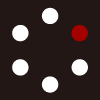
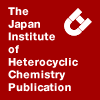
HETEROCYCLES
An International Journal for Reviews and Communications in Heterocyclic ChemistryWeb Edition ISSN: 1881-0942
Published online by The Japan Institute of Heterocyclic Chemistry
e-Journal
Full Text HTML
Received, 15th May, 2013, Accepted, 4th June, 2013, Published online, 10th June, 2013.
DOI: 10.3987/COM-13-S(S)13
■ Palladium-Catalyzed Direct Arylation and Alkenylation of 3-(Indol-3-yl)propionic Acids through C–H Bond Cleavage
Daisuke Takeda, Koji Hirano, Tetsuya Satoh,* and Masahiro Miura*
Department of Applied Chemistry, Graduate School of Engineering, Osaka University, 2-1 Yamadaoka, Suita, Osaka 565-0871, Japan
Abstract
The palladium-catalyzed direct arylation of 3-(indol-3-yl)propionic acids with aryl bromides proceeds through C–H bond cleavages to give C2-arylated product in good yields. The C2-alkenylation of the substrates can also be performed smoothly under appropriate oxidative conditions.INTRODUCTION
Indole structures possessing various substituents can be seen in a great number of biologically active natural and unnatural compounds, and thus, their selective syntheses are of considerable importance in organic synthesis field.1 Among others, 3-(2-substituted indol-3-yl)propionic acid derivatives are of particular interest due to their broad biological activities as well as tangible potential as neurokinin 1 (NK1) antagonists and antioxidants.2 Therefore, the development of synthetic methods for C2-functionalization of the indole moiety of 3-(indol-3-yl)propionic acids is of substantial interest. Meanwhile, the transition-metal-catalyzed direct functionalization reactions of (hetero)aromatic compounds via C–H bond cleavage have extensively studied in recent years due to their atom- and step-economical advantages over the conventional cross-coupling strategies.3 We have investigated the direct arylation, alkenylation, and annulation of heteroaromatics including indoles.4 In the context of our work, we demonstrated that (indol-3-yl)acetic acids undergo C2-arylation upon treatment with aryl bromides in the presence of a palladium catalyst.4a,5 The carboxylic function appears to act as an effective director for the reaction. During a further study, it was found that the C2-arylation of 3-(indol-3-yl)-propionic acids can be achieved effectively by using a similar catalytic system. Moreover, the substrates also underwent C2-alkenylation with alkenes in the presence of an appropriate oxidant.6 These new findings are described herein.
RESULTS AND DISCUSSION
In an initial attempt, 3-(1-methylindol-3-yl)propionic acid (1a, 0.4 mmol) was treated with bromobenzene (2a, 0.8 mmol) in the presence of Pd(OAc)2 (0.02 mmol), CyJohnPhos (2-(dicyclohexylphosphino)- biphenyl, 0.04 mmol), and K2CO3 (0.9 mmol) as catalyst, ligand, and base, respectively, in DMAc at 170 oC for 9 h. After treatment with MeI (6 mmol) and K2CO3 (3 mmol) at room temperature for quantification, methyl 3-(1-methyl-2-phenylindol-3-yl)propionate (3a) was obtained in 80% yield (Table 1, Entry 1). Both increase and decrease in the amount of 2a did not enhance the product yield (Entries 2
and 3). A comparable result was found to be obtained within 3 h (Entry 4 vs 1). Although the use of other bases such as Na2CO3, Cs2CO3, KOBut, KOAc, and KH2PO4 in place of K2CO3 decreased the product yield (Entries 5-9), KHCO3 and K3PO4 were found to be as effective as K2CO3 (Entries 10 and 11). Next, the reaction was conducted with various mono- and diphosphine ligands using KHCO3 as base (Entries 12-19). Among the ligands examined, XPhos (2-(dicyclohexylphosphino)-2’,4’,6’-triisopropylbiphenyl) was found to be the most effective (Entry 15). Thus, with XPhos (0.04 mmol), 3a was obtained in 98% yield.
