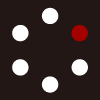
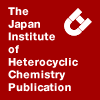
HETEROCYCLES
An International Journal for Reviews and Communications in Heterocyclic ChemistryWeb Edition ISSN: 1881-0942
Published online by The Japan Institute of Heterocyclic Chemistry
e-Journal
Full Text HTML
Received, 29th April, 2013, Accepted, 27th May, 2013, Published online, 5th June, 2013.
DOI: 10.3987/COM-13-S(S)10
■ Regioselectivity of the Intramolecular Biaryl Coupling Reaction of 3-Substituted Phenyl 2-Iodobenzoate using a Palladium Reagent
Kazuhiro Maeda, Takuya Matsukihira, Shumpei Saga, Yasuo Takeuchi,* Takashi Harayama, Yoshikazu Horino, and Hitoshi Abe*
Department of Environmental Applied Chemistry, Faculty of Engineering, Toyama University, Gofuku 3190, Toyama 930-8555, Japan
Abstract
This study investigated the regioselectivity of the intramolecular biaryl coupling reaction of the phenyl benzoate derivative which possesses a methyl or methoxy group at the meta-position of the phenoxy moiety. The type of base and the presence/absence of the phosphine ligand influenced the product ratio. A transition state model and the regioselectivity of the reaction are discussed.The 6H-dibenzo[b,d]pyran-6-one skeleton is an important heterocyclic system because many natural products and biologically active compounds involve this ring system.1 Among the various preparations for this ring system,2 the palladium-mediated aryl-aryl coupling reaction is one of the most convenient techniques for the carbon-carbon bond formation between two aromatic rings.3 We recently reported that the intramolecular aryl-aryl coupling reaction of phenyl benzoate derivatives using the palladium reagent is widely useful for the syntheses of several biaryl-type natural products.4 In some cases, the regioselectivity has been a significant issue for the natural product synthesis, however, there are a few examples of the systematic study of the regioselectivity of the intramolecular aryl-aryl coupling reaction.5 As shown in Scheme 1, when the substrate possesses a substituent at the m-position as 1, there are two reactive positions on the phenoxy part to generate two regioisomers 2 and 3.
In this report, we investigated in detail the reactivity and regioselectivity of the palladium-mediated coupling reaction of m-substituted phenyl benzoate derivatives.
We first examined the substrate 1a which possesses a methoxy group at the m-position in the phenoxy part. The results are summarized in Table 1. In each case, all the reactions were completed in 30 min using Pd(OAc)2 (10 mol %), base (100 mol %), ligand (20 mol %), and DMA (N,N-dimethylacetamide) as the solvent. We employed several bases for the reaction in the presence/absence of nBu3P as the ligand.
When using K2CO3 as the base without the phosphine ligand, the reaction proceeded with a slight regioselectivity (2a:3a = 1:2.0) (entry 1). Employing nBu3P under the same reaction conditions, the regioselectivity changed to 1:1.4 (entry 2), which was almost the same ratio compared to the result for entry 1. A similar outcome was also observed for entries 3-6, namely, the regioselectivity was in the range of 1:1.2 to 2.0 for each case whether the phosphine ligand was present or absent. When DABCO (diazabicyclo[2.2.2]octane) was used as the base, the chemical yield decreased to around 40% with the ratios of 1:3.5 and 1:2.0 (entries 7 and 8).
In entries 9 and 10, we evaluated Ag2CO3 as the base to investigate the effect of a metal ion. The major product was compound 3a with the regioselectivity of 1:4.5 when using no phosphine ligand (entry 9). In sharp contrast to this result, employing nBu3P lead to a dramatic change in the regioselectivity (2a:3a = 1:0.5) (entry 10). The same tendency was found for entries 11-14, when using Ag2O or AgOAc as the base. CuOAc was also examined as the base to investigate the difference in the type of the metal. Entries 15 and 16 showed results similar to that of silver.
Next, in order to investigate the coordination effect of the methoxy group, we employed a methyl-substituted compound 1b. In Table 2, very different results from the methoxy derivative 1a were observed, that is, the coupling reaction proceeded with a poor regioselectivity. It was also found that using the phosphine ligand did not affect the product ratio (entries 1 and 2). In spite of changing the base from K2CO3 to Ag2CO3, a low regioselectivity was observed (entries 3 and 4). These results indicated that the methoxy group in 1a has a significant effect on the regioselectivity.
