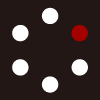
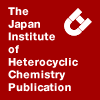
HETEROCYCLES
An International Journal for Reviews and Communications in Heterocyclic ChemistryWeb Edition ISSN: 1881-0942
Published online by The Japan Institute of Heterocyclic Chemistry
e-Journal
Full Text HTML
Received, 30th June, 2013, Accepted, 15th August, 2013, Published online, 26th August, 2013.
DOI: 10.3987/COM-13-S(S)69
■ Rotational Energy Barrier around the C1–C11 Single Bond in Lamellarins: A Study by Variable-Temperature NMR
Tsutomu Fukuda, Ryosuke Itoyama, Terufusa Minagawa, and Masatomo Iwao*
Division of Chemistry and Materials Science, Graduate School of Engineering, Nagasaki University, 1-14, Bunkyo-machi, Nagasaki 852-8521, Japan
Abstract
In order to estimate the free energy barrier to rotation around the C1–C11 single bond in lamellarins, new lamellarin analogues (1a), (1b), (2a), and (2b) possessing diastereotopic protons or carbons at the C1 aryl moiety were synthesized. Variable-temperature 1H and 13C NMR measurements of these analogues revealed that the free energy barriers to rotation around the C1–C11 axis in 5,6-saturated and 5,6-unsaturated lamellarins were around 72–74 and 83–87 kJ/mol, respectively.INTRODUCTION
The lamellarins constitute an important class of natural products of marine origin.1 Up to now, approximately fifty lamellarins have been characterized since the first isolation of lamellarins A–D from Lamellaria sp. in 1985.2–15 These lamellarins exhibit a number of interesting biological activities such as potent cytotoxicity against cancer cell lines,8,9,11,12,14–21 multi-drug resistance (MDR) reversal activity,15,16 anti-HIV activity,11,18,22 topoisomerase I inhibitory activity,23,24 inhibition of mitochondrial function,25–28 and protein kinases inhibitory activity.29 Lamellarins possess a unique 14-phenyl-6H-[1]benzopyrano- [4’,3’:4,5]pyrrolo[2,1-a]isoquinolin-6-one ring system (Figure 1). They are divided into two structural types different in the degree of unsaturation at 5,6-bond. The naturally occurring lamellarins are highly substituted by oxygen functionalities (OH and OMe) on this scaffold. The biological activities are dependent on the position and the number of OH and OMe groups.
According to X-ray crystallographic analyses of several lamellarins, the aryl group attached to C1 is
almost orthogonal to the pentacyclic ring.2,3,13,30 Therefore, if C1 aromatic ring is unsymmetrically substituted and rotation around the C1–C11 single bond is restricted, lamellarins are axially chiral. For the rotational energy barrier of lamellarins, Faulkner estimated it to be 600 kcal/mol for the highest maximum by molecular mechanics calculations (MM2).2 At this energy barrier, rotation around C1−C11 is essentially impossible. Interestingly, however, all naturally occurring lamellarins so far isolated were optically inactive, except for lamellarin S.7 The half-life of lamellarin S was estimated to be ca. 90 days by repeated measurements of its optical rotation over several months.7 These theoretical and experimental results suggested that lamellarins could be separated easily into their enantiomers by optical resolution. However, the resolution of naturally occurring or synthetic lamellarins has not been reported so far. Owing to our interest in the protein kinase inhibitory activity of lamellarin N and related compounds, we needed to prepare both enantiomers of lamellarin N. Thus, we synthesized several different types of O-protected lamellarins N and tried the optical resolution of them by chiral stationary phase HPLC. However, all attempts to produce optically active compounds were failed due to easy racemization of once-separated enantiomers in HPLC column at room temperature.31 Such discrepancy between our results and others prompted us to determine the actual rotational energy barriers of lamellarins by variable-temperature (VT) NMR experiments.32
RESULTS AND DISCUSSION
Charlton and coworkers investigated the rotational energy barriers of arylnaphthalene lignans such as justicidins A and B by VT NMR technique.33 Based upon Charlton’s studies, we designed lamellarin analogues (1) and (2) having 1,3-benzodioxol-5-yl group at the C1 position (Figure 2). These compounds may be suitable for VT NMR experiments, because two substituents R on the 1,3-benzodioxol-5-yl group are diastereotopic each other and, therefore, the chemical shifts of the substituents R in NMR spectra should be different, if rotation around C1–C11 single bond [interconversion between (aR)- and (aS)-isomers] is sufficiently slow on the NMR time scale.
The synthesis of lamellarin analogues (1) and (2) was effected by application of the method developed in our laboratories (Scheme 1).34 The known pentacyclic compound (3)34 was brominated regioselectively at C1 with N-bromosuccinimide (NBS) to give 4 in 89% yield. Subsequent Suzuki-Miyaura coupling of
4 with 1.5 equiv of arylboronic acids (5a) and (5b) under the standard conditions [Pd(PPh3)4 (10 mol%), Na2CO3, water, 1,2-dimethoxyethane (DME), reflux, 24 h] afforded the 5,6-saturated analogues (1a) and (1b) in 80% and 72% yields, respectively. Dehydrogenation of 1a and 1b using 2,3-dichloro-5,6-dicyano-1,4-benzoquinone (DDQ) in refluxing toluene produced the 5,6-unsaturated analogues (2a) and (2b) in 94% and 91% yields, respectively.
