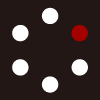
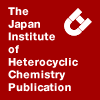
HETEROCYCLES
An International Journal for Reviews and Communications in Heterocyclic ChemistryWeb Edition ISSN: 1881-0942
Published online by The Japan Institute of Heterocyclic Chemistry
e-Journal
Full Text HTML
Received, 19th June, 2013, Accepted, 25th July, 2013, Published online, 29th July, 2013.
DOI: 10.3987/COM-13-S(S)38
■ Synthesis of 8-Amino-(dihydrofuran-Fused Perhydrophenanthrene) via Copper-Mediated Amination Reaction
Kenji Sugimoto, Kosuke Tamura, Naoki Toyooka, and Yuji Matsuya*
Graduate School of Medicine and Pharmaceutical Sciences for Research, University of Toyama, Sugitani 2630, Toyama, 930-0194, Japan
Abstract
Introduction of nitrogen substituents on a highly functionalized dihydrofuran-fused perhydrophenanthrene (DF) core was examined. Acetamide functionality could be introduced on the DF by copper-mediated Buchwald-type coupling. On the other hand, installation of free amino group on a DF was established via an aryl azide under the copper-mediated condition reported by Helquist and co-workers.Naturally occurring biologically active pentacyclic polyketides such as halenaquinone, halenaquinol, and xestoquinone were known to possess furan-fused highly oxygenated structure.1 Their structural and biological feature attracted synthetic organic chemists2 and we also developed an effective preparation of similar tetracyclic dihydrofurans (DFs) using originally elaborated o-quinodimethane chemistry.3 The biological evaluations on our DFs revealed that they show several fascinating biological activities. Firstly, dihydrofuran 1 was proved to exhibit anti-virus activity against HVJ.4 This dihydrofuran 1 also showed dose-dependent enhancement of a heat-induced apoptosis in human lymphoma U937 cells.5 Further structure-activity-relationship studies afforded potent anti-influenza agents, trifluoromethyl ether 2 and 3,5-difluorobenzyl ether 3.6 Additionally, 4 has been expected as novel anti-Alzheimer’s disease agent for its axonal and dendritic extension activities in Aβ-damaged neurons.7 Recently, extensive SAR studies on 4 suggested the efficiency of the introduction of hetero atom on the C8 position and finally proved that EtO- (5) or PhS- (6) substituent markedly enhance the dendritic extension effect.8 However, we could never have estimated the dendritic extension activities of nitrogen-introduced analogues for its difficulty in installing nitrogen functionalities on the common precursor in our previous report.8 Thus, the importance of the hetero atoms on the C8 position of DFs prompted us to develop a reaction which enable the late-stage introduction of amino group on DFs. We describe herein the synthesis of 8-amino-DFs 7, which could be a pivotal intermediate for further SAR studies, via copper-mediated amination reaction.
Prior to the synthetic studies, we examined a Cu-mediated introduction of N-methylacetamide on a simple model substrate, o-iodophenols under the conditions reported by Buchwald and co-workers (Table 1).9,10 The coupling reaction of o-(TBSO)-iodobenzene (8a) with N-methylacetamide (2 eq) was conducted in the presence of CuI (1 eq), DMEDA (2 eq) and K3PO4 (2 eq), at 100 °C in DMSO to give a complex mixture including o-iodophenol (8b) as a detectable product (entry 1). Dehalogenation reaction dominantly proceeded with o-iodophenol (8b) as a substrate (entry 2). On the other hand, o-(p-methoxybenzyloxy)-iodobenzene (8c) afforded corresponding acylanilide 9c in 7% yield (entry 3). The addition of (±)-trans-1,2-cyclohexanediamine as a ligand slightly improve the yield of 9c to 20% (entry 4).
