while cooling below 0 °C in CD2Cl2 allowed observation of coalescence for the signals owing to H4’ and H5’ at -20 °C and -60 °C, respectively, while the C2’-Me’ signal vanishes at -40 °C.
As evidenced in Figure 3, when cooling a solution of 13a, the broadening of the H4’-H7’signals is higher than that for the H4-H7 signals, which means the chemical environment for the protons of the indole portion is more different than that for the indoxyl portion. Furthermore, conformational evaluations of 13a were carried out by systematic and Monte Carlo search protocols within the Spartan 04 program24 employing the MMFF94 molecular mechanics force field. According to the conformer distribution, only four models arose as relevant contributors, which were submitted to geometry optimization using DFT calculations at the B3LYP/6-31G(d) level of theory. The Boltzmann distribution (p) and relative energy (Erel) for these four conformers are shown in Figure 4.
In conclusion, we demonstrated that 2-methylindole derivatives 12a,d,e are easily oxidized to indole dimers with DMD and that the N1 substituent influences the ratio and type of obtained monomers or dimers.
EXPERIMENTAL
General experimental procedures
Melting points were determined on a Büchi B-540 apparatus. IR spectra were recorded on a Perkin-Elmer GX FT-IR spectrophotometer. The 400 and 100 MHz 1H and 13C NMR spectra were obtained on JEOL Eclipse + 400 and Varian VNMRS 400 spectrometers using CDCl3, CD2Cl2 or CDCl2CDCl2 as solvents. The chemical shifts of the residual hydrogen present in the deuterated solvents were used as reference.25 For complete assignments 2D NMR, gHSQC and gHMBC spectra were used. Data are reported as follows: chemical shift in ppm, integration, multiplicity (s = singlet, d = doublet, t = triplet, q = quartet, m = multiplet, br = broad, AB = AB system, AA’BB’ system), coupling constant (Hz), and assignment. GC/MS analyses were conducted on a Varian CP 3800 GC equipped with a Varian Saturn 2000 selective mass detector and a 30 m, 0.25 mm i.d., CP-SIL capillary column, using helium as the carrier gas (1 mL/min), programmed from 70 °C to 250 °C at a rate of 30 °C/min, with the injector temperature at 200 °C. MS analyses were obtained in the electron impact (EI) mode at an ionizing voltage of 70 eV on a Hewlett Packard 5989-A spectrometer. Microanalytical determinations were performed on a Perkin-Elmer 2400 series PCII apparatus. Analytical thin-layer chromatography (TLC) was done on silica gel F254 coated aluminum sheets (0.25 mm thickness) with a fluorescent indicator. Visualization was accomplished with UV light (254 nm). Flash chromatography was done using Silica Gel 60 (230-400 mesh) from Aldrich.
General procedure for the oxidation of 2-alkylindoles 12a-f.
To a solution of 3.44 mmol of the appropriate indole 12a (0.500 g), 12b (0.452 g), 12c (0.652 g), 12d (0.548 g), 12e (0.714 g) or 12f (0.499 g) in acetone (50 mL) was added dropwise a solution of EDTA (0.031 g, 0.031 equiv) and NaHCO3 (1.014 g, 3.5 equiv) dissolved in the minimum amount of water. The resulting thick mixture was treated dropwise with a solution of oxone monopersulfate complex (2.650 g, 2.5 equiv of KHSO5) in water (5-10 mL) at room temperature over 10 min. After complete addition, the mixture was stirred at room temperature for 1 h, filtered off, the acetone was evaporated under reduce pressure and the residue was dissolved in EtOAc (50 mL). The organic phase was washed with brine (2 x 25 mL), dried over Na2SO4 and concentrated in vacuo. The resultant crude products were purified by flash column chromatography with EtOAc/hexane 1:3 for 13a-17a and 13d-15d, with EtOAc/hexane 1:4 for 10, 13e, 16b,c,e and 17b, and with EtOAc/MeOH 44:1 for 17f.
2-(1,2-Dimethyl-1H-indol-3-yl)-1,2-dimethyl-1,2-dihydro-3H-indol-3-one (13a).
