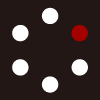
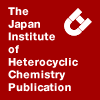
HETEROCYCLES
An International Journal for Reviews and Communications in Heterocyclic ChemistryWeb Edition ISSN: 1881-0942
Published online by The Japan Institute of Heterocyclic Chemistry
e-Journal
Full Text HTML
Received, 1st May, 2013, Accepted, 20th May, 2013, Published online, 28th May, 2013.
DOI: 10.3987/COM-13-S(S)11
■ Efficient Oxidative Spirolactamization by μ-Oxo Bridged Heterocyclic Hypervalent Iodine Compound
Toshifumi Dohi, Eisuke Mochizuki, Daisuke Yamashita, Keitaro Miyazaki, and Yasuyuki Kita*
College of Pharmaceutical Sciences, Ritsumeikan University, 1-1-1 Nojihigashi, Kusatsu, Shiga, 525-8577, Japan
Abstract
In this report, the authors have clarified that the μ-oxo bridged heterocyclic hypervalent iodine compound 1 shows a more effective reactivity in the oxidative dearomatizing spirolactamization compared to conventional PIDA (phenyliodine(III) diacetate). Besides the high reactivity, a new selectivity during the oxidations based on the steric discrimination of the nitrogen groups of the pre-spirocyclic substrates is demonstrated for the heterocyclic hypervalent iodine compound 1.INTRODUCTION
Lactams are ubiquitous in nature and also familiar as artificial molecules in industry and pharmaceutical utilizations for our benefits. In organic chemistry, some lactam structures would endow unique characteristics to organic molecules in discovering unexpected reactivities and physical properties for new applications. Detachable lactam functionalities were frequently installed as a directing group and chiral auxiliary in many reactions. One such example is the radical translocations for controlling the reaction course and selectivity.1 Cyclic lactam parts were sometimes introduced into ring systems, for example, Tröger’s base,2a,b for tailoring the molecular twist for the purpose of developing unusual properties.2 Consequently, the new design of lactam compounds has being elaborated even in recent years due to such abundant functional attributes expected from the structures.
Among the synthetic targets, a particular interest has recently been paid to the spirocyclic class of lactam compounds together with significant synthetic advances for accessing these structures. The presence of spirolactams in naturally-occurring systems and their unique three-dimensional structure including two planes make these compounds fascinating structural motifs for organic chemists. Thus, spirolactams are important targets in modern organic synthesis, and a variety of new and efficient systems have recently been reported in the literature.3
Our research group has also been interested for a long time in spirocyclizations induced by hypervalent iodine reagents for constructing quaternary spiro centers based on the oxidative dearomatization strategies of phenols and their derivatives.4-7 Using phenyliodine(III) diacetate [PIDA, PhI(OAc)2] (Figure 1) and phenyliodine(III) bis(trifluoroacetate) [PIFA, PhI(OCOCF3)2], we demonstrated that the oxidations of phenols carrying a nucleophilic part at the appropriate position of the side chain would cause dearomatizations accompanied by spirocyclizations leading to spirodienone compounds including lactones and lactams under the suitable conditions of solvent and activation.4 The high efficiency of the spirocyclization processes typically allowed the catalytic use of hypervalent iodine reagents in combination with a suitable stoichiometric oxidant.5 Recyclable alternatives to PIDA and PIFA have also been developed for the spirocyclizations by fine reagent designs.6 During these studies, we have recently encountered the high reactivity of an oxygen-bridged hypervalent iodine dimer, i.e., [PhI(OCOCF3)]2O, for the spirocyclizations and other phenolic oxidations.7
Based on the background and original knowledge, combined interest in defining a catalytic alternative of the reactive hypervalent iodine dimer further occurred for the spirocyclizations and reagent design meeting green chemistry. As a consequence, we have discovered the new dinuclear iodoarene 1’ as a designer organocatalyst for in situ efficiently generating µ-oxo bridged hypervalent iodine species to perform the greener oxidative spirocylization of amides 2 (Eq. 1).8
In this study, we partially experienced the potent high reactivity of the isolated heterocyclic hypervalent iodine compound 1 as an oxidant (Eq. 2). It seems that our dinuclear compound 1 generally shows high reactivities in many types of hypervalent iodine mediated oxidations as well as the spirolactamizations as a potent replacement of the conventional mononuclear hypervalent iodine reagents, while a sufficient reactivity study has not yet been completed except for the one case involving the spirolactamization of the substrate 2a and other types of cyclizations.9,10 Based on this objective, we investigated the inherent reactivity of our heterocyclic dinuclear-type hypervalent iodine compound 1 regarding the oxidative spirolactamization of phenol amides 2 for more extensive substrates. The present study concludes that the reactivity strongly depends on the steric situation of the nitrogen group of the pre-spirocyclic substrates 2 during the interaction with compound 1.