With the lamellarin analogues (1a), (1b), (2a), and (2b) in hand, we next carried out VT NMR experiments. Initially, 5,6-saturated lamellarin analogues (1a) and (1b) were analyzed. When 1H NMR of the sample (1a) was measured in chloroform-d at 22 °C, the diastereotopic methylene protons gave an AB-type spectrum at δ 6.017 and 6.030 ppm (Δν = 5.4 Hz and JAB = 1.1 Hz) showing the rotation around C1–C11 of 1a is sufficiently slow at this temperature (Figure 3). As the temperature increased, these peaks are broadened and coalesced at 50 °C (Tc = 323 K).
Next, 1H NMR spectra of 1b were measured at room temperature. Although variety of solvents (chloroform-d, acetone-d6, methanol-d4, DMSO-d6, benzene-d6 and toluene-d8) were tested, we couldn’t
obtain the spectra in which the signals corresponding to the diastereotopic methyl protons were separated into two singlets. On the other hand, the diastereotopic methyl carbon signals were observed as two singlets at δ 25.51 and 25.57 ppm (Δν = 6.0 Hz) in 13C NMR spectrum at 22 °C when toluene-d8 was used as a solvent (Figure 4). These peaks coalesced at 55 °C (Tc = 328 K).
The free energy barriers to rotation around C1–C11 single bond in 1a and 1b were calculated using these experimental data. The results were summarized in Table 1. By employing the Gutowsky-Holm equation [kc = 2–1/2πΔν for uncoupled signals and kc = 2–1/2π√(Δν + 6J2AB) for coupled signals] and the Eyring equation [ΔG‡ = 19.14Tc(10.32 + log Tc – log kc)],32, 35, 36 the free energy barriers (ΔG‡) at Tc for 1a and 1b were estimated to be 72.4 and 73.6 kJ/mol, respectively.37 The rates of enantiomerization (kenant) of 1a and 1b at 20 °C were roughly estimated to be 0.77 and 0.48 s–1, respectively, based on the approximation that ΔG‡ is invariant with temperature and the assumption that ΔG‡ is identical with the barrier to interconversion between (aR)- and (aS)-isomers.38 The half-lives of racemization for 1a and 1b were estimated to be 0.45 and 0.73 s, respectively, by using the equation (t1/2 = ln 2 / 2kenant).39 These data indicated that (aR)- and (aS)-isomers interconvert rapidly at 20 °C.40
Next, VT NMR experiments of 5,6-unsaturated lamellarin analogues (2a) and (2b) were carried out. The 1H NMR spectra of 2a were measured in toluene-d8 at the temperatures between 25 °C and 100 °C (Figure 5). When the sample (1a) was measured at 25 °C, the diastereotopic methylene protons gave an AB-type spectrum at δ 5.321 and 5.333 ppm (Δν = 4.8 Hz and JAB = 1.0 Hz). As the temperature increased, these peaks are broadened and coalesced at 95 °C (Tc = 368 K).
The 13C NMR spectra of 2b were measured in toluene-d8 at the temperatures between 22 °C and 120 °C (Figure 6). When the sample (2b) was measured at 22 °C, diastereotopic methyl carbon signals were observed at δ 25.53 and 25.61 ppm (Δν = 7.7 Hz). These peaks coalesced at 115 °C (Tc = 388 K).
From the VT NMR data for 2a and 2b, the free energy barriers (ΔG‡) at Tc, the rates of enantiomerization (kenant) at 20 °C, and the half-lives of racemization (t1/2) at 20 °C were estimated in a similar manner as described for 1a and 1b. The results were summarized in Table 2. The free energy barriers (ΔG‡) of 2a and 2b were found to be approximately 10 kJ/mol higher than those of 1a and 1b.37 These differences may be accounted for by the difference of flexibility of 5,6-saturated or 5,6-unsaturated lamellarin framework. The rotations around C1–C11 single bond of 5,6-unsaturated lamellarins may be somewhat more restricted than those of 5,6-saturated ones due to the rigidity of the unsaturated pentacyclic system.