Prepared from 12a as green crystals (177.0 mg, 34%); mp 187-188 °C (EtOAc/hexanes). Lit.,7h 155–157 °C, no crystallization solvent reported. Although 13a is known for four decades,7e it is spectroscopically not yet fully characterized. Thus, NMR data follow: 1H NMR (CDCl3, 400 MHz) δ 7.68 (1H, ddd, J = 7.7, 1.5, 0.8 Hz, H4), 7.53 (1H, td, J = 7.9, 1.3 Hz, H6), 7.44 (1H, brd, J = 7.7 Hz, H4’), 7.24 (1H, d, J = 8.4 Hz, H7’), 7.12 (1H, td, J = 7.4, 1.0 Hz, H6’), 6.97 (1H, td, J = 7.9, 1.1 Hz, H5’), 6.78 (1H, d, J = 8.1 Hz, H7), 6.75 (1H, t, J = 7.0 Hz, H5), 3.63 (3H, s, NMe’), 2.86 (3H, s, NMe), 2.27 (3H, s, Me’), 1.90 (3H, s, Me); 13C NMR (CDCl3, 100 MHz) δ 204.0 (C=O), 159.1 (C7a), 137.9 (C6), 136.9 (C7a’), 135.8 (C2’), 127.5 (C3a’), 125.6 (C4), 120.8 (C6’), 119.8 (C4’), 119.7 (C5’), 118.5 (C3a), 116.9 (C5), 109.2 (C7’), 108.3 (C7), 106.8 (C3’), 71.4 (C2), 29.6 (NMe’), 28.0 (NMe), 22.2 (Me’), 12.1 (Me).
1-Ethyl-2-(1-ethyl-2-methyl-1H-indol-3-yl)-2-methylindolin-3-one (13d).
Prepared from 12d as green crystals (183.2 mg, 32%); mp 107-108 °C (EtOAc/hexanes). 1H NMR (CDCl3, 400 MHz) δ 7.69 (1H, ddd, J = 8.2, 1.4, 0.7 Hz, H4), 7.51 (1H, ddd, J = 8.4, 7.1, 1.4 Hz, H6), 7.50 (1H, overlapped, H4’), 7.26 (1H, d, J = 8.2 Hz, H7’), 7.11 (1H, ddd, J = 8.2, 7.1, 1.1 Hz, H6’), 6.98 (1H, brt, J = 7.6 Hz, H5’), 6.77 (1H, d, J = 8.4 Hz, H7), 6.73 (1H, td, J = 7.4, 0.8 Hz, H5), 4.10 (2H, q, J = 7.3 Hz, CH2’CH3’), 3.33 (2H, q, J = 7.2 Hz, CH2CH3), 2.19 (3H, brs, CH3’), 1.95 (3H, s, CH3), 1.30 (3H, t, J = 7.2 Hz, CH2’CH3’), 1.04 (3H, t, J = 7.2 Hz, CH2CH3); 13C NMR (CDCl3, 100 MHz) δ 204.0 (C3=O), 157.9 (C7a), 137.6 (C6), 135.6 (C7a’), 134.8 (C2’), 127.7 (C3a’), 125.6 (C4), 120.5 (C6’), 119.7 (C4’), 119.5 (C5’), 118.1 (C3a), 116.3 (C5), 109.0 (C7’), 108.1 (C7), 107.0 (C3’), 71.5 (C2), 37.5 (CH2’CH3´), 37.0 (CH2CH3), 23.4 (Me), 15.1 (CH2’CH3´), 14.1 (CH2CH3), 11.6 (Me’); IR (KBr) νmax 3014, 2969, 2931, 2869, 1690, 1616, 1492, 1467, 1349, 1322 cm-1; EIMS m/z 332 [M+] (49), 317 (53), 289 (88), 213 (26), 173 (53), 158 (66), 144 (100), 130 (44), 117 (31), 103 (17), 77 (14).
2-Methyl-2-(2-methyl-1-phenyl-1H-inden-3-yl)-1-phenylindolin-3-one (13e).
Prepared from 12e as orange crystals (219.2 mg, 30%); mp 121-122 °C (EtOAc/hexanes). 1H NMR (CDCl3, 400 MHz) δ 7.78 (1H, d, J = 7.6 Hz, H4), 7.55-6.85 (17H, overlapped, H5-H7, H4’-H7’, H9-H13, H9’-H13’), 2.13 (3H, brs, Me or Me’), 1.83 (3H, brs, Me or Me’); 13C NMR (CDCl3, 100 MHz) δ 203.3 (C3), 157.9 (C7a), 139.8, 137.8, 137.4, 137.3, 129.4, 129.3, 128.8, 128.1, 126.0, 125.4 (C4), 121.3, 120.3, 119.8, 119.6, 118.9, 111.4, 110.2, 109.8, 72.6 (C2), 22.9, 12.90 (Me, Me’); IR (film) νmax 3059, 2922, 2850, 1716, 1610, 1595, 1499, 1478, 1466, 1454, 1371, 1319 cm-1; EIMS m/z 428 [M+] (34), 414 (30), 400 (17), 386 (100), 324 (27), 310 (30), 233 (37), 223 (65).
1,1’’-Dimethyl-3’,4’-dihydrodispiro[indole-2,2’-furan-5´,2”-indole]-3,3”(1H,1”H)-dione (14a).