RESULTS AND DISCUSSION
Our reported strategies in synthesizing spirocyclic compounds using hypervalent iodine reagents are based on the activation of phenols or side-chain functionalities for initiating the oxidations.4 As the first side-chain activation report, the spirocyclizations by the activation of the enamide part of the substituted phenols were developed in our laboratory, which were applied to the total synthesis of discorhabdin alkaloids.4g-i Later, the effective spirolactamizations of phenol derivatives 2-X starting from the oxidation of the side-chain nitrogen atom have been independently established by Kikugawa11 and Wardrop12 by utilizing the suitable N-directing methoxy and phthalimide groups (Scheme 1, X = OMe, N-phthanimide). The generated nitrenium ions would undergo cyclization and hydrolysis by ambient water, giving rise to the spirolactam products 3. This chemistry was nicely applied to the diverse syntheses of natural products and biologically active compounds, but in terms of the reaction chemistry, the main disadvantage requiring excess amounts of PIDA and PIFA is still required to achieve the satisfactory generation of nitrenium ions and to obtain good product yields. The reaction possibly becomes improved by employing a more reactive hypervalent iodine alternative.
The preparation of the heterocyclic dinuclear hypervalent iodine compound 1 in this study includes only two steps from a commercially available source, 1-iodo-3,5-dimethylbenzene (Scheme 2). It is a stable microcrystalline solid (mp 157 oC) compatible with storage in air at room temperature, if protected from light.13 The corresponding iodoarene precursor 1’ could be directly obtained by our oxidative biaryl coupling method.14 Subsequent treatment with SelectfluorTM (1-chloromethyl-4-fluoro-1,4-diazonia bicyclo[2.2.2]octane bis(tetrafluoroborate)) in a mixed solvent system of dilute acetic acid and acetonitrile15 nearly quantitatively produced the cyclic hypervalent iodine compound 1 from the crude precipitate of which after evaporation, the pure compound was obtained in 96% yield by trituration with hexane. Similarly, the heterocyclic compound 1 could be prepared from the precursor 1’ by the action of other typical oxidants for generation of hypervalent iodine species, i.e., peracetic acid (PAA) and m-chloroperbenzoic acid (mCPBA),5,6 in the presence of excess acetic acid. SelectfluorTM is the most prominent regarding the purity of the obtained compound 1 during the preparation. For structural elucidation, a sample suitable for an X-ray crystallographic analysis was grown by further recrystallization.16
For the obtained 1, we subsequently evaluated the reactivity toward representative substrates for the oxidative spirolactamization (Scheme 3 and Table 1). For a clear comparison, we selected the known phenol amides 2b-h already tested in the literature as authentic entries.8,11,12 Using the oxidant 1 at a slightly excess amount (1.1 x 1/2 equiv., which corresponds to 110 mol% iodine(III)), the reactions were performed in a mixture of trifluoroethanol (TFE)17 and dichloromethane (1/1 v/v) at room temperature in an open flask. Generally, the reactions could be completed within a few hours under mild conditions around the theoretical stoichiometry. Moreover, all the products 3b-h were more satisfactorily produced when compared to those using the conventional PIDA. Hence, the four- to six-membered spirocyclic lactams 3a-c were efficiently obtained by the treatment of the oxidant 1 (Eq. 2 and Scheme 3, Eq. 4). On the other hand, cyclization into seven-membered ring lactam was sluggish by this method. The protection of the phenolic oxygens is necessary in these reactions as the six- and four-membered products, 3b-OMe and 3c-OMe, were not obtained at all by treatment of the corresponding free phenol compounds.18 To better understand the higher reactivity of the dinuclear hypervalent iodine 1, it should be emphasized that lowering the reaction rate in the referenced cases of PIDA decreased yields by ~28% under the same
conditions for the same substrates. The propanamides 2d-f bearing substituents at the aromatic ring and side chain smoothly reacted to afford the corresponding spirolactams 3d-f (Table 1, entries 1-3). Due to the mild reaction conditions, the biaryl-derived phenol amides 2g and 2h also converted exclusively to the spirolactams 3g and 3h (entries 4 and 5) without causing any rearrangement process (e.x., dienone-phenol rearrangement), respectively. Evidently, the dinuclear compound 1 served as an efficient oxidizing agent for the carbon-nitrogen bond-forming spirocyclization event. In addition, most of it was recovered as the reduced form 1’ after the reactions by column chromatography eluting with hexane utilizing the very low polarity of the monovalent iodine 1’.
As the reaction involves the initial attack of the nitrogen atom of the substrates 2 on the hypervalent iodine,19 the observed reactivity differences between the dinuclear iodine 1 and conventional mononuclear reagent, i.e., PIDA, mostly depend on the rate of the ligand exchange step. Based on this consideration, the empirical bond lengths between the iodines and ligands of the two types of hypervalent iodine compounds in the crystallographic structures are summarized in Table 2.16,20 In the dinuclear compound 1, the hypervalent bonds are unequal and thus elongation of the I(III)-OAc bond occurs compared to those of PIDA (2.221 Å versus 2.165 Å). Thus, the µ-oxo 1 takes a more polarized structure and the iodine atoms are estimated to be rather cationic in a polar solvent, such as fluoroalcohols. Importantly, the strong secondary bonding between the iodine atom and carbonyl oxygen of the acetoxy group is present in the µ-oxo compound 1, also suggesting the enhanced positive character of the iodine atom.21 Therefore, we
reasoned that the high reactivity of the dinuclear iodine 1 might be due to the enhanced electrophilicity of the iodine atom and facile elimination of the acetoxy group caused by the extended bond length facilitating the interaction of the amide group and sequential ligand exchange.