The short half-life data (t1/2) indicated the separation of each enantiomer is still difficult in the 5,6-unsaturated lamellarins at room temperature.40
In conclusion, we have established the free energy barriers for rotation around the C1–C11 single bond of 5,6-saturated and 5,6-unsaturated lamellarins by VT NMR experiments. The energy barriers are found to be insufficiently high to allow the optical resolution of lamellarins. These data are in good agreement with the facts that almost all naturally occurring lamellarins are optically inactive. The reported optical activity of lamellarin S is not clearly understood at the present stage. The data obtained in this research indicate that lamellarins can be regarded as single compounds rather than racemic mixtures. This finding is especially important in assessment of the biological activities of lamellarins for drug discovery.40,41
EXPERIMENTAL
Melting points were determined with a Yanagimoto micro melting points apparatus and are uncorrected. IR spectra were obtained with a Thermo Nicolet Nexus 670 NT FT-IR instrument and are reported in terms of frequency of absorption (cm–1). NMR spectra were recorded on a JEOL JNM-AL400 instrument (400 MHz for 1H and 100 MHz for 13C) using tetramethylsilane as an internal standard (δ 0.0). Data for 1H NMR spectra are reported as follows: chemical shift (δ ppm), multiplicity, coupling constant (Hz), and integration. Data for 13C NMR spectra are reported in terms of chemical shift. High resolution mass spectra were recorded on a JEOL JMS-700N spectrometer. Elemental analysis was performed for C, H, and N using a Perkin Elmer 2400II instrument. Column chromatography was conducted on Silica Gel 60N, 63–210 m (Kanto Chemical Co., Inc.) or Chromatorex NH-DM1020 silica gel (Fuji Silysia Chemical Ltd.). Flash chromatography was conducted on Silica Gel 60N, 40–50 m (Kanto Chemical Co., Inc.). t-Butyllithium was used after titration with 2,5-dimethoxybenzyl alcohol. Solvents were dried and distilled by standard methods if necessary.
14-Bromo-3,11-diisopropoxy-2,12-dimethoxy-8,9-dihydro-6H-[1]benzopyrano[4’,3’:4,5]pyrrolo[2,1-a]isoquinolin-6-one (4). A solution of NBS (151 mg, 0.848 mmol) in DMF (13 mL) was added dropwise to a solution of 334 (382 mg, 0.824 mmol) in DMF (10 mL) at 0 °C. The mixture was stirred for 24 h at 0 °C. The solution was diluted with water and the product was extracted with CH2Cl2. The extract was washed with water and brine, dried over Na2SO4, and evaporated. The residue was purified by column chromatography over Silica Gel 60N (CH2Cl2–EtOAc = 20:1) to give 4 as pale yellow solid (398 mg, 89%). Recrystallization from CH2Cl2-hexane gave colorless powder. Mp 191.5–192.5 °C; IR (KBr): 1707, 1420, 1210, 1163, 1040 cm–1; 1H NMR (400 MHz, CDCl3): δ 1.43 (d, J = 6.1 Hz, 12H), 3.04 (t, J = 6.5 Hz, 2H), 3.95 (s, 3H), 3.96 (s, 3H), 4.58 (sep, J = 6.1 Hz, 1H), 4.63 (sep, J = 6.1 Hz, 1H), 4.71–4.78 (m, 2H), 6.83 (s, 1H), 6.91 (s, 1H), 8.14 (s, 1H), 8.20 (s, 1H); 13C NMR (100 MHz, CDCl3): δ 21.8, 22.1, 28.9, 42.6, 56.3, 56.3, 71.4, 86.6, 103.3, 104.8, 109.5, 109.7, 114.1, 114.7, 119.2, 127.1, 127.3, 135.3, 146.0, 146.6, 147.7, 148.1, 148.8, 154.8. Anal. Calcd for C27H28BrNO6: C, 59.79; H, 5.20; N, 2.58. Found: C, 59.98; H, 5.09; N, 2.37.
2,2-Dimethyl-1,3-benzodioxol-5-ylboronic acid (5b). A solution of NBS (3.92 g, 22.0 mmol) in DMF (20 mL) was added dropwise to a solution of 2,2-dimethyl-1,3-benzodioxole (3.00 g, 20.0 mmol) in DMF (20 mL) at 0 °C. The mixture was stirred for 24 h at 0 °C. The solution was diluted with water and the product was extracted with Et2O. The extract was washed with water and brine, dried over Na2SO4, and evaporated. The residue was purified by bulb-to-bulb distillation (80 °C / 0.15 mmHg) to give 5-bromo-2,2-dimethyl-1,3-benzodioxole (5b') as colorless oil (3.71 g, 81%). IR (KBr): 1484, 1378, 1250, 979, 839, 797 cm–1; 1H NMR (400 MHz, CDCl3): δ 1.67 (s, 6H), 6.59 (d, J = 8.0 Hz, 1H), 6.86 (d, J = 2.0 Hz, 1H), 6.89 (dd, J = 2.0 and 8.0 Hz, 1H); 13C NMR (100 MHz, CDCl3): δ 25.8, 109.4, 112.1, 112.3, 119.0, 123.6, 146.8, 148.4; HREIMS m/z. Calcd for C9H9BrO2 (M+): 227.9786. Found: 227.9765.