Under the optimized reaction conditions (Table 1, Entry 15), the reactions of a series of 4-substituted bromobenzenes 2b-f with 1a were next examined (Table 2, Entries 1-7). While 4-bromotoluene (2b) reacted with 1a smoothly (Entry 1), the reaction of 4-bromoanisole (2c) was somewhat sluggish (Entry 2). By increasing the amount of KHCO3 (1.5 mmol) and extending reaction time (20 h), product 3c was obtained in 97% yield (Entry 4). Under similar conditions, 4-chloro- (2d), 4-fluoro- (2e), and 4-trifluoromethyl- (2f) bromobenzenes also underwent the reaction with 1a efficiently to produce the corresponding methyl 3-(1-methyl-2-arylindol-3-yl)propionates 3d-f in good yields (Entries 5-7). 3-(1-Phenylindol-3-yl)propionic acid (1b) also reacted with 2a to afford methyl 3-(1,2-diphenylindol-3-yl)propionate (3g) (Entry 8). In contrast, 3-(1-unsubstituted indol-3-yl)propionic acid (1c) underwent phenylation on N1 rather than C2 upon treatment with 2a (Scheme 1).
The present arylation of 1 with 2 appears to proceed through similar reaction pathways to those of the direct arylation of indole derivatives.4a,7 It should be noted that treatment of methyl 3-(1-methylindol-3-yl)propionate (1d) with 2a gave 3a only in a moderate yield (Scheme 2). Therefore, the existence of the carboxylic group in 1 appears to promote the reaction at least partly.
Next, we examined the C2-alkenylation of 3-(indol-3-yl)propionic acid.8 Treatment of 1a (0.4 mmol) with butyl acrylate (4a, 0.8 mmol) in the presence of Pd(OAc)2 (0.02 mmol), Cu(OAc)2•H2O (0.8 mmol), and LiOAc (1.2 mmol) as catalyst, oxidant, and additive, respectively, in DMF at 140 oC for 6 h and subsequent esterification with MeI gave (E)-butyl 3-[3-(3-methoxy-3-oxopropyl)-1-methyl-1H-indol-2- yl]acrylate (5a) in 21% yield (Table 3, Entry 1).
Increasing the reaction temperature to 170 oC in DMAc improved the yield of 5a (Entry 2). The optimization of the amount of Cu(OAc)2•H2O (1.2 mmol) led to further enhancement of the yield of 5a up to 54% (Entries 3 and 4). Even in these cases, significant amounts of 1a were recovered (ca. 20%). At a relatively high temperature, palladium active species tend to be deactivated.9 Other oxidants such as Cu(2-ethylhexylCO2)2, AgOAc, and Ag2CO3 were found to be less effective (Entries 5-7). The addition of LiOAc was essential to conduct the reaction smoothly (Entry 8). While comparable results were obtained with NaOAc and KOAc (Entries 9 and 10), CsOAc, Li2CO3, and KHCO3 were less effective as an additive (Entries 11-13). The alkenylation did not proceeded at all in the presence of a Ru- or Rh-catalyst in place of the Pd catalyst (Entries 14 and 15), although all of them have been employed as catalysts in the C2-alkenylation of indole-3-carboxylic acids.4b,c,p
The reactions of 1a with various alkenes 4 were conducted under the conditions used for Entry 3 in Table 3. The corresponding C2-alkenylated products 5b-d were obtained by using isobutyl (4b) and cyclohexyl (4c) acrylates and N,N-dimethylacrylamide (4d) (Table 4, Entries 1-3). As observed in the arylation, the existence of the carboxylic group was essential for conducting the alkenylation smoothly. Thus, treatment of 1d with 4a gave 5a in a lower yield (Entry 4).
In summary, we have demonstrated that the C2-arylation and alkenylation of 3-(indol-3-yl)propionic acids can occur through C–H bond cleavage under palladium catalysis. The relatively more remote carboxylic group in the substrates appears to be still capable of promoting the reactions.
EXPERIMENTAL
General. 1H and 13C NMR spectra were recorded at 400 and 100 MHz for CDCl3 solutions. MS data were obtained by EI. GC analysis was carried out using a silicon OV-17 column (i. d. 2.6 mm x 1.5 m). GC-MS analysis was carried out using a CBP-1 capillary column (i. d. 0.25 mm x 25 m). The structures of all products listed below were unambiguously determined by 1H and 13C NMR with the aid of NOE, COSY, HMQC, and HMBC experiments.
Starting Materials. Indole-3-propionic acids 1a,10 1b,11 and 1d10 were prepared according to published procedures. Other starting materials were commercially available.