With these results, we examined an installation of N-acetyl-N-methyl amino group on our dihydrofuran-fused perhydrophenanthrene 108 as depicted in Scheme 1. Phenolic hydroxyl group was uneventfully protected as DMB ether 11, which could be cleaved under a milder condition than that for PMB ether. Cu-mediated coupling between 11 and N-methylacetamide took place to afford the corresponding anilide 12 in 18% yield accompanied with inevitable decomposition of the substrate including dehalogenation reaction.
Aiming at the synthesis and biological evaluation of broad nitrogen-substituted DFs, next we examined a preparation of the pivotal free aniline derivative. As indicated in Table 2, Cu-mediated amination reaction with NaN3, which was reported by Helquist and co-workers,11 was employed on o-iodophenols. Although, similar to the amidation reaction, unprotected phenolic hydroxyl group would prevent the desired amination reaction (entry 1), o-(p-methoxybenzyloxy)-iodobenzene worked well to provide the aniline 13c in 40% yield (entry 2). Finally, it was found that 8c was effectively transformed into the desired 13c with 52% in the presence of the excess amount of NaN3 (entry 4).
Under the optimal condition, DF 11 could be converted into desired aniline 14, pivotal aniline congener for further derivatization, in good yield (66%) as denoted in Scheme 2.
Finally, cleavage of the DMB group on the anilide 12 was performed as Scheme 3. Under quite mild condition such as DDQ in basic media, DF 15 was successfully obtained in 58% remaining the acid labile, acetal and dihydrofuran functional groups untouched.
In summary, we investigated the late-stage introduction of amino group into biologically potent DF core and could fortunately establish the key aniline derivatives in good yield based on Cu-mediated amination strategy. Further derivatization from the common aniline 14 and biological evaluation of the nitrogen-substituents on DFs are now in progress in our laboratory.
EXPERIMENTAL
Materials were obtained from commercial suppliers and used without further purification. Column chromatography was performed on Cica Silica Gel 60 N (spherical, neutral, 40–50 µm or 63–210 µm). Reaction and chromatographical fractions were monitored by employing precorted silica gel 60 F254 plates (Merck). All melting points were determined on Yanaco micro melting point apparatus and uncorrected. NMR spectra were recorded on JEOL ECX 400 spectrometer with CHCl3 (7.26 ppm for 1H) or CDCl3 (77.0 ppm for 13C) as an internal standard. Chemical shifts are expressed in δ (ppm) values, and coupling constants are expressed in hertz (Hz). The following abbreviations are used: s = singlet, d = doublet, t = triplet, q = quartet, m = multiplet, br s = broad singlet, dd = double-doublet, dt = double-triplet, td = triple-doublet, tt = triple-triplet, ddd = double-double-doublet, dddd = double-double-double-doublet. IR spectra were measured on JASCO FT/IR-660. MS spectra were recorded on JEOL D-200, JEOL JMS-GCmateII or JEOL AX 505 spectrometer.
General Procedure for Cu-mediated Amidation Reaction of Aryl Iodide (Table 1).
Under an Ar atmosphere, to a solution of the aryl iodide 8c (100 µmol) in dioxane (0.2 M) were added N-methylacetamide (2 eq), CuI (1 eq), ligand (2 eq), and K3PO4 (2 eq) at rt, and the mixture was stirred at 100 °C until the starting material was completely consumed (monitored by TLC). The reaction mixture was then cooled to rt, diluted with saturated aqueous NH4Cl and AcOEt, and stirred for 40 min at rt. After filtration through Celite, the aqueous phase was extracted with AcOEt. The combined organic layers were dried over MgSO4, filtered, and concentrated in vacuo. The resulting oil was purified by column chromatography on silica gel (AcOEt/hexane, 3:1, v/v) to afford the amide 9c.