Briefly, the dinuclear iodine 1 generally showed excellent reactivities for the oxidations of N-methoxy amides 2-OMe. We next turned our attention to the interactive nitrogen group of the substrates. Thus, the cyclization of the corresponding N-phthalimide of the phenol amide, 2a-Nphth (Eq. 3, Nphth = N- phthalimide), under the same reaction conditions was examined for the dinuclear iodine 1, and in this case, the formation of the expected product, 3a-Nphth, was, in turn, unexpectedly suppressed when compared to the reaction using PIDA (see ref. 11). After reacting for 3 hours, the product 3a-Nphth was thus obtained in only 61% yield. It appeared that the phthalimide group had an effect on the interaction with the iodine atom in compound 1 probably due to steric reasons. To clarify the characteristics, the reactivity and kinetics of the N-methoxy amide 2a-OMe and N-phthalimide 2a-Nphth toward our iodine compound 1 and conventional reagent were briefly checked by subjecting equimolar amounts of the two amides to the following competitive reaction conditions (Scheme 4, Eq. 5). For the dinuclear 1, it is interesting to observe that a clear preference for the N-methoxy amide 2a-OMe for providing the biased formation of the N-methoxy spirolactam 3a-OMe (3a-OMe/3a-Nphth = 11.4/1) was developed, while most of the other substrate 2a-Nphth remained unreacted. In contrast, when using the conventional PIDA instead, competitive reactions of the two substrates diminished the selectivity, and the spirolactams were produced
as a mixture in the relative ratios of 3.8/1 and 2.8/1 (average of two runs), respectively, under our standard and the reported conditions.11 Consequently, a new selectivity was confirmed for our dinuclear compound 1.
To summarize, our dinuclear iodine 1 seems to be inherently very reactive due to the high polarity of the iodine atoms, increasing the reaction rate of the hypervalent iodine oxidations and giving the products in remarkable yields, especially for the small substrates and functionalities. On the other hand, for a bulky substrate, the steric requirement arising from the introduced biaryl structure sometimes overrides the reactivity, which resulted in a rather decreasing rate of the reactions while possibly allowing the enhancement of the selectivity and molecular recognition of the hypervalent iodine.
CONCLUSION
In this study, we have extensively studied the unknown reactivity of the heterocyclic dinuclear hypervalent iodine compound 1 for the oxidative dearomatizing spirolactamizations. The high reactivity based on the high electrophilicity of the µ-oxo bridged iodine atoms allows fast generation of nitrenium ions from the N-methoxy amides, giving the spirolactam products in more satisfactory yields with the theoretical amount of the oxidant loading. At the same time, a unique selectivity, not observed for the conventional reagent, toward the nitrogen groups has emerged for the oxidant 1 during the transformation probably because of the change in the steric environment, based on the results of which the designer compound 1 as a chemoselective reagent in applications to some specific substrate and transformation is possible in the future study.22
EXPERIMENTAL
Melting point (mp) is uncorrected. All 1H- and 13C-NMR spectra of the products were measured in CDCl3 by spectrometers operating at 400 or 300 MHz (100 or 67.8 MHz for 13C NMR) at 25 oC. Chemical shifts of 1H-NMR were recorded in parts per million (ppm, δ) relative to tetramethylsilane (δ = 0.00 ppm) as an internal standard. Data are reported as follows: chemical shift in ppm (δ), multiplicity (s = singlet, d = doublet, t = triplet, q = quartet, brs = broad singlet, m = multiplet), coupling constant (J) in Hz, and integration. Chemical shifts of 13C-NMR were reported in ppm with the solvent as reference peak (CDCl3: δ = 77.0 ppm). Absorptions of infrared spectra (IR) are reported in reciprocal centimeters (cm-1) for representative peaks. High resolution mass measurements and elemental analysis were performed by the Elemental Analysis Section of Osaka University. Flash column chromatography was performed with Merck Silica Gel 60 (230-400 mesh) eluting with hexane and ethyl acetate for isolation of the products. Analytical thin-layer chromatography (TLC) was carried out on Merk Silica Gel F254 plates (0.25 mm). The spots and bands were detected by UV light of irradiation (254, 365 nm) and/or by staining with 5% phosphomolybdic acid followed by heating. Unless otherwise noted, all the experiments were carried out at room temperature in open flask.
Materials
A referred hypervalent iodine reagent, phenyliodine(III) diacetate (PIDA, PhI(OAc)2), is a commercial compound. SelectfluorTM is also commercially available. The starting substrates 2a-h for the spirolactamizations were prepared from the corresponding acid chlorides by condensation with methoxy amine hydrochloride or N-aminophthalimide according to the literatures.11 All other chemicals and solvents for the experiments and chromatography were obtained from commercial suppliers and used as received without further purification.