Typical Procedure for the Reactions of Indole-3-propionic Acids 1 with Aryl Bromides 2. A mixture of indole-3-propionic acid 1 (0.4 mmol), aryl bromide 2 (0.8 mmol), Pd(OAc)2 (0.02 mmol, 4.5 mg), XPhos (0.04 mmol, 19 mg), KHCO3 (0.9-1.5 mmol), and dibenzyl (ca. 40 mg) as internal standard was stirred in DMAc (2.5 mL) under nitrogen at 170 oC for 6-20 h. After the mixture was cooled, iodomethane (6 mmol, 852 mg) and K2CO3 (3 mmol, 415 mg) were added, and the resulting mixture was stirred under air at room temperature for 3 h. GC and GC-MS analyses of the mixtures confirmed formation of product 3. The reaction mixture was extracted with EtOAc (100 mL). The organic layer was
washed with water (100 mL, three times), and dried over Na2SO4. After evaporation of the solvent under vacuum, the product 3 was isolated by column chromatography on silica gel using hexane-EtOAc as eluant.
Typical Procedure for the Reactions of Indole-3-propionic Acids 1 with Alkenes 4. A mixture of indole-3-propionic acid 1 (0.4 mmol), alkene 4 (0.8 mmol), Pd(OAc)2 (0.02 mmol, 4.5 mg), Cu(OAc)2・H2O (1.2 mmol), LiOAc (1.2 mmol), and dibenzyl (ca. 40 mg) as internal standard was stirred in DMAc (2.5 mL) under nitrogen at 170 oC for 6 h. After the mixture was cooled, iodomethane (6 mmol, 852 mg) and K2CO3 (3 mmol, 415 mg) were added, and the resulting mixture was stirred under air at room temperature for 3 h. GC and GC-MS analyses of the mixtures confirmed formation of product 5. The reaction mixture was extracted with EtOAc (100 mL). The organic layer was washed with water (100 mL, three times), and dried over Na2SO4. After evaporation of the solvent under vacuum, the product 5 was isolated by column chromatography on silica gel using hexane-EtOAc as eluant and gel permeation chromatography using chloroform as eluant.
Characterization Data of Products.
Methyl 3-(1-Methyl-2-phenyl-1H-indol-3-yl)propanoate (3a): oil; 1H NMR (400 MHz, CDCl3) δ 2.56-2.60 (m, 2H), 3.03-3.07 (m, 2H), 3.57 (s, 3H), 3.60 (s, 3H), 7.14-7.18 (m, 1H), 7.24-7.28 (m, 1H), 7.33-7.38 (m, 3H), 7.42-7.51 (m, 3H), 7.64 (d, J = 7.8 Hz, 1H); 13C NMR (100 MHz, CDCl3) δ 20.2, 30.7, 35.4, 51.5, 109.4, 111.4, 118.7, 119.3, 121.8, 127.2, 128.2, 128.5, 130.5, 131.7, 137.0, 138.1, 173.7; HRMS m/z (M+) Calcd for C19H19NO2: 293.1416. Found 293.1417.
Methyl 3-(1-Methyl-2-(4-methylphenyl)-1H-indol-3-yl)propanoate (3b): mp 117-119 oC; 1H NMR (400 MHz, CDCl3) δ 2.44 (s, 3H), 2.55-2.60 (m, 2H), 3.02-3.06 (m, 2H), 3.56 (s, 3H), 3.61 (s, 3H), 7.13-7.17 (m, 1H), 7.23-7.34 (m, 6H), 7.62 (d, J = 7.8 Hz, 1H); 13C NMR (100 MHz, CDCl3) δ 20.3, 21.3, 30.7, 35.5, 51.5, 109.4, 111.2, 118.7, 119.2, 121.6, 127.2, 128.7, 129.2, 130.4, 137.0, 138.0, 138.2, 173.8; HRMS m/z (M+) Calcd for C20H21NO2: 307.1572. Found 307.1570.
Methyl 3-(2-(4-Methoxyphenyl)-1-methyl-1H-indol-3-yl)propanoate (3c): mp 90-92 oC; 1H NMR (400 MHz, CDCl3) δ 2.57 (t, J = 8.2 Hz, 2H), 3.03 (t, J = 8.0 Hz, 2H), 3.55 (s, 3H), 3.61 (s, 3H), 3.88 (s, 3H), 7.01-7.03 (m, 2H), 7.14 (t, J = 7.6 Hz, 1H), 7.22-7.33 (m, 4H), 7.62 (d, J = 7.8 Hz, 1H); 13C NMR (100 MHz, CDCl3) δ 20.3, 30.6, 35.4, 51.5, 55.3, 109.3, 111.1, 114.0, 118.6, 119.2, 121.6, 123.9, 127.2, 131.7, 136.9, 138.0, 159.5, 173.8; HRMS m/z (M+) Calcd for C20H21NO3: 323.1521. Found 323.1523.