Based on the above results, we would be able to provide a reasonable explanation for the regioselectivity of the intramolecular coupling reaction by considering transition state models. In 2007, Echavarren et al. proposed the intermolecular-assisted mechanism for the Pd-mediated aryl-aryl coupling reaction.6 Based on their model, we illustrate the transition stage in our substrates (Figure 1). Model A describes the reaction under the condition of using K2CO3, Na2CO3, or AcOK as the base. In this case, there is no predominant difference between A1 and A2 to control the reaction path, leading to an almost equal ratio of products 2 and 3. On the other hand, when using the silver salt, the soft character of the metal would be crucial by contributing an attractive interaction with the iodine atom. This strong attractive property between iodine and silver causes a partially positive charge on the palladium atom, which makes coordination of the methoxy group easier. Thus, the B1 transition state, which exhibits a tight interaction of O-Pd, must be more preferable than B2. This coordination effect is also supported by the result of entry 4 in Table 2, where the product ratio of 2b and 3b is 1:1.
Contrary to the above model, it is necessary to consider a different transition state when using no phosphine ligand. As depicted in C1 and C2, DMA molecules, which are employed as the solvent, would possibly coordinate around the palladium atom. Since the DMA molecules would be much bulkier than the phosphine ligand, the coordination between the methoxy group and the palladium atom cannot be developed. Besides, a severe electrostatic repulsion of the methoxy group and the Pd-solvent complex leads to produce compound 3a as the major isomer.
In summary, we systematically investigated the regioselectivity of the intramolecular coupling reaction of 3-substituted phenyl 2-iodobenzoates using a Pd catalyst. Based on the product ratio, plausible transition state models were proposed. Further application of this work is now ongoing in our laboratory.
EXPERIMENTAL
General: Melting points were measured using a Yanagimoto micro-melting point hot-plate apparatus and are uncorrected. The IR spectra were recorded using a JASCO FTIR-350 spectrophotometer. The NMR spectra were obtained using a Varian MERCURY-300
instrument with the chemical shifts being reported as δ ppm and the couplings expressed in Hertz. The elemental analysis was performed using a Yanaco MT-5 analyzer. Silica gel column chromatography was carried out using Daisogel 1002W or Merck 9385 Kieselgel 60. All reactions were carried out under an argon atmosphere.
3-Methoxy-6H-dibenzo[b,d]pyran-6-one (3a),7 1-methyl-6H-dibenzo[b,d]pyran-6-one (2b),8 and 3-methyl-6H-dibenzo[b,d]pyran-6-one (3b)9 are known compounds.
3-Methoxyphenyl 2-iodobenzoate (1a)
A mixture of SOCl2 (8 mL), 2-iodobenzoic acid (4.96 g, 20.0 mmol), and 2 drops of DMF was heated under reflux. After 1 h, the excess SOCl2 was removed under reduced pressure to give a brown residue which was diluted with CH2Cl2. To the solution of the obtained acid chloride, a mixture of 3-methoxyphenol (2.48 g, 2.16 mL, 20 mmol), Et3N (3.34 mL, 24.0 mmol), and CH2Cl2 (10 mL) was added, and then the mixture was stirred for 3 h at rt. The reaction mixture was poured into water and extracted with CH2Cl2. The organic layer was washed with brine, dried over MgSO4, and evaporated to give a crude residue, which was subjected to silica gel column chromatography using AcOEt : hexane = 1:6. Colorless 1a (6.32 g, 89%) was obtained in a pure form.
Colorless needles, mp 54.1—55.0 oC (Et2O-hexane). IR (KBr) cm-1:1750, 1240. 1H-NMR(300 MHz, CDCl3) δ: 3.83 (s, 3H, OCH3), 6.81—6.89 (m, 3H), 7.23 (m, 1H), 7.34 (ddd, 1H, J1=7.8 Hz, J2=7.8 Hz, J3=0.9 Hz), 7.48 (ddd, 1H, J1=7.8 Hz, J2=7.8 Hz, J3=1.6 Hz), 8.04 (dd, 1H, J1=7.8 Hz, J2=1.2 Hz), 8.07 (dd, 1H, J1= 7.8 Hz, J2=0.9 Hz). 13C-NMR(75 MHz, CDCl3)δ: 55.7, 94.8, 107.8, 112.2, 114.0, 128.3, 130.1, 131.7, 133.4, 134.3, 141.8, 151.8, 160.7, 165.0. Anal. Calcd for C14H11IO3, C, 47.48; H, 3.13; Found: C, 47.67; H, 3.02.