Preparation of µ-oxo bridged heterocyclic hypervalent iodine precursor 1’
To a stirred solution of 3,5-dimethyliodobenzene (1.62 g, 7.0 mmol) in CH2Cl2 (8.75 mL) was added dropwise a solution of phenyliodine(III) bis(trifluoroacetate) (PIFA, 1.51 g, 3.5 mmol) and BF3·Et2O (0.73 mL, 7.0 mmol) in CH2Cl2 (8.75 mL) under nitrogen atmosphere at -78 oC. The reaction mixture was stirred for 5 h at the same temperature. The reaction was quenched with saturated aqueous sodium hydrogen carbonate and the aqueous layer was extracted with CH2Cl2 several times. The combined organic layer was washed with brine and dried over anhydrous solid sodium sulfate. After filtration, removal of the solvent in vacuo followed by column chromatography of the residue on silica-gel (eluent: n-hexane) gave a pure biaryl compound 1’ (1.02 g, 2.2 mmol) in 63% yield.
2,2’-Diiodo-4,4’,6,6’-tetramethylbiphenyl (1’)14b
Colorless crystals; mp 108-111 oC; IR (KBr): 3014, 1599, 1541, 1035 cm-1; 1H-NMR (300 MHz, CDCl3): 1.95 (s, 6H), 2.31 (s, 6H), 7.06 (s, 2H), 7.62 (s, 2H) ppm; 13C-NMR (67.8 MHz, CDCl3): 20.4, 21.2, 100.7, 130.7, 136.9, 137.0, 139.0, 144.3 ppm.
Preparation of µ-oxo bridged heterocyclic hypervalent iodine compound 1
To a stirred solution of SelectfluorTM (2.69 g, 7.6 mmol) in MeCN (47.5 mL) was successively added acetic acid (17.1 mL) and the prepared 2,2’-diiodo-4,4’,6,6’-tetramethylbiphenyl 1’ (0.88 g, 1.9 mmol), and the mixture was stirred for overnight at room temperature. After removal of MeCN under reduced pressure, the residue was extracted with CH2Cl2 and then the organic solution was dried over anhydrous solid sodium sulfate. After evaporation of the solvent, the crude solid 1 was dissolved in minimal amount of CH2Cl2, which was added dropwise to hexane with stirring. The resulting suspension was filtered and solid was collected as pure µ-oxo bridged heterocyclic hypervalent iodine compound 1 (1.07 g, 1.8 mmol) in 96% yield.
The use of peracetic acid (PAA) instead of SelectfluorTM as oxidant also afforded the compound 1 in similar yield.8
5,7-Bis(acetyloxy)-5,7-dihydro-1,3,9,11-tetramethydibenzo[d,f][1,3,2]diiodoxepin (1)
Colorless crystals; mp 157 oC; IR (KBr): 1649, 1559, 1018, 750 cm-1; 1H-NMR (400 MHz, CDCl3): 1.85 (s, 6H), 2.16 (s, 6H), 2.46 (s, 6H), 7.38 (s, 2H), 7.86 (s, 2H) ppm; 13C-NMR (100 MHz, CDCl3): 21.2 (x 2), 21.4, 127.0, 133.4, 135.2, 137.7, 139.2, 142.8, 177.6 ppm.
Crystals of the heterocyclic hypervalent iodine compound 1 compatible for X-ray analysis was obtained by recrystallization from MeCN-hexane. For crystallographic data of 1 in CIF, see CCDC 779814.16
General procedure for the oxidative spirolactamization of phenol ether amides 2 using heterocyclic hypervalent iodine compound 1
To a stirred solution of phenol amide 2a (41.2 mg, 0.20 mmol) in trifluoroethanol (2 mL) and CH2Cl2 (2 mL) was added the prepared heterocyclic hypervalent iodine compound 1 (65.6 mg, 0.11 mmol) at once at room temperature in open flask. After 30 min, the solvents were removed under reduced pressure. To the residue CH2Cl2 was added and the extract was then washed with water, saturated aqueous sodium hydrogen carbonate, and dried over anhydrous solid sodium sulfate. After evaporation, a pure spirolactam product 3a (39.4 mg, 0.196 mmol, 98%) was obtained from the residue by column chromatography on silica-gel (hexane/EtOAc = 1/2).
The physical and spectral data of all the products 3a-h well matched those previously reported.
1-Methoxy-1-azaspiro[4.5]deca-6,9-diene-2,8-dione (3a)
Colorless crystals; mp 130-131 oC (lit.11b mp 129-131 oC); IR (KBr): 2358, 2341, 1743, 1672, 1278, 711 cm-1; 1H-NMR (300 MHz, CDCl3): 2.18 (t, J = 7.8 Hz, 2H), 2.56 (t, J = 7.8 Hz, 2H), 3.80 (s, 3H), 6.39 (d, J = 10.2 Hz, 2H), 6.84 (d, J = 10.2 Hz, 2H) ppm; 13C-NMR (67.8 MHz, CDCl3): 25.9, 27.5, 61.9, 65.3, 131.1, 147.2, 171.7, 184.4 ppm.
1-Methoxy-1-azaspiro[5.5]undeca-7,10-diene-2,9-dione (3b)
Colorless crystals; mp 112-113 oC; IR (KBr): 3506, 2939, 2358, 2341, 1668, 1392, 862 cm-1; 1H-NMR (300 MHz, CDCl3): 1.91-1.99 (m, 2H), 2.03-2.06 (m, 2H), 2.61 (d, J = 6.3 Hz, 2H), 3.74 (s, 3H), 6.34 (d, J = 9.9 Hz, 2H), 6.94 (d, J = 9.9 Hz, 2H) ppm; 13C-NMR (67.8 MHz, CDCl3): 18.3, 33.2, 35.6, 63.6, 64.5, 130.0, 148.7, 168.1, 184.4 ppm.