Prepared from 12a as yellow crystals (149.7 mg, 26%); mp 170-173 °C (EtOAc/hexanes). 1H NMR (CDCl3, 400 MHz) δ 7.52 (2H, d, J = 7.7 Hz, H4, H4’’), 7.45 (2H, td, J = 7.8, 1.5 Hz, H6, H6’’), 6.72 (2H, t, J = 7.3 Hz, H5, H5’’), 6.67 (2H, d, J = 8.1 Hz, H7, H7’’), 3.07 (6H, s, Me, Me’’), 2.76 and 2.15 (4H, AA’BB’, H3’,H4’); 13C NMR (CDCl3, 100 MHz) δ 201.2 (C3, C3’’), 161.2 (C7a, C7a’’), 138.7 (C6, C6’’), 125.2 (C4, C4’’), 118.1 (C5, C5’’), 117.6 (C3a, C3a’’), 108.5 (C7, C7’’), 97.1 (C2, C2’’), 28.5 (C3’, C4’), 26.9 (Me, Me’’); IR (film) νmax 2949, 1713, 1616, 1584, 1486, 1434, 1370, 1323 cm-1; EIMS m/z 334 [M+] (68), 199 (5), 175 (49), 159 (100), 133 (58), 105 (7); Anal. Calcd for C20H18N2O3: C 71.84; H 5.43; N 8.38. Found: C 71.71; H 5.54; N 8.38. FABHRMS m/z 334.1311 (calcd for C20H18N2O3, 334.1317).
1,1’’-Diethyl-3’,4’-dihydrodispiro[indole-2,2’-furan-5´,2”-indole]-3,3”(1H,1”H)-dione (14d).
Prepared from 12d as yellow crystals (96.1 mg, 15%); mp 167-170 °C (EtOAc/hexanes). 1H NMR (CDCl3, 400 MHz) δ 7.52 (2H, ddd, J = 7.6, 1.4, 0.7 Hz, H4, H4’’), 7.44 (2H, td, J = 7.1, 1.3 Hz, H6, H6’’), 6.69 (2H, td, J = 7.4, 0.8 Hz, H5, H5’’), 6.66 (2H, brd, J = 8.6 Hz, H7, H7’’), 3.71 (2H, dq, J = 15.1, 7.2 Hz, CH2CH3, CH2’’CH3’’), 3.56 (2H, dq, J = 15.1, 7.2 Hz, CH2CH3, CH2’’CH3’’), 2.80 and 2.11 (4H, AA’BB’, H3’, H4’), 1.22 (6H, t, J = 7.1 Hz, CH2CH3, CH2’’CH3’’); 13C NMR (CDCl3, 100 MHz) δ 201.6 (C3=O, C3’’=O), 160.1 (C7a, C7a’’), 138.4 (C6, C6’’), 125.2 (C4, C4’’), 117.4 (C5, C5’’), 117.2 (C3a, C3a’’), 108.4 (C7, C7’’), 96.7 (C2, C2’’), 35.0 (CH2CH3, CH2’’CH3’’), 28.9 (C3’,C4’), 13.9 (CH2CH3, CH2’’CH3’’); IR (KBr) νmax 2969, 2918, 2850, 1703, 1616, 1485, 1336, 1313 cm-1; EIMS m/z 362 [M+] (100), 345 (16), 188 (63), 172 (47), 173 (97), 158 (63), 147 (79), 130 (73), 117 (70), 104 (40), 77 (20), 51 (9). FABHRMS m/z 362.1639 (calcd for C22H22N2O3, 362.1630).
2-[(1,2-Dimethyl-3-oxoindolin-2-yl)methyl]-2-hydroxy-1-methylindolin-3-one (15a).
Prepared from 12a as yellow crystals (127.4 mg, 22%); mp 146-149 °C (EtOAc/hexanes). 1H NMR (CDCl3, 400 MHz) δ 7.39 (1H, d, J = 7.3 Hz, H4’), 7.29 (1H, dd, J = 8.4, 2.2 Hz, H4), 7.00 (1H, td, J = 6.9, 1.4 Hz, H6’), 6.97 (1H, td, J = 7.2, 1.2 Hz, H6), 6.57 (1H, td, J = 7.3, 0.7 Hz, H5’), 6.50 (1H, td, J = 7.4, 0.7 Hz, H5), 6.01 (1H, d, J = 8.4 Hz, H7’), 5.87 (1H, d, J = 8.0 Hz, H7), 3.89 (1H, brs, OH), 2.74 and 2.63 (2H, AB, J = 14.7 Hz, H8), 2.48 (3H, s, NMe), 2.42 (3H, s, NMe’), 1.08 (3H, s, Me’); 13C NMR (CDCl3, 100 MHz) δ 201.6 (C3’), 198.8 (C3), 159.6 (C7a), 159.3 (C7a’), 136.4 (C6), 135.6 (C6’), 123.4 (C4), 123.2 (C4’), 121.8 (C3a’), 119.9 (C3a), 118.0 (C5, C5’), 109.7 (C7’), 109.3 (C7), 87.8 (C2), 67.6 (C2’), 45.0 (C8), 28.4 (NMe’), 27.5 (NMe), 20.5 (Me’); IR (film) νmax 3386, 2927, 2883, 2825, 1698, 1617, 1485, 1361, 1320 cm-1. EIMS m/z 336 [M+] (36), 318 (4), 174 (100), 160 (46), 134 (10). FABHRMS m/z 336.1469 (calcd for C20H20O3N2, 336.1474).