Under an argon atmosphere, a pentane solution of t-butyllithium (1.50 M, 14.0 mL, 21.0 mmol) was added dropwise to a solution of 5-bromo-2,2-dimethyl-1,3-benzodioxole (5b') (2.30 g, 10.0 mmol) in THF (36 mL) at –78 °C. After being stirred for 1 h, trimethyl borate (1.67 mL, 15.0 mmol) was added as a neat liquid and the mixture was stirred for 1 h at –78 °C. The reaction mixture was allowed to warm to room temperature and stirred for an additional 1 h. The mixture was quenched with saturated aqueous NH4Cl and concentrated. The products were adjusted to pH 3 with acetic acid and the mixture was extracted with CH2Cl2. The extract was washed successively with water, saturated aqueous NaHCO3, and brine, dried over Na2SO4, and evaporated. The residue was washed with hexane and dried under reduced pressure to give 5b as colorless powder (1.29 g, 66%). This compound was used for the next reaction without further purification. IR (KBr): 3227, 1441, 1376, 1255, 980, 841 cm–1; 1H NMR (400 MHz, CDCl3): δ 1.72 (s, 6H), 6.86 (d, J = 7.6 Hz, 1H), 7.52 (d, J = 1.6 Hz, 1H), 7.74 (dd, J = 1.6 and 7.6 Hz, 1H); 13C NMR (100 MHz, CDCl3): δ 25.9, 108.3, 114.3, 118.0, 130.6, 147.3, 151.2.
14-(1,3-Benzodioxol-5-yl)-3,11-diisopropoxy-2,12-dimethoxy-8,9-dihydro-6H-[1]benzopyrano[4’,3’:4,5]pyrrolo[2,1-a]isoquinolin-6-one (1a). Under an argon atmosphere, a mixture of 5a (68.8 mg, 0.415 mmol), 4 (150 mg, 0.277 mmol), Pd(PPh3)4 (32.0 mg, 27.7 µmol), Na2CO3 (193 mg, 1.82 mmol), DME (9 mL), and degassed water (533 µL) was refluxed for 24 h. After cooling to room temperature, the solvent was evaporated in vacuo and the residue was extracted with CH2Cl2. The combined extracts were washed successively with water and brine, dried over Na2SO4, and concentrated in vacuo. The crude product was purified by column chromatography over Silica Gel 60N (toluene–EtOAc = 10:1) to give 1a as colorless solid (130 mg, 80%). Recrystallization from CH2Cl2–hexane gave colorless powder. Mp > 300 °C; IR (KBr): 1710, 1480, 1414, 1268, 1210, 1111, 1040 cm–1; 1H NMR (400 MHz, CDCl3): δ 1.38 (d, J = 6.1 Hz, 6H), 1.39 (d, J = 6.1 Hz, 6H), 3.02–3.16 (m, 2H), 3.42 (s, 3H), 3.51 (s, 6H), 4.54 (sep, J = 6.1 Hz, 1H), 4.56 (sep, J = 6.1 Hz, 1H), 4.65–4.74 (m, 1H), 4.83–4.91 (m, 1H), 6.02 (d, J = 1.1 Hz, 1H), 6.03 (d, J = 1.1 Hz, 1H), 6.72 (s, 1H), 6.73 (s, 1H), 6.77 (s, 1H), 6.92 (s, 1H), 6.99 (d, J = 1.5 Hz, 1H), 7.02 (d, J = 7.9 Hz, 1H), 7.04 (dd, J = 1.5 and 7.9 Hz, 1H); 13C NMR (100 MHz, CDCl3): δ 21.8, 22.1, 28.7, 42.4, 55.2, 55.6, 71.3, 71.5, 101.3, 103.5, 105.0, 109.1, 109.3, 110.3, 111.7, 113.7, 114.5, 114.6, 120.0, 124.7, 126.6, 128.2, 129.2, 136.1, 146.0, 146.5, 147.1, 147.3, 147.4, 148.3, 148.6, 155.6. Anal. Calcd for C34H33NO8: C, 69.97; H, 5.70; N, 2.40. Found: C, 70.15; H, 5.50; N, 2.25.