1-Methoxy-1-azaspiro[3.5]nona-5,8-diene-2,7-dione (3c)
Colorless crystals; mp 109-110 oC; IR (KBr): 3523, 2941, 1770, 1666, 1402 cm-1; 1H-NMR (300 MHz, CDCl3): 2.98 (s, 2H) 3.78 (s, 3H), 6.47 (d, J = 9.9 Hz, 2H), 6.93 (d, J = 9.9 Hz, 2H) ppm; 13C-NMR (67 MHz, CDCl3): 43.5, 60.4, 65.4, 132.3, 145.6, 162.1, 184.2 ppm.
1-Methoxy-4,6-dimethyl-1-azaspiro[4.5]deca-6,9-diene-2,8-dione (3d)
Colorless crystals; mp 92-93 oC; IR (KBr): 3500, 2972, 1714, 1666, 1286 cm-1; 1H-NMR (300 MHz, CDCl3): 0.97 (d, J = 6.9 Hz, 3H), 2.10 (s, 3H), 2.22 (dd, J = 16.5, 10.2 Hz, 1H), 2.55-2.63 (m, 1H), 2.72 (dd, J = 16.5, 8.4 Hz, 1H), 3.80 (s, 3H), 6.34 (s, 1H), 6.44 (dd, J = 10.2, 2.1 Hz, 1H), 6.75 (d, J = 10.2 Hz, 1H) ppm; 13C-NMR (67.8 MHz, CDCl3): 14.6, 18.5, 33.4, 34.8, 63.8, 68.6, 131.4, 131.9, 145.7, 155.7, 171.9, 184.5 ppm.
7-Acetyl-1-methoxy-1-azaspiro[4.5]deca-6,9-diene-2,8-dione (3e)
Colorless oil; IR (KBr): 2939, 1693, 1666, 1359, 1257 cm-1; 1H-NMR (300 MHz, CDCl3): 2.19-2.26 (m, 2H) 2.57 (s, 3H), 2.59-2.63 (m, 2H), 3.78 (s, 3H), 6.41 (d, J = 10.2 Hz, 1H), 6.87 (dd, J = 10.2, 3.0 Hz, 1H), 7.42 (d, J = 3.0 Hz, 1H) ppm; 13C-NMR (67 MHz, CDCl3): 25.6, 27.0, 30.5, 61.9, 65.0, 131.2, 139.2, 146.2, 150.6, 171.1, 181.9, 196.6 ppm.
1-Methoxy-3-methyl-1-azaspiro[4.5]deca-6,9-diene-2,8-dione (3f)
Colorless crystals; mp 105-107 oC; IR (KBr): 2938, 1719, 1672, 1250, 714 cm-1; 1H-NMR (400 MHz, CDCl3): 1.29 (d, J = 7.1 Hz, 3H), 1.82 (dd, J = 13.2, 9.5 Hz, 1H), 2.28 (dd, J = 13.2, 9.0 Hz, 1H), 2.58-2.64 (m, 1H), 3.75 (s, 3H), 6.34 (d, J = 10.7 Hz, 2H), 6.78 (d, J = 10.8, 2H) ppm; 13C-NMR (100 MHz, CDCl3): 17.2, 32.8, 37.1, 61.6, 65.8, 131.4, 131.9, 147.4, 149.1, 174.7, 185.0 ppm; HRMS (EI): calcd for C19H18S (M)+: 278.1129, found 278.1124.
2'-Methoxyspiro[2,5-cyclohexadiene-1,1'-[1H]isoindole]-3',4(2'H)-dione (3g)
Colorless crystals; mp 204-206 oC; IR (KBr): 3039, 2941, 1719, 1671, 1605 cm-1; 1H-NMR (300 MHz, CDCl3): 3.96 (s, 3H), 6.55 (d, J = 9.5 Hz, 2H), 6.67 (d, J = 9.5 Hz, 2H), 7.23 (d, J = 7.6 Hz, 1H), 7.59-7.65 (m, 2H), 7.95 (d, J = 7.6 Hz, 1H) ppm; 13C-NMR (67 MHz, CDCl3): 65.1, 66.1, 122.7, 124.9, 129.3, 130.1, 131.6, 133.5, 139.3, 145.6, 165.4, 184.6 ppm.
2'-Methoxyspiro[2,5-cyclohexadiene-1,1'(2'H)-isoquinoline]-3',4(4'H)-dione (3h)
Pale-yellow crystals; mp 209-210 oC; IR (KBr) : 2936, 1667, 1629, 1604, 1350, 1060, 683 cm-1; 1H-NMR (400 MHz, CDCl3): 3.79 (s, 3H), 3.87 (s, 2H), 6.34 (d, J = 9.9 Hz, 2H), 6.78 (d, J = 9.9 Hz, 2H), 7.05-7.29 (m, 4H) ppm; 13C-NMR (100 MHz, CDCl3): 36.3, 64.8, 67.0, 126.3, 127.6, 128.5, 129.2, 129.3, 130.2, 147.5, 166.1, 184.7 ppm.
