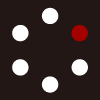
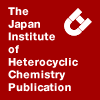
HETEROCYCLES
An International Journal for Reviews and Communications in Heterocyclic ChemistryWeb Edition ISSN: 1881-0942
Published online by The Japan Institute of Heterocyclic Chemistry
e-Journal
Full Text HTML
Received, 24th April, 2013, Accepted, 23rd May, 2013, Published online, 24th May, 2013.
DOI: 10.3987/COM-13-S(S)8
■ Two-Step Synthesis of 3,4-Ethylenedioxythiophene (EDOT) from 2,3-Butanedione
Iwao Hachiya, Toshihiro Yamamoto, Tatsuhiko Inagaki, Tomohiro Matsumoto, Atsushi Takahashi, Isao Mizota, and Makoto Shimizu*
Department of Chemistry for Materials, Graduate School of Engineering, Mie University, 1577 Kurimamachiya-cho Tsu, Mie 514-8507, Japan
Abstract
3,4-Ethylenedioxythiophene (EDOT) was synthesized from commercially available 2,3-butanedione in only a two-step procedure including ketalization and thiophene formation.Poly(3,4-ethylenedioxythiophene) (PEDOT) prepared by polymerization of 3,4-ethylenedioxythiophene (EDOT (1)) and its derivatives are one of the most successful electron-conducting polymers and widely used as antistatic treatment of plastics and electrode materials for solid state electrolyte capacitors.1 Several methods for the synthesis of EDOT (1) have been reported using the double Williamson etherification, 2 Mitsunobu reaction,3 transetherification,4 and zirconocene dichloride mediated reaction.5 We have already reported a synthetic route toward EDOT (1) from commercially available (Z)-but-2-ene-1,4-diol (2) or but-2-yne-1,4-diol (3) using epoxidation, etherification, and thiophene formation.6 The characteristics of the synthetic route were relatively mild reaction conditions such as without use of carcinogenic reagents and heavy metals. However, it needed eight or nine steps for the synthesis of EDOT (1) from each starting material (Scheme 1).
In the course of our efforts for the efficient synthesis of EDOT (1), we focused on commercially available 2,3-butanedione (4) as a starting material. Herein, we describe a two-step synthesis of EDOT (1) from commercially available 2,3-butanedione (4).
Scheme 2 shows a retrosynthetic analysis of EDOT (1): EDOT (1) could be synthesized by thiophene formation of 2,3-dimethylene-1,4-dioxane (5), which could be obtained by dienol bisetherification of commercially available 2,3-butanedione (4).
We first examined the synthesis of 2,3-dimethylene-1,4-dioxane (5) using 1,2-dibromoethane (6). The reactions of 2,3-butanedione (4) with 1,2-dibromoethane (6) were carried out in the presence of p-toluenesulfonic acid, NaHMDS, or triethylamine; however, 2,3-dimethylene-1,4-dioxane (5) was not obtained (Eq. 1).
We next examined the synthesis of 2,3-dimethylene-1,4-dioxane (5) using ethylene glycol (7) instead of 1,2-dibromoethane (6). The reaction of 2,3-butanedione (4) with ethylene glycol (7) and trimethyl orthoformate (8) in MeOH was carried out in the presence of a catalytic amount of camphorsulfonic acid (CSA) under reflux to give 2,3-dimethoxy-2,3-dimethyl-1,4-dioxane (9) in 95% yield instead of the desired 2,3-dimethylene-1,4-dioxane (5) (Eq. 2).7
We therefore planned the synthesis of EDOT (1) by trapping of 2,3-dimethylene-1,4-dioxane (5) generated in situ from 2,3-dimethoxy-2,3-dimethyl-1,4-dioxane (9) with a sulfur source (Scheme 3).
Komatsu reported that a facile [4+1] type synthetic route to thiophene (11) from dienol bissilyl ether (10) and elemental sulfur (Eq. 3).8 Therefore, we chose S8 as a sulfur source for the synthesis of EDOT (1).