3-Methylphenyl 2-iodobenzoate (1b)
A mixture of SOCl2 (4 mL), 2-iodobenzoic acid (2.48 g, 10.0 mmol), and 2 drops of DMF was heated under reflux. After 1 h, the excess SOCl2 was removed under reduced pressure to give a brown residue which was diluted with CH2Cl2. To the solution of the obtained acid chloride, a mixture of 3-methylphenol (1.08 g, 1.05 mL, 10 mmol), Et3N (1.66 mL, 12.0 mmol), and CH2Cl2 (5 mL) was added, and then the mixture was stirred for 3 h at rt. The reaction mixture was poured into water and extracted with CH2Cl2. The organic layer was washed with brine, dried over MgSO4, and evaporated to give a crude residue, which was subjected to silica gel column chromatography using AcOEt : hexane = 1:6. Colorless 1b (2.77 g, 82%) was obtained in a pure form.
Colorless needles, mp 41.4−42.6 oC (Et2O-hexane). IR (KBr) cm-1:1740, 1230. 1H-NMR(300 MHz, CDCl3) δ: 2.40 (s, 3H), 7.05−7.11 (m, 3H), 7.20−7.35 (m, 2H), 7.48 (ddd, 1H, J1=7.5 Hz, J2=7.5 Hz, J3=1.2 Hz), 8.03 (dd, 1H, J1=7.8 Hz, J2=1.8 Hz), 8.07 (dd, 1H, J1=8.1 Hz, J2=1.2 Hz). 13C-NMR(75 MHz, CDCl3)δ: 21.5, 94.8, 118.7, 122.3, 127.1, 128.2, 129.4, 131.7, 133.3, 134.4, 139.9, 141.8, 150.79, 165.2. MS (FAB, positive ion mode):339 (M+1+). Anal. Calcd for C14H11IO2, C, 49.73; H, 3.28; Found: C, 49.76; H, 3.10.
General Procedure of Coupling Reaction of 1a
To a solution of 1a (88.5 mg, 0.25 mmol) in DMA (3 mL), Pd(OAc)2, base, and additive were successively added. The mixture was heated for 30 min under reflux, and then diluted with EtOAc. After filtration, the mixture was poured into water and extracted with EtOAc. The organic layer was washed with brine, dried over MgSO4, and evaporated to give a crude residue which was subjected to silica gel column chromatography using AcOEt : hexane = 1:4 and CH2Cl2 : hexane = 1:1 as the eluent. Colorless crystals of 2a and 3a were separately obtained in a pure form.
1-Methoxy-6H-dibenzo[b,d]pyran-6-one (2a)
Colorless needles, mp 164.2−166.1 ℃ (AcOEt-hexane). IR (KBr) cm-1: 1740, 1260. 1H-NMR(300 MHz, CDCl3) δ: 4.00 (s, 3H), 6.80 (m, 1H), 6.95 (m, 1H), 7.33 (m, 1H), 7.49 (m, 1H), 7.73 (m, 1H), 8.37 (m, 1H), 8.91 (m, 1H). 13C-NMR(75 MHz, CDCl3) δ: 56.0, 106.9, 108.2, 110.4, 120.9, 127.6, 128.03, 129.9, 130.1, 134.6, 134.7, 152.6, 158.4, 161.5. Anal. Calcd for C14H10O3, C, 74.33; H, 4.46; Found: C, 74.18; H, 4.42.