8-Iodo-9-(3,4-dimethoxy-benzyloxy)-3-[1.3]dioxolan-2,3,5a,10c-tetrahydro-1H,6H-5-oxaacephenanthrylene-10b-carbonitrile (11): Under an Ar atmosphere, to a solution of 3,4-dimethoxy-benzyl alcohol (272 mg, 1.62 mmol) in CH2Cl2 (8.1 mL) was added dropwise PBr3 (305 µL, 3.24 mmol) at 0 °C, and the mixture was stirred for 8 h. The reaction was quenched with H2O, and the aqueous phase was extracted with CHCl3. The combined organic layers were washed with brine, dried over MgSO4, filtered, and concentrated in vacuo. The resulting bromide was used without purification. Under an Ar atmosphere, to a solution of the phenol 108 (341 mg, 780 µmol) and the above bromide in DMF (3.9 mL) was added K2CO3 (216 mg, 1.62 µmol) at 0 °C, and the mixture was stirred for 8 h at rt. The reaction was quenched with H2O, and the aqueous phase was extracted with Et2O. The combined organic layers were washed with brine, dried over MgSO4, filtered, and concentrated in vacuo. The resulting oil was purified by column chromatography on silica gel (AcOEt/CH2Cl2, 1:100, v/v) to afford the benzyl ether 11 (416 mg, 708 µmol, 91%) as a colorless solid (mp 192–193 °C); 1H-NMR (400 MHz, CDCl3) δ: 1.66 (1H, ddd, J = 3.7, 13.7, 14.2 Hz), 1.77 (1H, ddd, J = 3.7, 3.7, 13.7 Hz), 2.52 (1H, ddd, J = 3.7, 14.2, 14.7 Hz), 2.74 (1H, td, J = 3.7, 14.7 Hz), 3.07 (1H, dd, J = 2.3, 15.8 Hz), 3.18 (1H, dd, J = 3.2, 15.8 Hz), 3.84–4.01 (11H, m), 5.02 (1H, d, J = 11.4 Hz), 5.10 (1H, d, J = 11.4 Hz), 5.26 (1H, ddd, J = 2.3, 3.2, 10.5 Hz), 6.01 (1H, d, J = 1.8 Hz), 6.77 (1H, s), 6.86 (1H, d, J = 8.0 Hz), 6.96 (1H, dd, J = 1.8, 8.0 Hz), 7.07 (1H, d, J = 1.8 Hz), 7.72 (1H, s); 13C-NMR (100 MHz, CDCl3) δ: 29.2, 29.7, 31.3, 32.6, 35.8, 50.9, 55.9, 55.9, 63.7, 65.3, 71.6, 79.6, 87.6, 104.4, 110.1, 110.5, 111.1, 119.5, 121.4, 128.6, 129.8, 132.3, 140.9, 143.3, 148.9, 149.2, 156.8; IR (KBr): 2240 cm–1; MS (EI): m/z 587 (M+); HRMS (EI): calcd for C27H26INO6: 587.0805, found: 587.0800.
N-[10b-Cyano-9-(3,4-dimethoxy-benzyloxy)-3-[1.3]dioxolan-2,3,5a,10c-tetrahydro-1H,6H-5-oxaace-phenanthrylene-8-yl]-N-methyl-acetamide (12): Under an Ar atmosphere, to a solution of the iodide 11 (32.3 mg, 55.0 µmol) in dioxane (275 µL) were added N-methylacetamide (8.40 µL, 110 µmol), CuI (10.5 mg, 55.0 µmol), (±)-trans-1,2-cyclohexanediamine (13.2 µL, 110 µmol), and K3PO4 (29.3 mg, 110 µmol) at rt, and the mixture was stirred for 4 h at 100 °C. Then to the reaction mixture were further added N-methylacetamide (8.40 µL, 110 µmol), CuI (10.5 mg, 55.0 µmol), (±)-trans-1,2-cyclohexanediamine (13.2 µL, 110 µmol), and K3PO4 (29.3 mg, 110 µmol), and the mixture was stirred for 15 h at 100 °C. The reaction mixture was then cooled to rt, diluted with saturated aqueous NH4Cl and AcOEt, and stirred for 40 min at rt. After filtration through Celite, the aqueous phase was extracted with AcOEt. The combined organic layers were dried over MgSO4, filtered, and concentrated in vacuo. The resulting oil was purified by column chromatography on silica gel (AcOEt/hexane, 