14-(2,2-Dimethyl-1,3-benzodioxol-5-yl)-3,11-diisopropoxy-2,12-dimethoxy-8,9-dihydro-6H-[1]benzopyrano[4’,3’:4,5]pyrrolo[2,1-a]isoquinolin-6-one (1b). According to the procedure described for the preparation of 1a, 5b (42.9 mg, 0.221 mmol), 4 (80.0 mg, 0.147 mmol), and Pd(PPh3)4 (17.0 mg, 14.7 µmol) were reacted. After successive purification by column chromatography over Silica Gel 60N (toluene–EtOAc = 10:1) and flash chromatography over Silica Gel 60N (hexane–EtOAc = 2:1), 1b was obtained as colorless solid (65.4 mg, 72%). Recrystallization from CH2Cl2–hexane gave colorless powder. Mp 210–211 °C; IR (KBr): 1701, 1481, 1417, 1239, 1212, 1166, 1116 cm-1; 1H NMR (400 MHz, CDCl3): δ 1.38 (d, J = 6.1 Hz, 6H), 1.39 (d, J = 6.1 Hz, 6H), 1.70 (s, 3H), 1.70 (s, 3H), 3.09 (m, 2H), 3.43 (s, 3H), 3.51 (s, 3H), 4.54 (sep, J = 6.1 Hz, 1H), 4.56 (sep, J = 6.1 Hz, 1H), 4.68–4.77 (m, 1H), 4.79–4.88 (m, 1H), 6.75 (s, 1H), 6.77 (s, 1H), 6.78 (s, 1H), 6.88 (d, J = 1.5 Hz, 1H), 6.91 (d, J = 7.8 Hz, 1H), 6.91 (s, 1H), 6.97 (dd, J = 1.5 and 7.8 Hz, 1H); 1H NMR (400 MHz, toluene-d8): δ 1.14 (d, J = 6.1 Hz, 3H), 1.14 (d, J = 6.1 Hz, 3H), 1.19 (d, J = 6.1 Hz, 6H), 1.37 (s, 3H), 1.38 (s, 3H), 2.60 (t, J = 6.9 Hz, 2H), 3.28 (s, 3H), 3.32 (s, 3H), 4.18 (sep, J = 6.1 Hz, 1H), 4.28 (sep, J = 6.1 Hz, 1H), 4.54–4.62 (m, 1H), 4.62–4.71 (m, 1H), 6.51 (s, 1H), 6.67 (d, J = 7.8 Hz, 1H), 6.84 (s, 1H), 6.85 (dd, J = 1.6 and 7.8 Hz, 1H), 6.86 (s, 1H), 6.89 (d, J = 1.6 Hz, 1H), 6.91 (s, 1H); 13C NMR (100 MHz, CDCl3): δ 21.8, 21.9, 22.1, 25.8, 25.8, 28.6, 42.4, 55.0, 55.3, 71.3, 71.5, 103.6, 105.0, 108.8, 109.3, 110.4, 111.3, 113.7, 114.6, 114.8, 118.3, 120.2, 124.0, 126.5, 128.3, 128.4, 136.1, 146.0, 146.5, 147.0, 147.1, 147.3, 148.2, 148.6, 155.7; 13C NMR (100 MHz, toluene-d8): δ 22.0, 22.2, 25.5, 25.6, 28.6, 42.6, 54.7, 54.9, 71.1, 71.3, 104.3, 105.9, 108.9, 110.1, 111.0, 111.9, 114.7, 115.1, 116.1, 118.2, 121.1, 124.5, 126.7, 129.2, 129.5, 135.7, 146.9, 147.3, 147.5, 147.8, 147.9, 148.7, 149.8, 155.2. Anal. Calcd for C36H37NO8: C, 70.69; H, 6.10; N, 2.29. Found: C, 70.41; H, 6.12; N, 2.24.
14-(1,3-Benzodioxol-5-yl)-3,11-diisopropoxy-2,12-dimethoxy-6H-[1]benzopyrano[4’,3’:4,5]pyrrolo[2,1-a]isoquinolin-6-one (2a). Under an argon atmosphere, a solution of 1a (60.0 mg, 0.103 mmol) and DDQ (35.0 mg, 0.154 mmol) in toluene (6.0 mL) was refluxed for 17 h. After cooling to room temperature, the solvent was evaporated in vacuo. The residue was purified by column chromatography over Chromatorex NH-DM1020 silica gel (hexane–EtOAc = 2:1) to give 2a as colorless solid (56.3 mg, 94%). Recrystallization from CH2Cl2–hexane gave colorless powder. Mp 245.5–246 °C; IR (KBr): 1710, 1430, 1267, 1225, 1178, 1035 cm–1; 1H NMR (400 MHz, CDCl3): δ 1.41 (d, J = 6.1 Hz, 6H), 1.43 (d, J = 6.1 Hz, 6H), 3.52 (s, 3H), 3.53 (s, 3H), 4.57 (sep, J = 6.1 Hz, 1H), 4.70 (sep, J = 6.1 Hz, 1H), 6.06 (s, 2H), 6.78 (s, 1H), 6.96 (s, 1H), 7.01 (d, J = 7.4 Hz, 1H), 7.08 (d, J = 1.5 Hz, 1H), 7.09 (d, J = 7.9 Hz, 1H), 7.09 (s, 1H), 7.12 (dd, J = 1.5 and 7.9 Hz, 1H), 7.18 (s, 1H), 9.21 (d, J = 7.4 Hz, 1H); 1H NMR (400 MHz, toluene-d8): δ 1.14 (d, J = 6.1 Hz, 6H), 1.20 (d, J = 6.1 Hz, 6H), 3.24 (s, 3H), 3.28 (s, 3H), 4.17 (sep, J = 6.1 Hz, 1H), 4.32 (sep, J = 6.1 Hz, 1H), 5.32 (d, J = 1.0 Hz, 1H), 5.33 (d, J = 1.0 Hz, 1H), 6.62 (d, J = 7.3 Hz, 1H), 6.71 (d, J = 7.8 Hz, 1H), 6.82 (s, 1H), 6.84 (s, 1H), 6.85 (dd, J = 1.7 and 7.8 Hz, 1H), 6.88 (s, 1H), 6.88 (d, J = 1.7 Hz, 1H), 7.18 (s, 1H), 9.49 (d, J = 7.3 Hz, 1H); 13C NMR (100 MHz, CDCl3): δ 21.8, 21.9, 55.2, 55.6, 71.2, 71.5, 101.4, 103.5, 105.6, 105.7, 107.9, 109.2, 109.8, 110.5, 110.6, 112.2, 112.4, 118.9, 123.2, 124.8, 125.3, 129.4, 129.5, 134.4, 146.5, 146.6, 147.5, 147.9, 148.4, 148.5, 150.2, 155.5. Anal. Calcd for C34H31NO8: C, 70.21; H, 5.37; N, 2.41. Found: C, 69.98; H, 5.11; N, 2.23.
