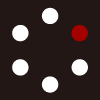
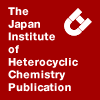
HETEROCYCLES
An International Journal for Reviews and Communications in Heterocyclic ChemistryWeb Edition ISSN: 1881-0942
Published online by The Japan Institute of Heterocyclic Chemistry
e-Journal
Full Text HTML
Received, 25th June, 2013, Accepted, 28th August, 2013, Published online, 4th September, 2013.
DOI: 10.3987/COM-13-S(S)49
■ Constituents from the Rhizomes of Curcuma comosa and Their Wnt Signal Inhibitory Activities
Rolly G. Fuentes, Kazufumi Toume, Midori A. Arai, Takashi Koyano, Thaworn Kowithayakorn, and Masami Ishibashi*
Department of Natural Products Chemistry, Graduate School of Pharmaceutical Science, Chiba University, 1-8-1 Inohana, Chuo, Chiba, Japan
Abstract
Activity-guided fractionation of the Curcuma comosa rhizome MeOH extract that inhibited Wnt signal activity led to the characterization of two new diarylheptanoids (1 and 2) together with six known diarylheptanoids (3-8) and two known sesquiterpenoids (9 and 10). Their structures were identified by 1D and 2D NMR, HRESIMS, optical rotation, and chemical evidences. Compounds 1 and 8 exhibited dose-dependent inhibitory activity against β-catenin/TCF transcriptional activity.The Wnt pathway is one of the signaling pathways that plays a significant role in the regulation of several embryonic development and cellular processes.1 When the Wnt signal is active, β-catenin accumulates, translocates to the nucleus, and activates T-cell factor/lymphoid enhancing factor (TCF/LEF) for the transcription of target genes.2 However, abnormal activation of this pathway may lead to the development of various diseases and abnormalities. Most cancer cells, particularly colon and melanoma cancer cells, harbor mutations upregulate the Wnt signaling pathway.3,4 Furthermore, deregulated activation of the Wnt pathway has been linked to the incidence of type II diabetes, and contributes to abnormalities including high bone mass and oligodontia.1,4 Thus, inhibition of the Wnt signal may present promising therapeutic strategies to treat or manage these diseases.
Several small molecules have been reported to inhibit the Wnt signal. Our group previously reported natural products with potent inhibitory activity against the β-catenin transcriptional pathway.5-7 In our continuing search for bioactive natural products exhibiting effects on Wnt signaling, we recently identified Curcuma comosa as a hit plant in our screening program. C. comosa is widely used in Thailand as a medicinal plant to treat inflammation. Previous chemical studies showed that the rhizome of this plant contained diarylheptanoids and sesquiterpenes.8,9 Pharmacological studies also demonstrated that it had estrogenic10 and anti-inflammatory activities,11 which were attributed to the presence of diarylheptanoids. In this study, we isolated and characterized the constituents of C. comosa with potent inhibitory activity of the Wnt signal. Activity-guided isolation with a luciferase cell-based assay system to evaluate the inhibition of β-catenin/TCF transcriptional activity yielded two new diarylheptanoids (compounds 1 and 2).
Based from our screening using the SuperTOPFlash assay, the MeOH rhizome extract of C. comosa showed 82% inhibition of TOP activity at 50 μg/mL. The viability of STF/293 reporter cells at the same concentration was 84%. Through activity-guided isolation, the active compounds were purified using silica gel, ODS column chromatography, and HPLC to give two new (1 and 2) together with six known (3-8) diarylheptanoids and two known sesquiterpenoids, zederone (9) and aerugidiol (10).
Compound 1 was isolated as a colorless solid and gave a molecular formula of C21H26O5 as determined by HRESIMS (m/z 381.1634 C21H26O5Na, Δ -4.4 mmu). Both the 1H and 13C NMR data (Table 1) of 1 are consistent with published data of its reported enantiomer (ent-1).12 1 gave a negative optical rotation, which suggests a 3S,5S configuration, while its reported 3R,5R enantiomer (ent-1) had a positive value. Deacetylation of 1 gave a product whose NMR data were identical with those of hannokinol.13,14 Optical rotation of the deacetylated product was determined using reversed phase HPLC equipped with an optical rotation detector (ORD) and its response was compared with the isolated (-)-hannokinol (7). The 3R,5R form (ent-7) was previously reported to be [α]26D +4.0 (c 0.10 MeOH),14 while the 3S,5S form (7) was found to be [α]25D -19.0.13 Compound 7 and the deacetylated product of 1 both gave a negative response in the ORD. These findings suggest that the deacetylated product of 1 had a negative optical rotation. Therefore, the structure of compound 1 was determined to be (-)-(3S,5S)-3-acetoxy-5-hydroxy-1,7-bis(4-hydroxyphenyl)heptane.