3:1, v/v) to afford the amide 12 (5.3 mg, 9.95 µmol, 18%) as a colorless oil; 1H-NMR (400 MHz, CDCl3) δ: 1.62–1.93 (5H, m), 2.53–2.62 (1H, m), 2.78 (1H, dd, J = 2.7, 14.7 Hz), 3.09 (1H, d, J = 16.0 Hz), 3.17 (3H, s), 3.21 (1H, dd, J = 3.2, 16.0 Hz), 3.83–4.05 (11H, m), 4.99 (1H, d, J = 11.4 Hz), 5.05 (1H, d, J = 11.4 Hz), 5.30 (1H, ddd, J = 3.2, 5.5, 10.1 Hz), 6.03 (1H, d, J = 1.8 Hz), 6.84–6.88 (3H, m), 6.95 (1H, s), 7.10 (1H, s); 13C-NMR (100 MHz, CDCl3) δ: 21.9, 25.9, 29.6, 31.9, 32.9, 35.7, 36.1, 51.0, 53.8, 55.9, 63.8, 65.3, 70.7, 79.5, 104.4, 110.1, 110.2, 111.2, 111.8, 119.4, 125.1, 128.6, 130.6, 131.7, 133.6, 143.4, 149.3, 153.3, 171.1; IR (neat): 1660, 2223 cm–1; MS (EI): m/z 532 (M+); HRMS (EI): calcd for C30H32N2O7: 532.2210, found: 532.2210.
General Procedure for Cu-mediated Amination Reaction of Aryl Iodide (Table 2).
Under an Ar atmosphere, to a solution of the iodide 8c (100 µmol) in DMSO (0.3 M) were added NaN3 (2~10 eq), CuI (1 eq), and DMEDA (1.3 eq) at rt, and the mixture was stirred for 1 h at 100 °C. The reaction mixture was then cooled to rt, diluted with saturated aqueous NH4Cl and AcOEt, and stirred for 1 h at rt. The mixture was filtered through Celite, and the aqueous phase was extracted with AcOEt. The combined organic layers were washed with brine, dried over MgSO4, filtered, and concentrated in vacuo. The resulting oil was purified by column chromatography on silica gel (AcOEt/hexane, 1:1, v/v) to afford the amine 13c.
8-Amino-9-(3,4-dimethoxy-benzyloxy)-3-[1.3]dioxolan-2,3,5a,10c-tetrahydro-1H,6H-5-oxaacephenanthrylene-10b-carbonitrile (14): Under an Ar atmosphere, to a solution of the iodide 11 (25.7 mg, 43.8 µmol) in DMSO (146 µL) were added NaN3 (28.5 mg, 438 µmol), CuI (8.3 mg, 43.8 µmol), and DMEDA (6.12 µL, 56.9 µmol) at rt, and the mixture was stirred for 1 h at 100 °C. The reaction mixture was then cooled to rt, diluted with saturated aqueous NH4Cl and AcOEt, and stirred for 1 h at rt. The mixture was filtered through Celite, and the aqueous phase was extracted with AcOEt. The combined organic layers were washed with brine, dried over MgSO4, filtered, and concentrated in vacuo. The resulting oil was purified by column chromatography on silica gel (AcOEt/hexane, 1:1, v/v) to afford the amine 14 (13.7 mg, 28.8 µmol, 66%) as a colorless oil; 1H-NMR (400 MHz, CDCl3) δ: 1.75–1.84 (2H, m), 2.44–2.52 (1H, m), 2.74 (1H, ddd, J = 3.2, 3.2, 14.7 Hz), 2.98 (1H, dd, J = 2.3, 16.0 Hz), 3.15 (1H, dd, J = 3.7, 16.0 Hz), 3.84–4.01 (11H, m), 4.93 (1H, d, J = 10.7 Hz), 4.99 (1H, d, J = 10.7 Hz), 5.25 (1H, ddd, J = 2.3, 3.7, 10.5 Hz), 6.03 (1H, d, J = 1.8 Hz), 6.63 (1H, s), 6.75 (1H, s), 6.87 (1H, d, J = 8.2 Hz), 6.95–6.96 (2H, m); 13C-NMR (100 MHz, CDCl3) δ: 29.2, 29.8, 31.3, 33.1, 35.3, 51.0, 55.9, 55.9, 63.6, 65.3, 71.2, 79.9, 104.7, 110.4, 110.9, 111.1, 116.7, 119.6, 120.4, 122.5, 128.4, 129.3, 137.0, 143.2, 145.5, 149.0, 149.1; IR (neat): 2226, 3374 cm–1; MS (EI): m/z 476 (M+); HRMS (EI): calcd for C27H28N2O6: 476.1947, found:476.1970.