Cross-over experiments between N-methoxy amide 2a-OMe and N-phthalimide amide 2a-NPhth
To a stirred solution including equimolar amount of N-methoxy amide 2a-OMe (20.9 mg, 0.10 mmol) and N-phthalimide amide 2a-NPhth (33.7 mg, 0.10 mmol) in trifluoroethanol (1 mL) and CH2Cl2 (1 mL) was added the heterocyclic hypervalent iodine compound 1 (29.8 mg, 0.05 mmol) or PIDA (32.2 mg, 0.10 mmol) at once at room temperature in open flask. The reaction progress was checked by TLC. After the reaction, the solvents were evaporated to dryness and the residue was subjected to 1H NMR measurement to determine the ratio of 3a-OMe and 3a-NPhth. The above-mentioned standard purification procedure afforded the corresponding polar spirolactam product 3a-OMe (Rf = 0.14 (hexane/EtOAc = 1/2)) and less polar 3a-NPhth (Rf = 0.47 (hexane/EtOAc = 1/2)), respectively, in pure forms with partial recovery of the starting materials 2a-OMe and 2a-NPhth. The selectivity of the products, 3a-OMe and 3a-NPhth, after the isolation was in good agreement with the calculated value determined by the 1H NMR measurement of the crude reaction mixture.
1-(1,3-Dihydro-1,3-dioxo-2H-isoindol-2-yl)-1-azaspiro[4.5]deca-6,9-diene-2,8-dione (3a-NPhth)11c
Colorless crystals; mp 255-258 oC (lit.11c mp 255-258 oC); IR (KBr): 1805, 1750, 1730, 1675, 715 cm-1; 1H-NMR (300 MHz, CDCl3): 2.46 (t, J = 8.0 Hz, 2H), 2.80 (t, J = 8.0 Hz, 2H), 6.27 (dd, J = 10.1, 2.7 Hz, 2H), 7.12 (dd, J = 10.1, 2.7 Hz, 2H), 7.78-7.84 (m, 2H), 7.85-7.91 (m, 2H) ppm; 13C-NMR (67 MHz, CDCl3): 21.6, 24.0, 57.5, 119.0, 124.5, 125.2, 129.9, 141.7, 159.4, 166.0, 179.1 ppm.
ACKNOWLEDGEMENTS
This work was supported by a Grant-in-Aid for Scientific Research (A) and Encouragement of Young Scientists (A) from JSPS, a Grant-in-Aid for Scientific Research on Innovative Areas “Advanced Molecular Transformations by Organocatalysts” from MEXT, and Ritsumeikan Global Innovation Research Organization (R-GIRO) project. T.D. is thankful for financial support from the Asahi Glass Foundation and the Industrial Technology Research Grant Program from NEDO of Japan.
References
1. (a) F. Beaulieu, J. Arora, U. Veith, N. J. Taylor, B. J. Chapell, and V. Snieckus, J. Am. Chem. Soc., 1996, 118, 8727; CrossRef (b) L. Giraud and P. Renaud, J. Org. Chem., 1998, 63, 9162; CrossRef (c) B. Alcaide, I. M. Rodríguez-Campos, J. Rodríguez-López, and A. Rodríguez-Vicente, J. Org. Chem., 1999, 64, 5377. CrossRef
2. For some recent reports, see: (a) J. Artacho, E. Ascic, T. Rantanen, J. Karlsson, C.-J. Wallentin, R. Wang, O. F. Wendt, M. Harmata, V. Snieckus, and K. Wärnmark, Chem. Eur. J., 2012, 18, 1038; CrossRef (b) J. Artacho, E. Ascic, T. Rantanen, C.-J. Wallentin, S. Dawaigher, K.-E. Bergquist, M. Harmata, V. Snieckus, and K. Wärnmark, Org. Lett., 2012, 14, 4706; CrossRef (c) S. A. Glover and A. A. Rosser, J. Org. Chem., 2012, 77, 5492; CrossRef (d) A. Witosińska, B. Musielak, P. Serda, M. Owińska, and B. Rys, J. Org. Chem., 2012, 77, 9784; CrossRef (e) B. Sliter, J. Morgan, and A. Greenberg, J. Org. Chem., 2011, 76, 2770; CrossRef A recent review: (f) M. Szostak and J. Aubé, Org. Biomol. Chem., 2011, 9, 27. CrossRef