1-Ethyl-2-((1-ethyl-2-hydroxy-3-oxoindolin-2-yl)methyl)-2-methylindolin-3-one (15d).
Prepared from 12d as yellow crystals (69.0 mg, 11%); mp 158-160 °C (EtOAc/hexanes). 1H NMR (CDCl3, 400 MHz) δ 7.35 (1H, ddd, J = 7.7, 1.3, 0.6 Hz, H4’), 7.27 (1H, ddd, J = 7.6, 1.3, 0.6 Hz, H4), 6.89 (2H, td, J = 7.7, 0.6 Hz, H6, H6’), 6.46 (1H, td, J = 7.5, 0.7 Hz, H5’), 6.42 (1H, td, J = 7.5, 0.7 Hz, H5), 5.84 (1H, d, J = 8.3 Hz, H7’), 5.75 (1H, d, J = 8.3 Hz, H7), 3.09 (1H, dq, J = 15.5, 7.5 Hz, CH2CH3), 2.89 (2H, m, CH2’CH3), 2.79 (1H, dq, J = 15.5, 7.0 Hz, CH2CH3), 2.74 and 2.59 (2H, AB, J = 14.6 Hz, H8), 1.10 (3H, s, Me’), 0.93 (3H, t, J = 7.1 Hz, CH2CH3), 0.88 (3H, t, CH2CH3’); 13C NMR (CDCl3, 100 MHz) δ 201.7 (C3’), 199.4 (C3), 157.6 (C7a), 157.3 (C7a’), 136.3 (C6), 135.3 (C6’), 123.6 (C4), 123.3 (C4´), 121.4 (C3a’), 119.5 (C3a), 117.3 (C5’), 116.9 (C5), 110.1 (C7’), 109.8 (C7), 88.1 (C2), 67.5 (C2’), 46.1 (C8), 36.1 (CH2’CH3’), 35.9 (CH2CH3), 22.2 (Me’), 12.4 (CH2CH3), 11.8 (CH2’CH3’); IR (KBr) νmax 3372, 2970, 2918, 2850, 1715, 1674, 1619, 1490, 1481, 1326 cm-1. EIMS m/z 364 [M+] (9), 318 (6), 188 (61), 174 (100), 160 (23), 146 (20), 130 (21).
2-Hydroxy-1,2-dimethylindolin-3-one (16a).
Prepared from 12a as red oil (4.2 mg, 0.7%). 1H NMR (CDCl3, 400 MHz) δ 7.98 (1H, ddd, J = 7.9, 1.6, 0.5 Hz, H4), 7.50 (1H, ddd, J = 8.4, 7.3, 1.6 Hz, H6), 7.07 (1H, ddd, J = 7.9, 7.3, 0.9 Hz, H5), 6.81 (1H, brd, J = 8.4 Hz, H7), 3.25 (3H, s, Me9), 1.62 (3H, s, Me8); 13C NMR (CDCl3, 100 MHz) δ 187.6 (C3), 153.0 (C7a), 135.1 (C6), 128.6 (C4), 122.0 (C5), 117.2 (C3a), 112.6 (C7), 99.8 (C2), 40.6 (C9), 19.7 (C8); IR (film) νmax 3382, 2920, 2851, 1690, 1615, 1482, 1371, 1323, 1295 cm-1. EIMS m/z 177 [M+] (8), 176 (80), 150 (25), 134 (20), 132 (100), 104 (31), 77 (12), 43 (9).
2-Hydroxy-2-methylindolin-3-one (16b).
Prepared from 12b as yellow crystals (69.3 mg, 12%); mp 120 °C (EtOAc/hexanes). 1H NMR (CDCl3, 400 MHz) δ 7.61 (1H, dm, J = 7.8 Hz, H4), 7.49 (1H, ddd, J = 8.3, 7.0, 1,3 Hz, H6), 6.94 (1H, dt, J = 8.3, 0.8 Hz, H7), 6.81 (1H, ddd, J = 7.8, 7.1, 0.8 Hz, H5), 6.08 (1H, brs, NH), 1.66 (1H, brs, OH), 1.15 (3H, s, Me8); 13C NMR (CDCl3, 100 MHz) δ 204.1 (C3), 160.9 (C7a), 138.1 (C6), 124.7 (C4), 119.7 (C3a), 118.5 (C5), 112.2 (C7), 68.5 (C2), 18.2 (C8); IR (KBr) νmax 3372, 3315, 2975, 2919, 1677, 1616, 1488, 1453, 1400, 1376, 1324, 1299, 1270 cm-1; EIMS m/z 146 [M+-17] (68), 117 (48), 104 (15), 89 (14).