14-(2,2-Dimethyl-1,3-benzodioxol-5-yl)-3,11-diisopropoxy-2,12-dimethoxy-6H-[1]benzopyrano[4’,3’:4,5]pyrrolo[2,1-a]isoquinolin-6-one (2b). According to the procedure described for the preparation of 2a, 1b (111 mg, 0.182 mmol) and DDQ (62.0 mg, 0.273 mmol) were reacted. After chromatographic purification over Chromatorex NH-DM1020 silica gel (hexane–EtOAc = 2:1), 2b was obtained as colorless solid (101 mg, 91%). Recrystallization from CH2Cl2-hexane gave colorless powder. Mp 208.5–210 °C; IR (KBr): 1702, 1483, 1427, 1265, 1224, 1176, 1111 cm–1; 1H NMR (400 MHz, CDCl3): δ 1.41 (d, J = 6.1 Hz, 6H), 1.43 (d, J = 6.1 Hz, 6H), 1.72 (s, 6H), 3.52 (s, 3H), 3.53 (s, 3H), 4.57 (sep, J = 6.1 Hz, 1H), 4.70 (sep, J = 6.1 Hz, 1H), 6.81 (s, 1H), 6.96 (s, 1H), 6.97 (d, J = 1.6 Hz, 1H), 6.97 (d, J = 7.8 Hz, 1H), 7.00 (d, J = 7.4 Hz, 1H), 7.05 (dd, J = 1.6 and 7.8 Hz, 1H), 7.09 (s, 1H), 7.22 (s, 1H), 9.19 (d, J = 7.4 Hz, 1H); 1H NMR (400 MHz, toluene-d8): δ 1.16 (d, J = 6.1 Hz, 3H), 1.17 (d, J = 6.1 Hz, 3H), 1.22 (d, J = 6.1 Hz, 3H), 1.23 (d, J = 6.1 Hz, 3H), 1.39 (s, 3H), 1.39 (s, 3H), 3.29 (s, 3H), 3.33 (s, 3H), 4.20 (sep, J = 6.1 Hz, 1H), 4.36 (sep, J = 6.1 Hz, 1H), 6.64 (d, J = 7.3 Hz, 1H), 6.69 (d, J = 7.8 Hz, 1H), 6.84 (dd, J = 1.7 and 7.8 Hz, 1H), 6.84 (s, 1H), 6.86 (s, 1H), 6.86 (s, 1H), 6.87 (d, J = 1.7 Hz, 1H), 7.23 (s, 1H), 9.39 (d, J = 7.3 Hz, 1H); 13C NMR (100 MHz, CDCl3): δ 21.8, 21.9, 21.9, 21.9, 25.8, 25.9, 54.9, 55.3, 71.2, 71.5, 103.5, 105.6, 105.7, 107.8, 108.9, 109.9, 110.5, 111.0, 111.7, 112.3, 118.5, 119.0, 123.2, 124.5, 124.7, 128.6, 129.5, 134.5, 146.5, 146.6, 147.4, 147.8, 148.3, 148.4, 150.1, 155.6; 13C NMR (100 MHz, toluene-d8): δ 22.0, 22.0, 22.0, 22.0, 25.5, 25.6, 54.4, 54.9, 70.9, 71.0, 104.1, 106.1, 106.4, 108.8, 108.9, 110.5, 111.0, 111.3, 112.3, 112.4, 118.3, 119.6, 123.3, 125.0, 125.0, 129.4, 129.6, 134.1, 147.2, 147.5, 147.7, 148.6, 148.7, 148.9, 151.0, 155.2. Anal. Calcd for C36H35NO8: C, 70.92; H, 5.79; N, 2.30. Found: C, 70.65; H, 5.73; N, 2.14.
VT NMR experiments. VT NMR spectra were obtained on JEOL JNM-AL400 instrument (400 MHz for 1H and 100 MHz for 13C). The temperature was measured at the probe. Samples were allowed to equilibrate for 10 minutes at each temperature before recording the spectrum.
ACKNOWLEDGEMENTS
This work was financially supported by a Grant–in–Aid for Scientific Research (B) (No. 20310135) from the Japan Society for the Promotion of Science (JSPS).