General Procedure of Coupling Reaction of 1b
To a solution of 1b (84.5 mg, 0.25 mmol) in DMA (3 mL), Pd(OAc)2, base, and additive were successively added. The mixture was heated for 30 min under reflux, and then diluted with EtOAc. After filtration, the mixture was poured into water and extracted with EtOAc. The organic layer was washed with brine, dried over MgSO4, and evaporated to give a crude residue which was subjected to silica gel column chromatography using AcOEt : hexane = 1:4 as the eluent. Inseparable mixture of 2b and 3b was obtained. All efforts to separate these compounds were unsuccessful. The product ratio was determined by 1H-NMR analysis based on the integration of 1-methyl and 3-methyl groups of 2b and 3b, respectively. 2b: δ 2.89 (s, 3H). 3b: δ 2.50 (s, 3H). These chemical shifts were referred to the already reported compounds.2d, 2e, 4b, 4d
ACKNOWLEDGEMENTS
A part of this research was financially supported by the JSPS KAKENHI Grant. No. 22590003 to H. A. The authors thank the SC-NMR Laboratory of Okayama University for the NMR experiments.
References
1. (a) H.-W. Zhang, W.-Y. Huang, Y.-C. Song, J.-R. Chen, and R.-X. Tan, Helv. Chim. Acta, 2005, 88, 2861; CrossRef (b) T. Tanahashi, M. Kuroishi, A. Kuwahara, N. Nagakura, and N. Hamada, Chem. Pharm. Bull., 1997, 45, 1183; CrossRef (c) N. Hamada, T. Tanahashi, S. Goldsmith, and T. H. Nash III, Symbiosis, 1997, 23, 219; (d) T. Tanahashi, Y. Takenaka, N. Nagakura, and N. Hamada, Phytochemistry, 2003, 62, 71; CrossRef (e) E. Hormazabal, G. Schmeda-Hirschmann, L. Astudillo, J. Rodriguez, and C. Theoduloz, Z. Naturforsch, 2005, 60c, 11; (f) Y.-C. Song, W.-Y. Huang, C. Sun, F.-W. Wang, and R.-X. Tan, Biol. Pharm. Bull., 2005, 28, 506; CrossRef (g) T. Matsumoto, T. Hosoya, and H. Shigemori, Heterocycles, 2010, 81, 1231. CrossRef
2. (a) For a pioneering work on the synthesis of 6H-dibenzo[b,d]pyran-6-one, see: B. I. Alo, A. Kandil, P. A. Patil, M. J. Sharp, M. A. Siddiqui, V. Snieckus, and P. D. Josephy, J. Org. Chem., 1991, 56, 3763; CrossRef (b) R. Singha, S. Roy, S. Nandi, P. Ray, and J. K. Ray, Tetrahedron Lett., 2013, 54, 657; CrossRef (c) C.-L. Sun, J. Liu, Y. Wang, X. Zhou, B.-J. Li, and Z.-J. Shi, Synlett, 2011, 883; CrossRef (d) J. Cudaj and J. Podlech, Tetrahedron Lett., 2010, 51, 3092; CrossRef (e) M. Altemöller, T. Gehring, J. Cudaj, J. Podlech, H. Goesmann, C. Feldmann, and A. Rothenberger, Eur. J. Org. Chem., 2009, 2130; CrossRef (f) C. A. James and V. Snieckus, Tetrahedron Lett., 1997, 38, 8149; CrossRef (g) J. Luo, Y. Lu, S. Liu, J. Liu, and G.-J. Deng, Adv. Synth. Catal., 2011, 353, 2604; CrossRef (h) I. R. Pottie, P. R. Nandaluru, W. L. Benoit, D. O. Miller, L. N. Dawe, and G. J. Bodwell, J. Org. Chem., 2011, 76, 9015; CrossRef (i) K. Koch, J. Podlech, E. Pfeiffer, and M. Metzler, J. Org. Chem., 2005, 70, 3275; CrossRef (j) G. A. Molander, K. M. George, and L. G. Monovich, J. Org. Chem., 2003, 68, 9533. CrossRef