Methyl 2-methyl-3-oxoindoline-1-carboxylate (16c).
Prepared from 12c as white crystals (534.8 mg, 76%); mp 71-72 °C (EtOAc/hexanes). 1H NMR (CDCl3, 400 MHz) δ 8.24 (1H, brs, H7), 7.74 (1H, brd, J = 6.9 Hz, H4), 7.67 (1H, td, J = 7.9, 1.4 Hz, H6), 7.18 (1H, td, J = 7.5, 0.8 Hz, H5), 4.29 (1H, brs, H2), 3.92 (3H, s, CO2Me), 1.56 (3H, d, J = 7.1 Hz, C2-Me); 13C NMR (CDCl3, 100 MHz) δ 199.3 (C3), 152.4 (C9, C7a), 137.2 (C6), 124.1 (C4), 123.4 (C5), 123.2 (C3a), 116.8 (C7), 61.5 (C2), 53.1 (CO2Me), 16.9 (C2-Me); IR (KBr) νmax 3412, 3004, 2986, 2956, 2940, 1716, 1607, 1472, 1434, 1377, 1324, 1299, 1270 cm-1. EIMS m/z 205 [M+] (100), 162 (35), 146 (39), 118 (73), 91 (20).
2-Hydroxy-2-methyl-1-phenylindolin-3-one (16e).
Prepared from 12e as a green oil (43.8 mg, 5%). 1H NMR (CDCl3, 400 MHz) δ 7.63 (1H, dm, J = 7.7 Hz, H4), 7.50-7.40 (5H, overlapped, H10, H14, H11, H13, H6), 7.31 (1H, tt, J = 7.0, 1.7, Hz, H12), 6.88 (1H, dd, J = 8.4, 0.5 Hz, H7), 6.83 (1H, ta, J = 7.7 Hz, H5), 3.40 (1H, brs, OH), 1.34 (3H, s, C8); 13C NMR (CDCl3, 100 MHz) δ 200.9 (C3), 158.3 (C7a), 138.3 (C9), 138.2 (C6), 129.5 (C10, C14), 126.7 (C12), 126.1 (C11, C13), 125.6 (C4), 119.4 (C5), 117.8 (C3a), 111.0 (C7), 89.1 (C2), 21.4 (Me8); IR (film) νmax 3411, 3387, 1704, 1612, 1594, 1498, 1481, 1467, 1366, 1320 cm-1. EIMS m/z 239 [M+] (6), 221 (100), 192 (35), 165 (14), 89 (10), 77 (7), 63 (5), 51 (9).
N-(2-Formylphenyl)-N-methylacetamide (17a).
Prepared from 12a as a brown oil (32.1 mg, 5%). Although 17a is known,26 it is spectroscopically not yet fully characterized. Thus, NMR data follow: 1H NMR (CDCl3, 400 MHz) δ 10.16 (1H, s, H7), 8.00 (1H, dd, J = 7.8, 1.5 Hz, H6), 7.73 (1H, td, J = 7.7, 1.7 Hz, H4), 7.56 (1H, ta, J = 7.6 Hz, H5), 7.32 (1H, dd, J = 7.9, 1.0 Hz, H3), 3.31 (3H, s, NMe), 1.82 (3H, s, COMe); 13C NMR (CDCl3, 100 MHz) δ 189.4 (HC=O), 170.5 (MeC=O), 146.1 (C2), 135.8 (C4), 132.3 (C1), 130.3 (C6), 129.1 (C3), 129.0 (C5), 37.9 (NMe), 22.4 (MeC=O) IR (film) νmax 2962, 2925, 2854, 2753, 1695, 1661, 1597, 1489, 1456, 1424, 1382, 1352, 1301, 1263; EIMS m/z 177 [M+] (6), 148 (58), 135 (46), 106 (100), 77 (19), 51 (17), 43 (27).
2-Acetamidobenzoic acid (17b).
Prepared from 12b as brown crystals (252.6 mg, 41%). 1H NMR and 13C NMR spectroscopic data match those reported.27
2-Propionamidobenzoic acid (17f).
Prepared from 12f as a brown oil (215.0 g, 32%). 1H NMR spectroscopic data match those reported.28
1,5'-Diphenyl-4',5'-dihydro-3'H-spiro[indoline-2,2'-pyrano[3,2-b]indol]-3-one (10).