Methyl 3-(2-(4-Chlorophenyl)-1-methyl-1H-indol-3-yl)propanoate (3d): mp 142-144 oC; 1H NMR (400 MHz, CDCl3) δ 2.57 (t, J = 8.0 Hz, 2H), 3.03 (t, J = 8.0 Hz, 2H), 3.56 (s, 3H), 3.61 (s, 3H), 7.16 (t, J = 7.4 Hz, 1H), 7.27-7.35 (m, 4H), 7.48 (d, J = 8.2 Hz, 2H), 7.63 (d, J = 7.8 Hz, 1H); 13C NMR (100 MHz, CDCl3) δ 20.2, 30.7, 35.3, 51.5, 109.5, 111.8, 118.8, 119.5, 122.1, 127.1, 128.8, 130.2, 131.8, 134.4, 136.7, 137.2, 173.5; HRMS m/z (M+) Calcd for C19H18ClNO2: 327.1026. Found 327.1023.
Methyl 3-(2-(4-Fluorophenyl)-1-methyl-1H-indol-3-yl)propanoate (3e): oil; 1H NMR (400 MHz, CDCl3) δ 2.55-2.59 (m, 2H), 3.00-3.04 (m, 2H), 3.54 (s, 3H), 3.61 (s, 3H), 7.14-7.22 (m, 3H), 7.24-7.28 (m, 1H), 7.32-7.36 (m, 3H), 7.63 (d, J = 7.8 Hz, 1H); 13C NMR (100 MHz, CDCl3) δ 20.2, 30.7, 35.3, 51.5, 109.4, 111.6, 115.6 (d, J = 22.1 Hz), 118.8, 119.4, 121.9, 127.1, 127.7 (d, J = 2.9 Hz), 132.3 (d, J = 7.7 Hz), 136.95, 137.01, 162.7 (d, J = 247.2 Hz), 173.6; HRMS m/z (M+) Calcd for C19H18FNO2: 311.1322. Found 311.1322.
Methyl 3-(1-Methyl-2-(4-(trifluoromethyl)phenyl)-1H-indol-3-yl)propanoate (3f): mp 114-116 oC; 1H NMR (400 MHz, CDCl3) δ 2.59 (t, J = 8.0 Hz, 2H), 3.05 (t, J = 8.2 Hz, 2H), 3.58 (s, 3H), 3.60 (s, 3H), 7.16-7.20 (m, 1H), 7.27-7.31 (m, 1H), 7.36 (d, J = 8.2 Hz, 1H), 7.52 (d, J = 8.2 Hz, 2H), 7.65 (d, J = 8.3 Hz, 1H), 7.76 (d, J = 7.8 Hz, 2H); 13C NMR (100 MHz, CDCl3) δ 20.2, 30.9, 35.3, 51.6, 109.6, 112.4, 119.0, 119.7, 122.4, 125.5 (q, J =3.8 Hz), 126.8 (q, J = 273.2 Hz), 127.1, 130.2 (q, J =32.6 Hz), 130.9, 135.5, 136.4, 137.4, 173.5; HRMS m/z (M+) Calcd for C20H18F3NO2: 361.1290. Found 361.1287.
Methyl 3-(1,2-Diphenyl-1H-indol-3-yl)propanoate (3g): mp 85-87 oC; 1H NMR (400 MHz, CDCl3) δ 2.68 (t, J = 8.2 Hz, 2H), 3.19 (t, J = 8.2 Hz, 2H), 3.64 (s, 3H), 7.15-7.34 (m, 13H), 7.68-7.71 (m, 1H); 13C NMR (100 MHz, CDCl3) δ 20.3, 35.2, 51.6, 110.6, 113.3, 118.8, 120.2, 122.5, 126.8, 127.5, 127.7, 127.9, 128.1, 129.0, 130.5, 131.7, 137.47, 137.54, 138.2, 173.6; HRMS m/z (M+) Calcd for C24H21NO2: 355.1572. Found 355.1574.
Methyl 3-(1-Phenyl-1H-indol-3-yl)propanoate (3a’): oil; 1H NMR (400 MHz, CDCl3) δ 2.27 (t, J = 7.8 Hz, 2H), 3.16 (t, J = 7.8 Hz, 2H), 3.69 (s, 3H), 7.16-7.25 (m, 3H), 7.31-7.34 (m, 1H), 7.46-7.52 (m, 4H), 7.56 (d, J = 8.3 Hz, 1H), 7.65 (d, J = 7.3Hz, 1H); 13C NMR (100 MHz, CDCl3) δ 20.5, 34.6, 51.7, 110.6, 116.0, 119.0, 120.0, 122.5, 124.1, 125.3, 126.2, 128.6, 129.6, 136.0, 139.8, 173.8; HRMS m/z (M+) Calcd for C18H17NO2: 279.1259. Found 279.1260.