The reactions of 2,3-dimethoxy-2,3-dimethyl-1,4-dioxane (9) with S8 were carried out in the presence of several additives such as CSA, Pd/C, palladium black, palladium salts, copper salts, and molecular sieves 4A (MS 4A); however, EDOT (1) was obtained in yields ranging from 0 to 15% (Eq. 4).
We next examined the synthesis of EDOT (1) under microwave irradiation to improve the yield. Table 1 summarizes the results. When the reaction was carried out under microwave irradiation in toluene at 180 oC, EDOT (1) was not obtained (entry 1). Several additives were examined. Among additives tested, CuI was found to be efficient (entries 2-12). Effects of concentration, temperature, and reaction time were next investigated. When the reaction was carried out in the presence of CuI (0.1 equiv) at 180 oC for 6 h, EDOT (1) was obtained in 37% yield (entry 12).
A plausible reaction mechanism for the formation of EDOT (1) is shown in Scheme 4 which refers to that reported by Komatsu.8 2,3-Dimethylene-1,4-dioxane (5) would be generated from 2,3-dimethoxy-2,3-dimethyl-1,4-dioxane (9) along with elimination of MeOH. Nucleophilic reaction of 2,3-dimethylene-1,4-dioxane (5) to S8 would occur to give the ring-opening intermediate (12). Intramolecular cyclization of the intermediate (12) would give the 1,2-dithiine intermediate (13) along with elimination of S6, and the subsequent elimination of H2S gives EDOT (1). Although the role of CuI as an additive is not yet clear, we presume that it acts as a mild Lewis acid catalyst for the elimination of MeOH and ring-opening of S8.
In conclusion, we have developed a two-step synthesis of EDOT (1) from commercially available 2,3-butanedione (4). Although the yield of EDOT (1) was moderate, the present method provides a very short-step and easy access to several EDOT derivatives using substituted 1,2-diols instead of ethylene glycol (7), and the overall yield is good. Furthermore, the application of the process to the industrial synthesis of EDOT (1) may be possible by an efficient heating device.
EXPERIMENTAL
General. Reactions under microwave irradiation were carried out using a Biotage Initiator. Infrared spectra were recorded on a JASCO FT/IR-460 Plus spectrometer. 1H NMR spectra were recorded on a JEOL JNM α-500 spectrometer (500 MHz) with tetramethylsilane as an internal standard. 13C NMR spectra were recorded on a JEOL JNM α-500 spectrometer (126 MHz). Chemical sifts are reported in δ units, parts per million from the central peak of CDCl3 (δ 77.0) as an internal reference. High resolution mass spectra (EI) were recorded on a JEOL JMS-700D mass spectrometer. MeOH was distilled from magnesium methoxide and stored over molecular sieves 3A. Toluene was pre-dried with CaCl2, distilled, and stored over molecular sieves 4A. Purification of products was performed by column chromatography on silica gel (Kanto Chemical Co. Inc., Silica Gel 60 N (spherical, neutral)) and/or preparative TLC on silica gel (Merck Kiesel Gel GF254).
Synthesis of 2,3-Dimethoxy-2,3-dimethyl-1,4-dioxane (9):
To a solution of ethylene glycol (7) (7.88 g, 127 mmol) in MeOH (63 mL) was added 2,3-butanedione (4) (10.0 g, 116 mmol), trimethyl orthoformate (8) (29.6 g, 279 mmol), and camphorsulfonic acid (2.93 g, 12.6 mmol) at room temperature under an argon atmosphere. The mixture was stirred at reflux for 24 h. Triethylamine (1.9 mL) was added to the reaction mixture to quench the reaction. The mixture was diluted with Et2O and washed with water, sat. aqueous NaHCO3, and brine, dried over anhydrous Na2SO4, and filtered. The solvents were evaporated in vacuo and then the residue was purified by chromatography on silica gel (n-Hex/EtOAc = 1/1, as an eluent) to give 2,3-dimethoxy-2,3-dimethyl-1,4-dioxane (9) (19.4 g, 95%) as a yellow oil.