Prepared from 12e as orange crystals (176.0 mg, 23%); mp 192-193 °C (EtOAc/hexanes). Lit.,8 192 °C. 1H NMR and 13C NMR spectroscopic data match those reported.8
General procedure to obtain N,N’-dialkylindigos 27a,d.
To a solution of 0.15 mmol of the appropriate spirobisindoxyl 14a (50 mg) or 14d (54 mg) in CHCl3 (10 mL) was added two drops of concentrated HCl (38%) and the mixture was stirred at room temperature for 1 h. The volatiles were evaporated under vacuum to afford 27a or 27d.
(2E,2'E)-2,2'-(Ethane-1,2-diylidene)bis(1-methylindolin-3-one) (27a).
Prepared from 14a as a blue solid (47 mg, 99%); mp 274-275 °C (CHCl3). Although 27a is known,23 it is spectroscopically not yet fully characterized. Thus, NMR data follow: 1H NMR (CDCl3, 400 MHz) δ 8.04 (2H, s, 2H8), 7.66 (2H, dm, J = 7.7 Hz, 2H4), 7.45 (2H, td, J = 7.7, 1.2 Hz, 2H6), 6.88 (2H, d, J = 8.1 Hz, 2H7), 6.89 (2H, t, J = 7.1 Hz, 2H5), 3.38 (6H, s, 2Me); 13C NMR (CDCl3, 100 MHz) δ 185.7 (2C3), 151.6 (2C7a), 138.0 (2C2), 135.5 (2C6), 124.4 (2C4), 121.5 (2C3a), 119.5 (2C5), 111.7 (2C8), 108.9 (2C7), 28.8 (2Me).
(2E,2'E)-2,2'-(Ethane-1,2-diylidene)bis(1-ethylindolin-3-one) (27d).
Prepared from 14d as a blue solid (51 mg, 99%); mp 237-239 °C (CHCl3). Although 27d is known,23 it is spectroscopically not yet fully characterized. Thus, NMR data follow: 1H NMR (CDCl3, 400 MHz) δ 8.15 (2H, s, 2H8), 7.67 (2H, d, J = 8.3 Hz, 2H4), 7.46 (2H, td, J = 7.7, 1.3 Hz, 2H6), 6.91 (2H, d, J = 8.0 Hz, 2H7), 6.90 (2H, t, J = 7.5 Hz, 2H5), 3.89 (4H, q, J = 7.2 Hz, 2CH2), 1.35 (6H, t, J = 7.2 Hz, 2CH3); 13C NMR (CDCl3, 100 MHz) δ 186.0 (2C3), 150.7 (2C7a), 136.6 (2C2), 135.5 (2C6), 124.6 (2C4), 121.7 (2C3a), 119.5 (2C5), 111.7 (2C8), 108.9 (2C7), 37.0 (2CH2), 12.1 (2CH3).
Single crystal X-ray diffraction analyses
Data collections for 13a,d,e, 14a,d and 15d were done on an Enraf-Nonius CAD4 diffractometer using Cu Kα radiation (λ = 1.54184 Å). The structures were solved by direct methods using the SHELXS-9729 program included in the WINGX v1.6 package.30 Structural refinements were carried out by full-matrix least squares on F2. The non-hydrogen atoms were treated anisotropically, and the hydrogen atoms, included in the structure factor calculation, were refined isotropically. Atomic coordinates, bond lengths, bond angles and anisotropic thermal parameters are in deposit at the Cambridge Crystallographic Data Center. Table 1 summarizes the relevant data and CCDC deposition numbers.
ACKNOWLEDGMENTS
We are pleased to acknowledge the financial support from CONACYT (Mexico) grant 132048. AAD thanks fellowship No. 256340 from CONACYT.