(E)-Butyl 3-(3-(3-Methoxy-3-oxopropyl)-1-methyl-1H-indol-2-yl)acrylate (5a): oil; 1H NMR (400 MHz, CDCl3) δ 0.98 (t, J = 7.3 Hz, 3H), 1.46 (qt, J = 7.5, 7.4 Hz, 2H), 1.72 (tt, J = 7.3, 7.1 Hz, 2H), 2.66 (t, J = 7.8 Hz, 2H), 3.27 (t, J = 7.8 Hz, 2H), 3.67 (s, 3H), 3.82 (s, 3H), 4.24 (t, J = 6.6 Hz, 2H), 6.31 (d, J = 16.5 Hz, 1H), 7.10-7.14 (m, 1H), 7.29 (d, J = 3.7 Hz, 2H), 7.62 (d, J = 7.8 Hz, 1H), 7.88 (d, J = 16.0 Hz, 1H); 13C NMR (100 MHz, CDCl3) δ 13.8, 19.2, 20.4, 30.8, 31.2, 35.0, 51.7, 64.6, 109.6, 118.0, 119.0, 119.6, 119.9, 124.3, 127.0, 131.1, 132.3, 138.8, 167.3, 173.2; HRMS m/z (M+) Calcd for C20H25NO4: 343.1784. Found 343.1784.
(E)-Isobutyl 3-(3-(3-Methoxy-3-oxopropyl)-1-methyl-1H-indol-2-yl)acrylate (5b): oil; 1H NMR (400 MHz, CDCl3) δ 1.01 (d, J = 6.9 Hz, 6H), 2.04 (tqq, J = 6.9, 6.7, 6.7 Hz, 1H), 2.66 (t, J = 7.8 Hz, 2H), 3.27 (t, J = 7.8 Hz, 2H), 3.67 (s, 3H), 3.83 (s, 3H), 4.03 (d, J = 6.9 Hz, 2H), 6.32 (d, J = 16.0 Hz, 1H), 7.11-7.15 (m, 1H), 7.30 (d, J = 3.7 Hz, 2H), 7.63 (d, J = 7.8 Hz, 1H), 7.89 (d, J = 16.5 Hz, 1H); 13C NMR (100 MHz, CDCl3) δ 19.2, 20.4, 27.8, 31.3, 35.0, 51.7, 70.8, 109.6, 118.0, 119.1, 119.6, 119.9, 124.3, 127.0, 131.2, 132.3, 138.8, 167.3, 173.2; HRMS m/z (M+) Calcd for C20H25NO4: 343.1784. Found 343.1782.
(E)-Cyclohexyl 3-(3-(3-Methoxy-3-oxopropyl)-1-methyl-1H-indol-2-yl)acrylate (5c): oil; 1H NMR (400 MHz, CDCl3) δ 1.26-1.61 (m, 6H), 1.78-1.95 (m, 4H), 2.66 (t, J = 8.0 Hz, 2H), 3.27 (t, J = 7.8 Hz, 2H), 3.67 (s, 3H), 3.83 (s, 3H), 4.89-4.94 (m, 1H), 6.29 (d, J = 16.0 Hz, 1H), 7.10-7.14 (m, 1H), 7.30 (d, J = 3.7 Hz, 2H), 7.62 (d, J = 7.8 Hz, 1H), 7.87 (d, J = 16.0 Hz, 1H); 13C NMR (100 MHz, CDCl3) δ 20.4, 23.9, 25.4, 31.3, 31.8, 35.0, 51.7, 73.0, 109.5, 118.6, 118.9, 119.6, 119.8, 124.2, 127.0, 131.2, 132.1, 138.8, 166.6, 173.2; HRMS m/z (M+) Calcd for C22H27NO4: 369.1940. Found 369.1943.