3. For selected reports of spirolactamization methods appeared in recent few years, see: (a) R. Gámez- Montaño, T. Ibarra-Rivera, L. El Kaïm, and L. D. Miranda, Synthesis, 2010, 1285; CrossRef (b) M. Li and D. J. Dixon, Org. Lett., 2010, 12, 3784; CrossRef (c) B. Alcaide, P. Almendros, T. Martínez del Campo, and R. Carrascosa, Eur. J. Org. Chem., 2010, 4912; CrossRef (d) Z. Xu, K. Huang, T. Liu, M. Xie, and H. Zhang, Chem. Commun., 2011, 47, 4923; CrossRef (e) J. Li, Y. Liu, C. Li, H. Jie, and X. Jia, Green Chem., 2012, 14, 1314; CrossRef (f) K. Jiang, B. Tiwari, and Y. R. Chi, Org. Lett., 2012, 14, 2382; CrossRef (g) I. Allous, S. Comesse, M. Sanselme, and A. Daïch, Eur. J. Org. Chem., 2011, 5303; CrossRef (h) T. Piou, L. Neuville, and J. Zhu, Org. Lett., 2012, 14, 3760; CrossRef (i) S.-W. Duan, Y. Li, Y.-Y. Liu, Y.-Q. Zou, D.-Q. Shi, and W.-J. Xiao, Chem. Commun., 2012, 48, 5160; CrossRef (j) H. Lv, B. Tiwari, J. Mo, C. Xing, and Y. R. Chi, Org. Lett., 2012, 14, 5412; CrossRef (k) C. Hempel, N. M. Weckenmann, C. Maichle-Moessmer, and B. J. Nachtsheim, Org. Biomol. Chem., 2012, 10, 9325. CrossRef
4. For selected examples of our primary works on spirocyclizations of phenols and related compounds, see: (a) Y. Tamura, T. Yakura, J. Haruta, and Y. Kita, J. Org. Chem., 1987, 52, 3927; CrossRef (b) Y. Kita, H. Tohma, K. Kikuchi, M. Inagaki, and T. Yakura, J. Org. Chem., 1991, 56, 435; CrossRef (c) Y. Kita, T. Takada, M. Gyoten, H. Tohma, M. H. Zenk, and J. Eichhorn, J. Org. Chem., 1996, 61, 5857; CrossRef (d) Y. Kita, M. Arisawa, M. Gyoten, M. Nakajima, R. Hamada, H. Tohma, and T. Takada, J. Org. Chem., 1998, 63, 6625; CrossRef (e) H. Hamamoto, Y. Shiozaki, H. Nambu, K. Hata, H. Tohma, and Y. Kita, Chem. Eur. J., 2004, 10, 4977; CrossRef (f) K. Hata, H. Hamamoto, Y. Shiozaki, and Y. Kita, Chem. Commun., 2005, 2465; CrossRef (g) Y. Kita, T. Yakura, H. Tohma, K. Kikuchi, and Y. Tamura, Tetrahedron Lett., 1989, 30, 1119; CrossRef (h) Y. Kita, H. Tohma, M. Inagaki, K. Hatanaka, and T. Yakura, J. Am. Chem. Soc., 1992, 114, 2175; CrossRef (i) H. Tohma, Y. Harayama, M. Hashizume, M. Iwata, Y. Kiyono, M. Egi, and Y. Kita, J. Am. Chem. Soc., 2003, 125, 11235. CrossRef
5. (a) T. Dohi, A. Maruyama, M. Yoshimura, K. Morimoto, H. Tohma, and Y. Kita, Angew. Chem. Int. Ed., 2005, 44, 6193; CrossRef (b) T. Dohi, A. Maruyama, Y. Minamitsuji, N. Takenaga, and Y. Kita, Chem. Commun., 2007, 1224; CrossRef (c) T. Dohi, Y. Minamitsuji, A. Maruyama, S. Hirose, and Y. Kita, Org. Lett., 2008, 10, 3559; CrossRef (d) Y. Minamitsuji, D. Kato, H. Fujioka, T. Dohi, and Y. Kita, Aust. J. Chem., 2009, 62, 648; CrossRef (e) T. Dohi, A. Maruyama, N. Takenaga, K. Senami, Y. Minamitsuji, H. Fujioka, S. B. Cämmerer, and Y. Kita, Angew. Chem. Int. Ed., 2008, 47, 3787; CrossRef (f) T. Dohi, N. Takenaga, T. Nakae, Y. Toyoda, M. Yamasaki, M. Shiro, H. Fujioka, A. Maruyama, and Y. Kita, J. Am. Chem. Soc., 2013, 135, 4558; CrossRef Account: (g) T. Dohi and Y. Kita, Chem. Commun., 2009, 2073. CrossRef
6. Recyclable reagents for the spirocyclzations: (a) H. Tohma, A. Maruyama, A. Maeda, T. Maegawa, T. Dohi, M. Shiro, T. Morita, and Y. Kita, Angew. Chem. Int. Ed., 2004, 43, 3595; CrossRef (b) T. Dohi, A. Maruyama, M. Yoshimura, K. Morimoto, H. Tohma, M. Shiro, and Y. Kita, Chem. Commun., 2005, 2205; CrossRef (c) T. Dohi, Chem. Pharm. Bull., 2010, 58, 135. CrossRef
7. μ-Oxo bridged dimers: (a) N. Takenaga, T. Uchiyama, D. Kato, H. Fujioka, T. Dohi, and Y. Kita, Heterocycles, 2011, 82, 1327; CrossRef (b) T. Dohi, T. Uchiyama, D. Yamashita, N. Washimi, and Y. Kita, Tetrahedron Lett., 2011, 52, 2212; CrossRef (c) T. Dohi, T. Nakae, N. Takenaga, T. Uchiyama, K. Fukushima, H. Fujioka, and Y. Kita, Synthesis, 2012, 44, 1183. CrossRef
8. T. Dohi, N. Takenaga, K. Fukushima, T. Uchiyama, D. Kato, M. Shiro, H. Fujioka, and Y. Kita, Chem. Commun., 2010, 46, 7697. CrossRef