References
1. J. M. Finefield, J. C. Frisvad, D. H. Sherman, and R. M. Williams, J. Nat. Prod., 2012, 75, 812. CrossRef
2. C. Niemann and J. W. Kessel, J. Org. Chem., 1966, 31, 2265. CrossRef
3. D. F. Dickel, C. L. Holden, R. C. Maxfield, L. E. Paszek, and W. I. Taylor, J. Am. Chem. Soc., 1958, 80, 123. CrossRef
4. (a) P. S. Steyn, Tetrahedron Lett., 1971, 3331; CrossRef (b) P. S. Steyn, Tetrahedron, 1973, 29, 107. CrossRef
5. M. S. C. Pedras and D. P. O. Okinyo, Chem. Commun., 2006, 1848. CrossRef
6. R. Sulsky, J. Z. Gougoutas, J. DiMarco, and S. A. Biller, J. Org. Chem., 1999, 64, 5504. CrossRef
7. (a) R. J. Sundberg, 'The Chemistry of indoles', Academic Press, New York and London, 1970, p. 282; (b) S. P. Hiremath and M. Hooper, 'Advances in Heterocyclic Chemistry', Vol. 22, Academic Press, New York and London, 1978, p. 123; (c) R. J. Sundberg, 'Indoles', Academic Press, London and San Diego, 1996, p. 152; (d) L. J. Dolby and R. M. Rodia, J. Org. Chem., 1970, 35, 1493; CrossRef (e) I. Saito, M. Imuta, and T. Matsuura, Chem. Lett., 1972, 1173; CrossRef (f) C. I. A. Kiraz, T. J. Emge, and L. S. Jimenez, J. Org. Chem., 2004, 69, 2200; CrossRef (g) C. Berti, L. Greci, R. Andruzzi, and A. Trazza, J. Org. Chem., 1982, 47, 4895; CrossRef (h) P. Astolfi, L. Greci, C. Rizzoli, P. Sgarabotto, and G. Marrosu, J. Chem. Soc., Perkin Trans. 2, 2001, 1634; CrossRef (i) E. Braudeau, S. David, and J. C. Fischer, Tetrahedron 1974, 30, 1445; CrossRef (j) Z. Wang and L. S. Jimenez, J. Am. Chem. Soc., 1994, 116, 4977; CrossRef (k) M. Nakagawa, N. Ohyoshi, and T. Hino, Heterocycles, 1976, 4, 1275; CrossRef (l) X. Zhang, C. S. Foote, and S. I. Khan, J. Org. Chem., 1993, 58, 47; CrossRef (m) K. Q. Ling, Synth. Commun., 1995, 25, 3831; CrossRef (n) K. Q. Ling, Synth. Commun., 1996, 26, 149; CrossRef (o) E. Braudeau, S. David, and J. C. Fischer, Tetrahedron, 1974, 30, 1445; CrossRef (p) J. B. Patrick and B. Witkop, J. Org. Chem., 1954, 19, 1824. CrossRef
8. J. Kosmrlj, S. Kafka, I. Leban, and M. Grad, Magn. Reson. Chem., 2007, 45, 700. CrossRef
9. (a) G. Berkovic, V. Krongauz, and V. Weiss, Chem. Rev., 2000, 100, 1741; CrossRef (b) L. Zhu, W. Wu, M.-Q. Zhu, J. J. Han, J. K. Hurst, and A. D. Q. Li, J. Am. Chem. Soc., 2007, 129, 3524; CrossRef (c) F. M. Raymo and S. Giordani, J. Am. Chem. Soc., 2001, 123, 4651; CrossRef (d) B. I. Ipe, S. Mahima, and K. G. Thomas, J. Am. Chem. Soc., 2003, 125, 7174. CrossRef
10. (a) Y. Shiraishi, K. Adachi, M. Itoh, and T. Hirai, Org. Lett., 2009, 11, 3482; CrossRef (b) Y. Shiraishi, M. Itoh, and T. Hirai, Tetrahedron, 2011, 67, 891; CrossRef (c) S. Sumiya, T. Doi, Y. Shiraishi, T. Hirai, Tetrahedron, 2012, 68, 690. CrossRef
11. G. B. Feigelson and S. J. Danishefsky, J. Org. Chem., 1988, 53, 3391. CrossRef
12. (a) A. Karadeolian and M. A. Kerr, J. Org. Chem., 2010, 75, 6830; CrossRef (b) C. Chien, T. Suzuki, T. Kawasaki, and M. Sakamoto, Chem. Pharm. Bull., 1984, 32, 3945; CrossRef (c) C. Chien, T. Takanami, T. Kawasaki, and M. Sakamoto, Chem. Pharm. Bull., 1985, 33, 1843; CrossRef (d) Z. Wang and L. Jimenez, Tetrahedron Lett., 1996, 34, 6049. CrossRef
13. E. Balogh-Hergovich and G. Speier, J. Mol. Catal., 1989, 57, L9. CrossRef
14. (a) X. Zhang and C. S. Foote, J. Am. Chem. Soc., 1993, 115, 8867; CrossRef (b) V. J. Colandrea, S. Rajaraman, and L. S. Jimenez, Org. Lett., 2003, 5, 785; CrossRef (c) W. Zhang, C. Wang, and L. S. Jimenez, Synth. Commun., 2000, 30, 351. CrossRef
15. (a) R. M. Williams, T. Glinka, E. Kwast, H. Coffman, and J. K. Stille, J. Am. Chem. Soc., 1990, 112, 808; CrossRef (b) P. S. Baran and E. J. Corey, J. Am. Chem. Soc., 2002, 124, 7904; CrossRef (c) A. J. Hutchison and Y. Kishi, J. Am. Chem. Soc., 1979, 101, 6786; CrossRef (d) R. M. Williams, J. F. Sanz-Cervera, F. Sancenón, J. A. Marco, and K. Halligan, J. Am. Chem. Soc., 1998, 120, 1090; CrossRef (e) T. Hino, H. Yamaguchi, K. Matsuki, K. Nakano, M. Sodeoka, and M. Nakagawa, J. Chem. Soc., Perkin Trans. 1, 1983, 141; CrossRef (f) D. M. Harrison, Tetrahedron Lett., 1984, 25, 6063; CrossRef (g) A. S. Bourlot, E. Desarbre, and J. Y. Mérour, Synthesis, 1994, 411; CrossRef (h) R. W. Clawson, C. A. Dacko, R. E. Deavers III, N. G. Akhmedov, and B. C. G. Söderberg, Tetrahedron, 2009, 65, 8786. CrossRef