(E)-Methyl 3-(2-(3-(Dimethylamino)-3-oxoprop-1-en-1-yl)-1-methyl-1H-indol-3-yl)propanoate (5d): mp 94-96 oC; 1H NMR (400 MHz, CDCl3) δ 2.64-2.68 (m, 2H), 3.11 (s, 3H), 3.21 (s, 3H), 3.26-3.30 (m, 2H), 3.68 (s, 3H), 3.81 (s, 3H), 6.85 (d, J = 15.6 Hz, 1H), 7.10-7.14 (m, 1H), 7.26-7.29 (m, 2H), 7.61 (d, J = 8.2 Hz, 1H), 7.87 (d, J = 15.6 Hz, 1H); 13C NMR (100 MHz, CDCl3) δ 21.0, 30.7, 34.8, 36.1, 37.3, 51.7, 109.5, 116.4, 118.6, 119.2, 119.7, 123.6, 127.2, 130.4, 132.2, 138.0, 166.5, 173.4; HRMS m/z (M+) Calcd for C18H22N2O3: 314.1630. Found 314.1632.
ACKNOWLEDGEMENTS
This work was partly supported by Grants-in-Aid from MEXT, JSPS, and JST, Japan.
References
1. Selected examples: (a) G. R. Humphrey and J. T. Kuethe, Chem. Rev., 2006, 106, 2875; CrossRef (b) D. A. Horton, G. T. Bourne, and M. L. Smythe, Chem. Rev., 2003, 103, 893; CrossRef (c) B. E. Evans, K. E. Rittle, M. G. Bock, R. M. DiPardo, R. M. Freidinger, W. L. Whitter, G. F. Lundell, D. F. Verber, P. S. Anderson, R. S. L. Chang, V. J. Lotti, D. H. Cerino, T. B. Chen, P. J. Kling, K. A. Kunkel, J. P. Springer, and J. Hirshfield, J. Med. Chem., 1988, 31, 2235. CrossRef
2. Selected examples: (a) R. Takasawa, A. Tao, K. Saeki, N. Shionozaki, R. Tanaka, H. Uchiro, S. Takahashi, A. Yoshimori, and S.-I. Tanuma, Bioorg. Med. Chem. Lett., 2011, 21, 4337; CrossRef (b) M. S. Estevão, L. C. Carvalho, D. Ribeiro, D. Couto, M. Freitas, A. Gomes, L. M. Ferreira, E. Fernandes, and M. M. B. Marques, Eur. J. Med. Chem., 2010, 45, 4869; CrossRef (c) C. A. Willoughby, S. M. Hutchins, K. G. Rosauer, M. J. Dhar, K. T. Chapman, G. G. Chicchi, S. Sadowski, D. H. Weinberg, S. Patel, L. Malkowitz, J. Di Salvo, S. G. Pacholok, and K. Cheng, Bioorg. Med. Chem. Lett., 2002, 12, 93; CrossRef (d) D. Shaw, G. G. Chicchi, J. M. Elliott, M. Kurtz, D. Morrison, M. P. Ridgill, N. Szeto, A. P. Watt, A. R. Williams, and C. J. Swain, Bioorg. Med. Chem. Lett., 2001, 11, 3031; CrossRef (e) L. C. Cooper, G. G. Chicchi, K. Dinnell, J. M. Elliott, G. J. Hollingworth, M. M. Kurtz, K. L. Locker, D. Morrison, D. E. Shaw, K.-L. Tsao, A. P. Watt, A. R. Williams, and C. J. Swain, Bioorg. Med. Chem. Lett., 2001, 11, 1233; CrossRef (f) B. Poeggeler, M. A. Pappolla, R. Hardeland, A. Rassoulpour, P. S. Hodgkins, P. Guidetti, and R. Schwarcz, Brain Res., 1999, 815, 382. CrossRef
3. Selected reviews: (a) D. A. Colby, A. S. Tsai, R. G. Bergman, and J. A. Ellman, J. A. Acc. Chem. Res., 2012, 45, 814; CrossRef (b) K. M. Engle, T.-S. Mei, M. Wasa, and J.-Q. Yu, Acc. Chem. Res., 2012, 45, 788; CrossRef (c) E. A. Mitchell, A. Peschiulli, N. Lefevre, L. Meerpoel, and B. U. W. Maes, Chem. Eur. J., 2012, 18, 10092; CrossRef (d) S. H. Cho, J. Y. Kim, J. Kwak, and S. Chang, Chem. Soc. Rev., 2011, 40, 5068; CrossRef (e) J. Wencel-Delord, T. Droge, F. Liu, and F. Glorius, Chem. Soc. Rev., 2011, 40, 4740; CrossRef (f) Y. Kuninobu and K. Takai, Chem. Rev., 2011, 111, 1938; CrossRef (g) C. Liu, H. Zhang, W. Shi, and A. Lei, Chem. Rev., 2011, 111, 1780; CrossRef (h) L. Ackermann, Chem. Rev., 2011, 111, 1315; CrossRef (i) J. Roger, A. L. Gottumukkala, and H. Doucet, ChemCatChem, 2010, 2, 20; CrossRef (j) T. W. Lyons and M. S. Sanford, Chem. Rev., 2010, 110, 1147; CrossRef (k) D. A. Colby, R. G. Bergman, and J. A. Ellman, Chem. Rev., 2010, 110, 624; CrossRef (l) C.