References
1. For recent reviews, see: (a) C. Bailly, Curr. Med. Chem. - Anti-Cancer Agents, 2004, 4, 363; CrossRef (b) P. Cironi, F. Albericio, and M. Álvarez, Prog. Heterocycl. Chem., 2005, 16, 1; CrossRef (c) S. T. Handy and Y. Zhang, Org. Prep. Proc. Int., 2005, 37, 411; CrossRef (d) H. Fan, J. Peng, M. T. Hamann, and J.-F. Hu, Chem. Rev., 2008, 108, 264; CrossRef ; CrossRef (f) J. Kluza, P. Marchetti, and C. Bailly, 'Modern Alkaloids: Structure, Isolation, Synthesis and Biology,' ed. by E. Fattorusso and O. Taglialatela-Scafati, Wiley-VCH, Weinheim, 2008, pp. 171-187; (g) D. Pla, F. Albericio, and M. Álvarez, Med. Chem. Commun., 2011, 2, 689; CrossRef (h) A.-L. Fan, W.-H. Lin, and Y.-X. Jia, J. Chin. Pharm. Sci., 2011, 20, 425; (i) T. Fukuda, F. Ishibashi, and M. Iwao, Heterocycles, 2011, 83, 491. CrossRef
2. R. J. Andersen, D. J. Faulkner, H. Cun-heng, G. D. Van Duyne, and J. Clardy, J. Am. Chem. Soc., 1985, 107, 5492. CrossRef
3. N. Lindquist and W. Fenical, J. Org. Chem., 1988, 53, 4570. CrossRef
4. A. R. Carroll, B. F. Bowden, and J. C. Coll, Aust. J. Chem., 1993, 46, 489. CrossRef
5. S. Urban, M. S. Butler, and R. J. Capon, Aust. J. Chem., 1994, 47, 1919. CrossRef
6. S. Urban, L. Hobbs, J. N. A. Hooper, and R. J. Capon, Aust. J. Chem., 1995, 48, 1491. CrossRef
7. S. Urban and R. J. Capon, Aust. J. Chem., 1996, 49, 711.
8. M. V. R. Reddy, D. J. Faulkner, Y. Venkateswarlu, and M. R. Rao, Tetrahedron, 1997, 53, 3457. CrossRef
9. C. L. Cantrell, A. Groweiss, K. R. Gustafson, and M. R. Boyd, Nat. Prod. Lett., 1999, 14, 39. CrossRef
10. R. A. Davis, A. R. Carroll, G. K. Pierens, and R. J. Quinn, J. Nat. Prod., 1999, 62, 419. CrossRef
11. M. V. R. Reddy, M. R. Rao, D. Rhodes, M. S. T. Hansen, K. Rubins, F. D. Bushman, Y. Venkateswarlu, and D. J. Faulkner, J. Med. Chem., 1999, 42, 1901. CrossRef
12. J. Ham and H. Kang, Bull. Korean Chem. Soc., 2002, 23, 163. CrossRef
13. P. Krishnaiah, V. L. N. Reddy, G. Venkataramana, K. Ravinder, M. Srinivasulu, T. V. Raju, K. Ravikumar, D. Chandrasekar, S. Ramakrishna, and Y. Venkateswarlu, J. Nat. Prod., 2004, 67, 1168. CrossRef
14. S. M. Reddy, M. Srinivasulu, N. Satyanarayana, A. K. Kondapi, and Y. Venkateswarlu, Tetrahedron, 2005, 61, 9242. CrossRef
15. F. Plisson, X.-C. Huang, H. Zhang, Z. Khalil, and R. J. Capon, Chem. Asian J., 2012, 7, 1616. CrossRef
16. A. R. Quesada, M. D. G. Grávalos, and J. L. F. Puentes, Br. J. Cancer, 1996, 74, 677. CrossRef
17. F. Ishibashi, S. Tanabe, T. Oda, and M. Iwao, J. Nat. Prod., 2002, 65, 500. CrossRef
18. C. P. Ridley, M. V. R. Reddy, G. Rocha, F. D. Bushman, and D. J. Faulkner, Bioorg. Med. Chem., 2002, 10, 3285. CrossRef
19. C. Tardy, M. Facompré, W. Laine, B. Baldeyrou, D. García-Gravalos, A. Francesch, C. Mateo, A. Pastor, J. A. Jiménez, I. Manzanares, C. Cuevas, and C. Bailly, Bioorg. Med. Chem., 2004, 12, 1697. CrossRef
20. D. Pla, A. Marchal, C. A. Olsen, A. Francesch, C. Cuevas, F. Albericio, and M. Álvarez, J. Med. Chem., 2006, 49, 3257. CrossRef
21. M. Chittchang, P. Batsomboon, S. Ruchirawat, and P. Ploypradith, ChemMedChem, 2009, 4, 457. CrossRef
22. H. Kamiyama, Y. Kubo, H. Sato, N. Yamamoto, T. Fukuda, F. Ishibashi, and M. Iwao, Bioorg. Med. Chem., 2011, 19, 7541. CrossRef
23. M. Facompré, C. Tardy, C. Bal-Mahieu, P. Colson, C. Perez, I. Manzanares, C. Cuevas, and C. Bailly, Cancer Res., 2003, 63, 7392.