3. For reviews, see, (a) J. Yamaguchi, A. D. Yamaguchi, and K. Itami, Angew. Chem. Int. Ed., 2012, 51, 8960; CrossRef (b) D. Alberico, M. E. Scott, and M. Lautens, Chem. Rev., 2007, 107, 174; CrossRef (c) J. Hassan, M. Sévignon, C. Gozzi, E. Schulz, and M. Lemaire, Chem. Rev., 2002, 102, 1359; CrossRef (d) G. Bringmann, T. Gulder, T. A. M. Gulder, and M. Breuning, Chem. Rev., 2011, 111, 563; CrossRef (e) G. Bringmann, M. Breuning, and S. Tasler, Synthesis, 1999, 525; CrossRef (f) G. Bringmann, A. J. P. Mortimer, P. A. Keller, M. J. Gresser, J. Garner, and M. Breuning, Angew. Chem. Int. Ed., 2005, 44, 5384; CrossRef (g) H. Abe and T. Harayama, Heterocycles, 2008, 75, 1305. CrossRef
4. (a) H. Abe, T. Kawai, Y. Komatsu, M. Kamimura, Y. Takeuchi, and Y. Horino, Heterocycles, 2012, 86, 785; CrossRef (b) H. Abe, T. Fukumoto, Y. Horino, T. Harayama, and Y. Takeuchi, Heterocycles, 2010, 82, 851; CrossRef (c) H. Abe, N. Kobayashi, Y. Takeuchi, and T. Harayama, Heterocycles, 2010, 80, 873; CrossRef (d) H. Abe, T. Fukumoto, Y. Takeuchi, and T. Harayama, Heterocycles, 2007, 74, 265; CrossRef (e) H. Abe, T. Fukumoto, K. Nishioka, M. Arai, Y. Takeuchi, and T. Harayama, Heterocycles, 2006, 69, 217; CrossRef (f) H. Abe, K. Nishioka, S. Takeda, M. Arai, Y. Takeuchi, and T. Harayama, Tetrahedron Lett., 2005, 46, 3197; CrossRef (g) S. Takeda, H. Abe, Y. Takeuchi, and T. Harayama, Tetrahedron, 2007, 63, 396; CrossRef (h) H. Abe, S. Takeda, T. Fujita, K. Nishioka, Y. Takeuchi, and T. Harayama, Tetrahedron Lett., 2004, 45, 2327. CrossRef
5. (a) R. Bernini, S. Cacchi, G. Fabrizi, and A. Sferrazza, Synthesis, 2008, 729; CrossRef (b) T. Harayama, Y. Kawata, C. Nagura, T. Sato, T. Miyagoe, H. Abe, and Y. Takeuchi, Tetrahedron Lett., 2005, 46, 6091; CrossRef (c) T. Harayama, C. Nagura, T. Miyagoe, Y. Kawata, H. Abe, and Y. Takeuchi, Heterocycles, 2010, 81, 2609; CrossRef (d) H. Nishioka, C. Nagura, H. Abe, Y. Takeuchi, and T. Harayama, Heterocycles, 2006, 70, 549; CrossRef (e) T. Harayama, M. Asai, T. Miyagoe, H. Abe, Y. Takeuchi, A. Yamaguchi, and S. Fujii, Heterocycles, 2010, 81, 1881. CrossRef
6. (a) D. Garcia-Cuadrado, P. de Mendoza, A. A. C. Braga, F. Maseras, and A. M. Echavarren, J. Am. Chem. Soc., 2007, 129, 6880; CrossRef (b) D. Garcia-Cuadrado, A. A. C. Braga, F. Maseras, and A. M. Echavarren, J. Am. Chem. Soc., 2006, 128, 1066; CrossRef see also, (c) M. Lafrance, D. Lapointe, and K. Fagnou, Tetrahedron, 2008, 64, 6015; CrossRef (d) L.-C. Campeau, M. Parisien, M. Leblanc, and K. Fagnou, J. Am. Chem. Soc., 2004, 126, 9186; CrossRef (e) S. I. Gorelsky, Coord. Chem. Rev., 2013, 257, 153; CrossRef (f) S. Pascual, P. deMendoza, A. A. C. Braga, F. Maseras, and A. M. Echavarren, Tetrahedron, 2008, 64, 6021. CrossRef
7. D. J. Hart, A. Kim, R. Krishnamurthy, G. H. Merriman, and A.-M. Waltos, Tetrahedron, 1992, 48, 8179. CrossRef
8. C. Grundmann and E. Litten, Chem. Ber., 1952, 85, 261. CrossRef
9. U. Kraatz and F. Korte, Chem. Ber., 1973, 106, 62. CrossRef