-L. Sun, B.-J. Li, and Z.-J. Shi, Chem. Commun., 2010, 46, 677; CrossRef (m) L. Ackermann, R. Vicente, and A. R. Kapdi, Angew. Chem. Int. Ed., 2009, 48, 9792; CrossRef (n) X. Chen, K. M. Engle, D.-H. Wang, and J.-Q. Yu, Angew. Chem. Int. Ed., 2009, 48, 5094; CrossRef (o) O. Daugulis, H.-Q. Do, and D. Shabashov, Acc. Chem. Res., 2009, 42, 1074; CrossRef (p) G. P. McGlacken and L. M. Bateman, Chem. Soc. Rev., 2009, 38, 2447; CrossRef (q) F. Bellina and R. Rossi, Tetrahedron, 2009, 65, 10269; CrossRef (r) F. Kakiuchi and T. Kochi, Synthesis, 2008, 3013; CrossRef (s) Y. J. Park, J.-W. Park, and C.-H. Jun, Acc. Chem. Res., 2008, 41, 222; CrossRef (t) A. Mori and A. Sugie, Bull. Chem. Soc. Jpn., 2008, 81, 548; CrossRef (u) E. M. Beccalli, G. Broggini, M. Martinelli, and S. Sottocornola, Chem. Rev., 2007, 107, 5318; CrossRef (v) D. Alberico, M. E. Scott, and M. Lautens, Chem. Rev., 2007, 107, 174; CrossRef (w) I. V. Seregin and V. Gevorgyan, Chem. Soc. Rev., 2007, 36, 1173; CrossRef (x) K. Godula and D. Sames, Science, 2006, 312, 67; CrossRef (y) F. Kakiuchi and N. Chatani, Adv. Synth. Catal., 2003, 345, 1077; CrossRef (z) G. Dyker, Angew. Chem. Int. Ed., 1999, 38, 1698. CrossRef
4. (a) D. Takeda, M. Yamashita, K. Hirano, T. Satoh, and M. Miura, Chem. Lett., 2011 40, 1015; CrossRef (b) S. Mochida, K. Hirano, T. Satoh, and M. Miura, J. Org. Chem., 2011, 76, 3024; CrossRef (c) T. Ueyama, S. Mochida, T. Fukutani, K. Hirano, T. Satoh, and M. Miura, Org. Lett., 2011, 13, 706; CrossRef (d) M. Kitahara, N. Umeda, K. Hirano, T. Satoh, and M. Miura, J. Am. Chem. Soc., 2011, 133, 2160; CrossRef (e) N. Umeda, K. Hirano, T. Satoh, N. Shibata, H. Sato, and M. Miura, J. Org. Chem., 2011, 76, 13; CrossRef (f) M. Miyasaka, K. Hirano, T. Satoh, and M. Miura, J. Org. Chem., 2010, 75, 5421; CrossRef (g) K. Morimoto, K. Hirano, T. Satoh, and M. Miura, Org. Lett., 2010, 12, 2068; CrossRef (h) H. Hachiya, K. Hirano, T. Satoh, and M. Miura, Angew. Chem. Int. Ed., 2010, 49, 2202; CrossRef (i) M. Miyasaka, K. Hirano, T. Satoh, and M. Miura, Adv. Synth. Catal., 2009, 351, 2683; CrossRef (j) M. Yamashita, K. Hirano, T. Satoh, and M. Miura, J. Org. Chem., 2009, 74, 7481; CrossRef (k) M. Yamashita, K. Hirano, T. Satoh, and M. Miura, Org. Lett., 2009, 11, 2337; CrossRef (l) H. Hachiya, K. Hirano, T. Satoh, and M. Miura, Org. Lett., 2009, 11, 1737; CrossRef (m) T. Yoshizumi, T. Satoh, K. Hirano, D. Matsuo, A. Orita, J. Otera, and M. Miura, Tetrahedron Lett., 2009, 50, 3273; CrossRef (n) M. Miyasaka, A. Fukushima, T. Satoh, K. Hirano, and M. Miura, Chem. Eur. J., 2009, 15, 