24. E. Marco, W. Laine, C. Tardy, A. Lansiaux, M. Iwao, F. Ishibashi, C. Bailly, and F. Gago, J. Med. Chem., 2005, 48, 3796. CrossRef
25. J. Kluza, M.-A. Gallego, A. Loyens, J.-C. Beauvillain, J.-M. F. Sousa-Faro, C. Cuevas, P. Marchetti, and C. Bailly, Cancer Res., 2006, 66, 3177. CrossRef
26. M.-A. Gallego, C. Ballot, J. Kluza, N. Hajji, A. Martoriati, L. Castéra, C. Cuevas, P. Formstecher, B. Joseph, G. Kroemer, C. Bailly, and P. Marchetti, Oncogene, 2008, 27, 1981. CrossRef
27. C. Ballot, J. Kluza, A. Martoriati, U. Nyman, P. Formstecher, B. Joseph, C. Bailly, and P. Marchetti, Mol. Cancer Ther., 2009, 8, 3307. CrossRef
28. C. Ballot, J. Kluza, S. Lancel, A. Martoriati, S. M. Hassoun, L. Mortier, J.-C. Vienne, G. Briand, P. Formstecher, C. Bailly, R. Nevière, and P. Marchetti, Apoptosis, 2010, 15, 769. CrossRef
29. D. Baunbæk, N. Trinkler, Y. Ferandin, O. Lozach, P. Ploypradith, S. Rucirawat, F. Ishibashi, M. Iwao, and L. Meijer, Mar. Drugs, 2008, 6, 514. CrossRef
30. (a) M. Banwell, B. Flynn, and D. Hockless, Chem. Commun., 1997, 2259; CrossRef (b) M. G. Banwell, B. L. Flynn, D. C. R. Hockless, R. W. Longmore, and A. D. Rae, Aust. J. Chem., 1999, 52, 755.
31. K. Yoshida, R. Itoyama, M. Yamahira, J. Tanaka, N. Loaëc, O. Lozach, E. Durieu, T. Fukuda, F. Ishibashi, L. Meijer, and M. Iwao, J. Med. Chem., in press.
32. (a) C. Wolf, 'Dynamic Stereochemistry of Chiral Compounds,' The Royal Society of Chemistry, Cambridge, 2008, pp. 136-179; (b) D. Casarini, L. Lunazzi, and A. Mazzanti, Eur. J. Org. Chem., 2010, 2035; CrossRef (c) H. Friebolin, 'Basic One- and Two-Dimensional NMR Spectroscopy,' WILEY-VCH Verlag GmbH & Co. KGaA, Weinheim, 2011, pp. 313-342.
33. J. L. Charlton, C. J. Oleschuk, and G.-L. Chee, J. Org. Chem., 1996, 61, 3452. CrossRef
34. T. Ohta, T. Fukuda, F. Ishibashi, and M. Iwao, J. Org. Chem., 2009, 74, 8143. CrossRef
35. P. J. Garratt, S. N. Thorn, and R. Wrigglesworth, Tetrahedron, 1994, 50, 12211. CrossRef
36. A. R. Modarresi-Alam, H. Keykha, F. Khamooshi and H. A. Dabbagh, Tetrahedron, 2004, 60, 1525. CrossRef
37. The free energy barriers to rotation around the C1–C11 axis in 5,6-saturated and 5,6-unsaturated lamellarins (1a) and (2a) were calculated to be around 69–72 and 82–90 kJ/mol, respectively, by DFT at the B3LYP level with the 6-31G(d) basis set. These results are in good agreement with the experimental results. See supporting information for more details.
38. A. Ahmed, R. A. Bragg, J. Clayden, L. W. Lai, C. McCarthy, J. H. Pink, N. Westlund, and S. A. Yasin, Tetrahedron, 1998, 54, 13277. CrossRef
39. C. Wolf, 'Dynamic Stereochemistry of Chiral Compounds,' The Royal Society of Chemistry, Cambridge, 2008, pp. 29-135.
40. (a) S. R. LaPlante, P. J. Edwards, L. D. Fader, A. Jakalian, and O. Hucke, ChemMedChem, 2011, 6, 505; CrossRef (b) S. R. LaPlante, L. D. Fader, K. R. Fandrick, D. R. Fandrick, O. Hucke, R. Kemper, S. P. F. Miller, and P. J. Edwards, J. Med. Chem., 2011, 54, 7005. CrossRef
41. (a) J. Clayden, W. J. Moran, P. J. Edwards, and S. R. LaPlante, Angew. Chem. Int. Ed., 2009, 48, 6398; CrossRef (b) A. Zask, J. Murphy, and G. A. Ellestad, Chirality, 2013, 25, 265. CrossRef