N-[10b-Cyano-9-hydroxy-3-[1.3]dioxolan-2,3,5a,10c-tetrahydro-1H,6H-5-oxaacephenanthrylene-8-yl]-N-methyl-acetamide (15): To a solution of benzyl ether 12 (7.9 mg, 14.8 µmol) in CH2Cl2/sat. NaHCO3 aq. (150 µL, 9:1, v/v) was added DDQ (13.4 mg, 59.2 µmol), and the mixture was stirred for 5 h at rt. The reaction mixture was diluted with saturated aqueous NaHCO3, and the aqueous phase was extracted with CH2Cl2. The combined organic layers were dried over MgSO4, filtered, and concentrated in vacuo. The resulting oil was purified by column chromatography on silica gel (MeOH/CH2Cl2, 1:50, v/v) to afford the phenol 15 (3.3 mg, 8.63 µmol, 58%) as a colorless oil; 1H-NMR (400 MHz, CDCl3) δ: 1.82–2.04 (5H, m), 2.56 (1H, ddd, J = 4.1, 14.7, 14.7 Hz), 2.84 (1H, d, J = 14.7 Hz), 3.07 (1H, d, J = 16.0 Hz), 3.20–3.25 (4H, m), 3.88–4.04 (5H, m), 5.30 (1H, ddd, J = 2.7, 5.5, 10.5 Hz), 6.06 (1H, d, J = 1.4 Hz), 7.01 (1H, s), 7.04 (1H, s); 13C-NMR (100 MHz, CDCl3) δ: 22.3, 29.7, 31.3, 32.8, 35.6, 36.2, 40.5, 50.9, 63.8, 65.4, 79.5, 104.6, 110.4, 115.9, 127.7, 129.4, 130.3, 132.7, 143.2, 151.6, 172.5; IR (neat): 1636, 2231, 3223 cm–1; MS (EI): m/z 382 (M+); HRMS (EI): calcd for C21H22N2O5: 382.1529, found: 382.1508.
ACKNOWLEDGEMENT
This study was supported by Health and Welfare Department of Toyama Prefectural Government (Toyama, Japan).