3674; CrossRef (o) M. Nakano, H. Tsurugi, T. Satoh, and M. Miura, Org. Lett., 2008, 10, 1851; CrossRef (p) A. Maehara, H. Tsurugi, T. Satoh, and M. Miura, Org. Lett., 2008, 10, 1159; CrossRef (q) T. Yoshizumi, H. Tsurugi, T. Satoh, and M. Miura, Tetrahedron Lett., 2008, 49, 1598; CrossRef (r) A. Maehara, T. Satoh, and M. Miura, Tetrahedron, 2008, 64, 5982; CrossRef (s) M. Nakano, T. Satoh, and M. Miura, J. Org. Chem., 2006, 71, 8309; CrossRef (t) A. Yokooji, T. Satoh, M. Miura, and M. Nomura, Tetrahedron, 2004, 60, 6757; CrossRef (u) A. Yokooji, T. Okazawa, T. Satoh, M. Miura, and M. Nomura, Tetrahedron, 2003, 59, 5685; CrossRef (v) T. Okazawa, T. Satoh, M. Miura, and M. Nomura, J. Am. Chem. Soc., 2002, 124, 5286; CrossRef (w) S. Pivsa-Art, T. Satoh, Y. Kawamura, M. Miura, and M. Nomura, Bull. Chem. Soc. Jpn., 1998, 71, 467; CrossRef For reviews, see: (x) K. Hirano and M. Miura, Synlett, 2011, 294; CrossRef (y) T. Satoh and M. Miura, Chem. Eur. J., 2010, 16, 11212; CrossRef (z) T. Satoh and M. Miura, Chem. Lett., 2007, 36, 200. CrossRef
5. For ortho-arylation of phenylacetic acids, see: (a) K. M. Engle, P. S. Thuy-Boun, M. Dang, and J.-Q. Yu, J. Am. Chem. Soc., 2011, 133, 18183; CrossRef (b) D.-H. Wang, T.-S. Mei, and J.-Q. Yu, J. Am. Chem. Soc., 2008, 130, 17676. CrossRef
6. For ortho-alkenylation of phenylacetic acids, see: (a) K. M. Engle, D.-H. Wang, and J.-Q. Yu, Angew. Chem. Int. Ed., 2010, 49, 6169; CrossRef (b) B. F. Shi, Y. H. Zhang, J. K. Lam, D.-H. Wang, and J.-Q. Yu, J. Am. Chem. Soc., 2010, 132, 460; CrossRef (c) D.-H. Wang, K. M. Engle, B. F. Shi, and J.-Q. Yu, Science, 2010, 327, 315. CrossRef
7. Selected examples for C2-arylation of indoles: (a) E. T. Nadres, A. Lazareva, and O. Daugulis, J. Org. Chem., 2011, 76, 471; CrossRef (b) S. Potavathri, K. C. Pereira, S. I. Gorelsky, A. Pike, A. P. LeBris, and B. DeBoef, J. Am. Chem. Soc., 2010, 132, 14676; CrossRef (c) B. Liéegault, I. Petrov, S. I. Gorelsky, and K. Fagnou, J. Org. Chem., 2010, 75, 1047; CrossRef (d) R. J. Phipps, N. P. Grimster, and M. J. Gaunt, J. Am. Chem. Soc., 2008, 130, 8172; CrossRef (e) N. Lebrasseur and I. Larrosa, J. Am. Chem. Soc., 2008, 130, 2926; CrossRef (f) X. Wang, D. V. Gribkov, and D. Sames, J. Org. Chem., 2007, 72, 1476; CrossRef (g) N. R. Deprez, D. Kalyani, A. Krause, and M. S. Sanford, J. Am. Chem. Soc., 2006, 128, 4972. CrossRef
8. Selected examples for C2-alkenylation of indoles: (a) A. García-Rubia, R. G. Arrayás, and J. C. Carretero, Angew. Chem. Int. Ed., 2009, 48, 6511; CrossRef (b) N. P. Grimster, C. Gauntlett, C. R. A. Godfrey, and M. J. Gaunt, Angew. Chem. Int. Ed., 2005, 44, 3125. CrossRef
9. S. S. Stahl, Angew. Chem. Int. Ed., 2004, 43, 3400. CrossRef
10. C. Prandi, E. G. Occhiato, S. Tabasso, P. Bonfante, M. Novero, D. Scarpi, M. E. Bova, and I. Miletto, Eur. J. Org. Chem., 2011, 3781. CrossRef
11. J. C. Antilla, A. Klapars, and S. L. Buchwald, J. Am. Chem. Soc., 2002, 124, 11684. CrossRef