Compound 2 was obtained as a mixture with a minor unknown compound. An intense peak at m/z 399.1762 ([M+Na]+, C21H28O6Na Δ -2.1 mmu) was observed in HRESIMS, which is consistent with the molecular formula of C21H28O6. The 1H NMR spectra of 2 and the unknown compound exhibited some overlapping peaks, which indicated that they are structurally-related compounds. A singlet peak at δ 3.82 (6H) was due to the two symmetric methoxy groups and were assigned at C-3’ and C-5’. The presence of two symmetric benzene protons was indicated by the correlations observed between a singlet proton peak at δ 6.39 and carbon peak at δ 104.9 in both HMBC (Figure 1) and HMQC. They were assigned at H-2’ and H-6’. The two doublets at δ 6.99 (J = 8.3 Hz) and 6.73 (J = 8.3 Hz) suggested the presence of 1,4-disubstituted aromatic protons and were assigned to four aromatic protons, H-2”/H-6” and H-3”/H-5”, respectively. From these results, 2 was assigned as 3,5-dihydroxy-1-(4-hydroxy-3,5-dimethoxyphenyl)-7-(4-hydroxyphenyl)heptane.
Acetonidation of compound 2 using Rychnovsky’s method yielded its acetonide derivative 2a. The HMQC data of 2a showed correlations between the two methyl peaks at δ 1.32 and δ 1.62, and 13C peaks at δ 21.9 and δ 32.5, respectively. These results suggested that 2a was produced from a syn-1,3-diol.15 An intense peak at m/z 439.2070 ([M+Na]+, C24H32O6Na, Δ -2.7 mmu) was observed based on HRESIMS and corresponded to 2a. The absolute stereochemistry of 2 remains undefined.
The structure of the six known diarylheptanoids (5R)-5-hydroxy-7-(4-hydroxyphenyl)-1-(4-hydroxy-3-methoxyphenyl)-3-heptanone (3),12,16 (3R,5R)-3,5-diacetoxy-1,7-bis(3,4-di- hydroxyphenyl)heptane (4),17 (3R,5R)-3,5-diacetoxy-1-(3,4-dihydroxyphenyl)-7-(4-hydroxyphenyl)hep-tane (5),12 (5S)-5-hydroxy-1,7-bis(4-hydroxyphenyl)heptan-3-one (6),18,19 (-)-hannokinol (7),13 1,7-bis(4-hydroxyphenyl)hepta-4E,6E-dien-3-one (8),20 and two known sesquiterpenoids, zederone (9)21 and aerugidiol (10)22,23 were identified by comparing their spectral values and optical rotations with those in the literature.
The Wnt signal inhibitory activities of the characterized compounds were determined using the luciferase reporter assay described previously.5 Quercetin (IC50 = 30.4 µM),5 which was previously shown to possess β-catenin/TCF transcriptional inhibitory activity,23 was used as a positive control. Compounds 1, 7, and 8 showed the highest inhibitory activity in the SuperTOPflash assay with IC50 values of 3.0, 1.0, and 2.9 μM, respectively. However, only 1 and 8 did not affect SuperFOPflash activity up to 5 μM, which suggests that they have inhibitory activity against β-catenin/TCF transcriptional pathway (Figure 2). The other diarylheptanoids (2-7) as well as zederone (9) also showed dose-dependent inhibitory activity against the SuperTOPflash assay; however, since their IC50 values were higher than 10 μM (data not shown), they were not subjected to further assays. Aerugidiol (10), on the other hand, was inactive.