16. J. Stachel, M. Nilges, and D. L. Van Vranken, J. Org. Chem., 1997, 62, 4756. CrossRef
17. M. C. Hewitt and L. Shao, ARKIVOC, 2006, xi, 37S..
18. I. Mutule, E. Suna, K. Olofsson, and B. Pelcman, J. Org. Chem., 2009, 74, 7195. CrossRef
19. (a) O. R. Suárez-Castillo, M. Sánchez-Zavala, M. Meléndez-Rodríguez, L. E. Castelán-Duarte, M. S. Morales-Ríos, and P. Joseph-Nathan, Tetrahedron, 2006, 62, 3040; CrossRef (b) O. R. Suárez-Castillo, M. Sánchez-Zavala, M. Meléndez-Rodríguez, E. Aquino-Torres, M. S. Morales-Ríos, and P. Joseph-Nathan, Heterocycles, 2007, 71, 1539; CrossRef (c) O. R. Suárez-Castillo, C. I. Bautista-Hernández, M. Sánchez-Zavala, M. Meléndez-Rodríguez, A. Sierra-Zenteno, M. S. Morales-Ríos, and P. Joseph-Nathan, Heterocycles, 2012, 85, 2147. CrossRef
20. (a) M. A. Seefeld, W. H. Miller, K. A. Newlander, W. J. Burgess, W. E. DeWolf Jr., P. A. Elkins, M. S. Head, D. R. Jakas, C. A. Janson, P. M. Keller, P. J. Manley, T. D. Moore, D. J. Payne, S. Pearson, B. J. Polizzi, X. Qiu, S. F. Rittenhouse, I. N. Uzinskas, N. G. Wallis, and W. F. Huffman, J. Med. Chem., 2003, 46, 1627; CrossRef (b) L. Zhu, P. Guo, G. Li, J. Lan, R. Xie, and J. You, J. Org. Chem., 2007, 72, 8535; CrossRef (c) A. R. Katritzky and K. Akutagawa, J. Am. Chem. Soc., 1986, 108, 6808. CrossRef
21. S. Mithani, D. M. Drew, E. H. Rydberg, N. J. Taylor, S. Mooibroek, and G. I. Dmitrienko, J. Am. Chem. Soc., 1997, 119, 1159. CrossRef
22. G. Zhou, J. Zhu, Z. Xie, and Y. Li, Org. Lett., 2008, 10, 721. CrossRef
23. L. Fitjer, R. Gerke, W. Lüttke, P. Müller, and I. Usón, Tetrahedron, 1999, 55, 14421. CrossRef
24. Computer package Spartan04, Windows v 1.0.1; Wavefunction Inc.: Irvine, CA, USA, 2004.
25. (a) H. E. Gottlieb, V. Kotlyar, and A. Nudelman, J. Org. Chem., 1997, 62, 7512; CrossRef (b) G. R. Fulmer, A. J. M. Miller, N. H. Sherden, H. E. Gottlieb, A. Nudelman, B. M. Stoltz, J. E. Bercaw, and K. I. Goldber, Organometallics, 2010, 29, 2176. CrossRef
26. Y. Sato, H. Kojima, and H. Shirai, J. Org. Chem., 1976, 41, 195. CrossRef
27. Aldrich/ACD library of FT NMR spectra pro, v 1.00; Advanced Chemistry Development, Inc. and Aldrich Chemical Company, Inc., Toronto, Canada and Milwaukee, USA, 1998.
28. S. Storelli, D. Verzijl, J. Al-Badie, N. Elders, L. Bosch, H. Timmerman, M. J. Smit, I. J. P. De Esch, and R. Leurs, Arch. Parm. Chem. Life Sci., 2007, 340, 281. CrossRef
29. G. M. Sheldrick, ‘Programs for Crystal Structure Analysis,’ Institüt für Anorganische Chemie der Universität; University of Göttingen: Göttingen, Germany, 1988.
30. L. J. Farrugia, J. Appl. Crystallogr., 1999, 32, 837. CrossRef