1H NMR (500 MHz, CDCl3) δ = 3.95 (dd, J = 12.2, 19.5 Hz, 2H), 3.43 (dd, J = 12.2, 19.5 Hz, 2H), 3.31 (s, 6H), 1.29 (s, 6H). 13C NMR (126 MHz, CDCl3) δ = 98.5, 58.9, 48.1, 17.8. IR (neat) 2954, 2831, 1449, 1373, 1308, 1264, 1269, 1141, 1084, 1037, 941, 918, 871, 829 cm-1. HRMS (EI): Calculated for C8H16O4 (M)+ 176.1049, found 176.1050.
Synthesis of EDOT (1)9 (Table 1, entry 12):
S8 (640 mg, 2.5 mmol), 2,3-dimethoxy-2,3-dimethyl-1,4-dioxane (9) (88 mg, 0.50 mmol), toluene (5.0 mL), CuI (9.5 mg, 0.050 mmol), and a magnetic stirring bar were placed in a microwave reactor vial. The vial was capped, placed in the microwave reactor, and irradiated at 180 oC for 6 h. The mixture was cooled to room temperature, diluted with EtOAc, and filtered through a Celite pad. The solvents were evaporated in vacuo and then the residue was purified by preparative TLC on silica gel (developed twice with n- Hex and once with n-Hex/EtOAc = 8/1) to give EDOT (1) (26.0 mg, 37%) as a yellow oil.
1H NMR (500 MHz, CDCl3) δ = 6.32 (s, 2H), 4.20 (s, 4H). 13C NMR (126 MHz, CDCl3) δ = 141.8, 99.6, 64.7. IR (neat) 3111, 2974, 2924, 2872, 1488, 1449, 1422, 1236, 1274, 1186, 1057, 932, 893, 761 cm-1.
References
1. For a review, see: L. Groenendaal, F. Jonas, D. Freitag, H. Pielartzik, and J. R. Reynolds, Adv. Mater., 2000, 12, 481 and references therein. CrossRef
2. Q. Pei, G. Zuccarello, M. Ahlskog, and O. Inganäs, Polymer, 1994, 35, 1347. CrossRef
3. (a) K. Zong, L. Madrigal, L. Groenendaal, and J. R. Reynolds, Chem. Commun., 2002, 2498; CrossRef (b) D. Caras-Quintero and P. Bäuerle, Chem. Commun., 2002, 2690. CrossRef
4. Short step synthesis of EDOT via p-TsOH catalyzed transetherification of 3,4-dimethoxythiophene with ethylene glycol, see: (a) F. von Kieseritzky, F. Allared, E. Dahlstedt, and J. Hellberg, Tetrahedron Lett., 2004, 45, 6049; CrossRef (b) S. Chen, B. Lu, X. Duan, and J. Xu, J. Polym. Sci. Part A: Polym. Chem., 2012, 50, 1967. CrossRef
5. S. Das, P. K. Dutta, S. Panda, and S. S. Zade, J. Org. Chem., 2010, 75, 4868. CrossRef
6. I. Hachiya, T. Matsumoto, T. Inagaki, A. Takahashi, and M. Shimizu, Heterocycles, 2010, 82, 449. CrossRef
7. For a review on 1,2-diacetals, see: S. V. Ley, D. K. Baeschlin, D. J. Dixon, A. C. Foster, S. J. Ince, H. W. M. Priepke, and D. J. Reynolds, Chem. Rev., 2001, 101, 53; CrossRef For examples, see: (b) N. Mariet, M. Ibrahim-Ouali, and M. Santelli, Tetrahedron Lett., 2003, 44, 5315; CrossRef (c) S. V. Ley, E. Diez, D. J. Dixon, R. T. Guy, P. Michel, G. L. Nattrass, and T. D. Sheppard, Org. Biomol. Chem., 2004, 2, 3608. CrossRef
8. Y. Kasano, A. Okada, D. Hiratsuka, Y. Oderaotoshi, S. Minakata, and M. Komatsu, Tetrahedron, 2006, 62, 537. CrossRef
9. 1H NMR, 13C NMR, and IR spectra of the synthetic and the commercially available EDOT (1) were identical.