Since the assay involved the addition of LiCl, an inhibitor of glycogen synthase kinase-3 (GSK3), the data may suggest that 1 and 8 act downstream of the Wnt signaling pathway or on β-catenin itself. However, western blot analysis using SW480 cells showed that β-catenin levels in both cytoplasmic and nuclear fractions were not affected. Some curcumin derivatives exhibiting Wnt/β-catenin pathway inhibitory activity were also reported to have no effect on β-catenin levels. Instead, they showed to decrease the level of p300, which is a positive regulator of the Wnt signal pathway.24 The FMCA assay was used to determine the effect of 1 and 8 on the viability of colon cancer cells, SW480, HCT116, and DLD1. Several studies previously reported that inhibiting β-catenin/TCF transcriptional activity decreased the viability of colon cancer cells.24,25 However, after 24 hr incubation, neither compounds exhibited inhibitory activity on the growth of these three colon cancer cells.
In conclusion, we isolated two new diarylheptanoids alongside six known diarylheptanoids and two known sesquiterpenoids. Our results indicate that although compounds 1 and 8 showed inhibitory activity against the Wnt signal, they did not affect the proliferation of various colon cancer cells. Another mechanism also involving β-catenin may compensate for the loss of β-catenin/TCF transcriptional activity. Or, cell permeability of the compounds may provide an explanation why level of β-catenin and proliferation of the cancer cells were not affected.
EXPERIMENTAL
General Experimental Procedure. 1H and 13C NMR spectra were recorded on JEOL JNM-ECA600 spectrometers with deuterated solvent. HRESIMS were measured on a JEOL JMS-T100LP spectrometer. Optical rotation was determined with a JASCO P-1020 polarimeter. UV spectra were obtained in a Shimadzu UV mini-1240 spectrometer. IR spectra were measured on ATR in a JASCO FTIR 230 spectrophotometer.
Plant Materials. The rhizome of C. comosa was collected from Khon Kaen, Thailand and identified by T. Kowithyakorn. A voucher specimen (7-196) was deposited in our laboratory.
Cell culture. The STF/293 cell line was a generous gift from Prof. Jeremy Nathans (John Hopkins Medical School); HEK293, HCT116, and DLD1 cells were purchased from ATCC; SW480 cells were derived from the Institute of Development, Aging, and Cancer, Tohoku University. STF/293, HEK293, SW480, DLD1, and HCT116 were cultured in Dulbecco’s modified Eagle medium (DMEM) with 10% FBS. Cultures were maintained in a humidified incubator at 37 oC in 5% CO2/95% air.
Luciferase assay. TCF/β-catenin transcriptional activity was determined using the method previously described.5 STF/293 cells (3 x 104), which were stably transfected with SuperTOPflash, were seeded into 96-well plates and, after 24 h incubation, treated with compounds combined with 15 mM LiCl. After 24 h, cells were lysed and luciferase activity was measured. HEK293 cells (1 x 105) were briefly split into 24-well plates for the control reporter assay (FOPFlash). After 24 h, cells were transfected with 1 μg of the luciferase reporter construct (SuperFOPflash) and 2 ng of pRL-CMV (Promega, USA) for normalization. At 3 h post-transfection, the compounds combined with 15 mM LiCl were added. Cells were lysed after 24 h incubation, and luciferase activity was measured using the Dual-Glo Luciferase Assay System (Promega).
Cell viability assay. Cell viability was measured using the FMCA assay.26 Cells were inoculated into 96-well plates for 24 h. Compounds were then added and incubated for another 24 h. After incubation, they were treated with fluorescein diacetate (Wako) in PBS buffer. Fluorescence was detected after 1 h incubation.