9. For the reactivities of the μ-oxo bridged heterocyclic hypervalent iodine compound 1 toward alkynes in the presence of activators, see: (a) T. Dohi, D. Kato, R. Hyodo, D. Yamashita, M. Shiro, and Y. Kita, Angew. Chem. Int. Ed., 2011, 50, 3784; CrossRef (b) T. Dohi, T. Nakae, Y. Ishikado, D. Kato, and Y. Kita, Org. Biomol. Chem., 2011, 9, 6899. The inherent reactivity of the compound 1 in the absence of activator was not investigated. CrossRef
10. For a related heterocyclic hypervalent iodine compound based on binapthalene structure, see: M. Ochiai, Y. Takaoka, Y. Masaki, Y. Nagao, and M. Shiro, J. Am. Chem. Soc., 1990, 112, 5677. In this report, reactivity of the μ-oxo compound was not investigated. CrossRef
11. (a) E. Miyazawa, T. Sakamoto, and Y. Kikugawa, Heterocycles, 2003, 59, 149; CrossRef (b) E. Miyazawa, T. Sakamoto, and Y. Kikugawa, J. Org. Chem., 2003, 68, 5429; CrossRef (c) Y. Kikugawa, A. Nagashima, T. Sakamoto, E. Miyazawa, and M. Shiiya, J. Org. Chem., 2003, 68, 6739; CrossRef A review: (d) Y. Kikugawa, Heterocycles, 2009, 78, 571. CrossRef
12. (a) D. J. Wardrop and A. Basak, Org. Lett., 2001, 3, 1053; CrossRef (b) D. J. Wardrop and W. Zhang, Org. Lett., 2001, 3, 2353; CrossRef (c) D. J. Wardrop, C. L. Landrie, and J. A. Ortíz, Synlett, 2003, 1352. CrossRef
13. Y. Kita and T. Dohi, Jpn. Kokai Tokkyo Koho (2012), JP 2012051846 A 20120315.
14. (a) H. Tohma, H. Morioka, S. Takizawa, M. Arisawa, and Y. Kita, Tetrahedron, 2001, 57, 345; CrossRef (b) H. Tohma, M. Iwata, T. Maegawa, and Y. Kita, Tetrahedron Lett., 2002, 43, 9241. CrossRef
15. C. Ye, B. Twamley, and J . M. Shreeve, Org. Lett., 2005, 7, 3961. CrossRef
16. CCDC 779814 contains the crystallographic data of the heterocyclic hypervalent iodine compound 1. The data can be obtained free of charge from The Cambridge Crystallographic Data Centre via www.ccdc.cam.ac.uk/data_ request/cif.
17. The fluoroalcohols are useful in many hypervalent iodine oxidations. For account and reviews discussing the property and utility, see: (a) Y. Kita, T. Takada, and H. Tohma, Pure Appl. Chem., 1996, 68, 627; CrossRef (b) L. Eberson, M. P. Hartshorn, O. Persson, and F. Radner, Chem. Commun., 1996, 2105; CrossRef (c) J.-P. Bégué, D. Bonnet-delpon, and B. Crousse, Synlett, 2004, 18; CrossRef (d) T. Dohi, N. Yamaoka, and Y. Kita, Tetrahedoron, 2010, 66, 5775. CrossRef
18. Similar limitation was reported in literatures in alternative spirolactamization studies: (a) S. Canesi, P. Belmont, D. Bouchu, L. Rousset, and M. A. Ciufolini, Tetrahedron Lett., 2002, 43, 5193; CrossRef (b) S. Canesi, D. Bouchu, and M. A. Ciufolini, Angew. Chem. Int. Ed., 2004, 43, 4336. CrossRef
19. No detection of the iodanyl amide (N-I(III)) intermediates during the reactions in spectroscopic study would suggest first ligand exchange of amides toward hypervalent iodine atom as rate-determining step.
20. X-Ray crystallographic data of PIDA: C.-K. Lee, T. C. W. Mak, W.-K. Li, and J. F. Kimer, Acta Crystallogr. B, 1977, B33, 1620. CrossRef
21. Similar observation of secondary bonding was reported in μ-oxo dimers of PIDA and PIFA: (a) N. W. Alcock, R. M. Countryman, S. Esperås, and J. F. Sawyer, J. Chem. Soc., Dalton Trans., 1979, 854; CrossRef (b) N. W. Alcock, W. D. Harrison, and C. Howes, J. Chem. Soc., Dalton Trans., 1984, 1709; CrossRef (c) R. Bell and K. J. Morgan, J. Chem. Soc., 1960, 1209. CrossRef
22. Recently, our dinuclear-type hypervalent iodine compounds are being utilized in other useful transformations. See: (a) A. P. Antonchick, R. Samanta, K. Kulikov, and J. Lategahn, Angew. Chem. Int. Ed., 2011, 50, 8605; CrossRef (b) R. Samanta, J. O. Bauer, C. Strohmann, and A. P. Antonchick, Org. Lett., 2012, 14, 5518; CrossRef (c) C. Röben, J. A. Souto, E. C. Escudero-Adán, and K. Muñiz, Org. Lett., 2013, 15, 1008. CrossRef