References
1. For halenaquinones, see (a) D. M. Roll, P. J. Scheuer, G. K. Matsumoto, and J. Clardy, J. Am. Chem. Soc., 1983, 105, 6177; CrossRef (b) M. Kobayashi, N. Shimizu, Y. Kyogoku, and I. Kitagawa, Chem. Pharm. Bull., 1985, 33, 1305; CrossRef (c) R. H. Lee, D. L. Slate, R. Moretti, K. A. Alvi, and P. Crews, Biochem. Biophys. Res. Commun., 1992, 184, 765; CrossRef (d) K. A. Alvi, M. C. Diaz, P. Crews, D. L. Slate, and R. Lee, J. Org. Chem., 1992, 57, 6604; CrossRef For halenaquinols, see (e) M. Kobayashi, N. Shimizu, I. Kitagawa, Y. Kyogoku, N. Harada, and H. Uda, Tetrahedron Lett., 1985, 26, 3833; CrossRef (f) N. Harada, H. Uda, M. Kobayashi, N. Shimizu, and I. Kitagawa, J. Am. Chem. Soc., 1989, 111, 5668; CrossRef (g) S. Ikegami, N. Kajiyama, Y. Ozaki, Y. Myotoishi, S. Miyashiro, S. Takayama, M. Kobayashi, and I. Kitagawa, FEBS Lett., 1992, 302, 284; CrossRef For xestquinones, see (h) H. Nakamura, J. Kobayashi, M. Kobayashi, Y. Ohizumi, and Y. Hirata, Chem. Lett., 1985, 713; CrossRef (i) M. Kobayashi, H. Nakamura, J. Kobayashi, and Y. Ohizumi, J. Pharmacol. Exp. Ther., 1991, 257, 82; (j) M. Kobayashi, A. Muroyama, H. Nakamura, J. Kobayashi, and Y. Ohizumi, J. Pharmacol. Exp. Ther., 1991, 257, 90; (k) H. Sakamoto, K. Furukawa, K. Matsunaga, H. Nakamura, and Y. Ohizumi, Biochemistry, 1995, 34, 12570. CrossRef
2. For total syntheses of related natural products, see (a) N. Harada, T. Sugioka, Y. Ando, H. Uda, and T. Kuriki, J. Am. Chem. Soc., 1988, 110, 8483; CrossRef (b) N. Harada, T. Sugioka, H. Uda, and T. Kuriki, J. Org. Chem., 1994, 59, 6606; CrossRef (c) A. Kojima, T. Takemoto, M. Sodeoka, and M. Shibasaki, J. Org. Chem., 1996, 61, 4876; CrossRef (d) S. P. Maddaford, N. G. Anderson, W. A. Cistofoli, and B. A. Keay, J. Am. Chem. Soc., 1996, 118, 10766; CrossRef (e) H. S. Sutherland, K. C. Higgs, N. Taylor, and R. Rodrigo, Tetrahedron, 2001, 57, 309. CrossRef
3. (a) N. Toyooka, M. Nagaoka, and H. Nemoto, Synlett, 2001, 1123; CrossRef (b) N. Toyooka, M. Nagaoka, E. Sasaki, H. Qin, H. Kakuda, and H. Nemoto, Tetrahedron, 2002, 58, 60973; CrossRef (c) Y. Matsuya, H. Qin, M. Nagaoka, and H. Nemoto, Heterocycles, 2004, 62, 207. CrossRef
4. Y. Matsuya, K. Sasaki, M. Nagaoka, H. Kakuda, N. Toyooka, N. Imanishi, H. Ochiai, and H. Nemoto, J. Org. Chem., 2004, 69, 7989. CrossRef
5. D.-Y. Yu, Y. Matsuya, Q.-L. Zhao, K. Ahmad, Z.-L. Wei, H. Nemoto, and T. Kondo, Apoptosis, 2007, 12, 1523. CrossRef
6. (a) Y. Matsuya, K. Sasaki, H. Ochiai, and H. Nemoto, Bioorg. Med. Chem., 2007, 15, 424; CrossRef (b) Y. Matsuya, N. Suzuki, S. Kobayashi, T. Miyahara, H. Ochiai, and H. Nemoto, Bioorg. Med. Chem., 2010, 18, 1477. CrossRef
7. K. Sugimoto, K. Tamura, N. Ohta, C. Tohda, N. Toyooka, H. Nemoto, and Y. Matsuya, Bioorg. Med. Chem. Lett., 2012, 22, 449. CrossRef
8. K. Sugimoto, K. Tamura, C. Tohda, N. Toyooka, H. Nemoto, and Y. Matsuya, Bioorg. Med. Chem., 2013, 21, 4459. CrossRef
9. Several palladium-catalyzed reactions were examined, however, no corresponding products were observed and starting materials were recovered.
10. A. Klapars, X. Huang, and S. L. Buchwald, J. Am. Chem. Soc., 2002, 124, 7421. CrossRef
11. J. T. Markiewicz, O. Wiest, and P. Helquist, J. Org. Chem., 2010, 75, 4887. CrossRef