Isolation of the constituents. The dried rhizome of C. comosa (226 g) was extracted using MeOH. The MeOH crude extract (6.6 g) of C. comosa was directly subjected to silica gel column chromatography (φ50 x 270 mm) eluting with the hexane-EtOAc gradient system (1:0 to 0:1) to give fractions 1A-1L. Fraction 1E (76.5 mg) was recrystallized to give compound 9 (28.4 mg). Fraction 1J (548 mg), which showed high activity on the TOP assay, was then subjected to ODS flash column chromatography (φ25 x 180 mm) to yield fractions 3A-3H. Active fraction 3D (59 mg) was further purified using silica gel column chromatography (φ25 x 170 mm; CHCl3:MeOH gradient system (1:0 - 0:1)) to give compound 4 (11.2 mg). Another fraction from 3D was further purified by preparative HPLC [Cosmosil 5C18-AR-II; φ10 x 250 mm; 60% MeOH] to give compounds 1 (5.7 mg) and 5 (4.9 mg). Fraction 3B (58.2 mg) was subjected to flash silica column chromatography (φ25 x 230 mm) using CHCl3: MeOH (1:0 to 0:1) to give fractions 6A-6H. Fraction 6B was identified as compound 10 (6.2 mg). Fraction 6F was further purified by preparative HPLC [Cosmosil 5C18-AR-II; φ10 x 250 mm; 53% MeOH] to afford compound 6 (2.9 mg). Fraction 6C gave compound 3 (1.1 mg) after purification by HPLC [Cosmosil 5C18-AR-II; φ10 x 250 mm; 45% MeOH]. The active fraction 1K was subjected to ODS flash column chromatography (φ45 x 180 mm) and eluted with increasing MeOH (67-100%), followed by silica gel column chromatography (φ30 x 235 mm) using CHCl3:MeOH (1:0 to 0:1) yielded ten fractions (11A-11J). Fraction 11H was identified as compound 7 (14.8 mg). Fraction 11E, which showed weak activity, was further purified using by preparative HPLC [Cosmosil π-NAP; φ10 x 250 mm; 60% MeOH] to give a mixture (6.8 mg) of compound 2 and an unknown compound. Another active fraction, 1I (203.2 mg), was subjected to ODS flash column chromatography (φ40 x 170 mm) and eluted with increasing MeOH (60%-80%) to give 11 fractions (15A-15K). Fraction 15F (18.3 mg), which showed strong activity, was subjected to silica gel column chromatography using CHCl3:MeOH (10:1-0:1) and yielded 8 fractions (16A-16H). Fraction 16D (7.1 mg) was further purified using Sephadex LH-20, followed by silica gel column chromatography [φ25 x 150 mm; CHCl3:MeOH (1:0-0:1)] to afford compound 8 (0.7 mg).
Compound 1. Colorless solid; [α]26D -9.9 (c 0.5 MeOH); positive HRESIMS [M+Na]+ m/z 381.1634 (calcd. for C21H26O5Na 381.1678, Δ -4.4 mmu); UV λmax (MeOH) nm (log ε) 278 (3.6) and 223 (4.2) IR νmax 3354, 2924, 1706, 1514, and 1242; 1H NMR (CD3OD, 600 MHz) and 13C NMR (CD3OD, 150 MHz), see Table 1.
Deacetylation of 1.12 A drop of conc. HCl was added to a MeOH (0.5 mL) solution of 1 (1.4 mg, 3.9 µmol) . The mixture was stirred at room temperature overnight, vacuum dried, and purified by silica gel chromatography using CHCl3:MeOH (9:1) to yield 7 (0.5 mg, 1.6 µmol, 42% yield). The optical rotation of 7 was determined through its response (negative) in HPLC with an optical rotation detector (ORD).
Compound 2. Colorless solid; positive HRESIMS [M+Na]+ m/z 399.1762 (calcd. for C21H26O5Na 399.1784, Δ -2.1 mmu; 1H NMR (CDCl3, 600 MHz) and 13C NMR (CDCl3, 150 MHz), see Table 1.
Acetonidation of 2. The acetonide derivative of 2 was prepared using the procedure presented by Rychnovsky et al.15 with minor modifications. 2,2-Dimethoxypropane (1 mL) was added to a solution of 2 (1.0 mg, 2.7 μmol) and pyridinium/p-toluenesulfonate (2.3 mg, 9.2 μmol) in dehydrated acetone (0.5 mL). The reaction mixture was stirred at room temperature for 1 h and dried in a stream of N2 vapor. The residue was subjected to silica gel chromatography using CHCl3:MeOH (95:5) as the eluent and afforded 2a (0.6 mg, 1.4 µmol, 55% yield). 1H NMR (600 MHz, C6D6): δ 0.93 (1H, dt, J = 1.2, 12.6 Hz, H-4), δ 0.99 (1H, dt, J = 1.2, 12.6 Hz, H-4), δ 1.32 (3H, s, CH3), δ 1.55 (2H, m, H-2 and 6), δ 1.62 (3H, s, CH3), δ 1.84 (2H, m, H-2 and 6), δ 2.66 (2H, m, H-1 and 7), δ 2.76 (2H, m, H-1 and 7), δ 3.37 (6H, m, 3’-OCH3 and 5’-OCH3), δ 6.37 (2H, s, H-2’ and 6’), δ 6.52 (2H, d, J = 8.7, H-3 and 5), δ 6.95 (2H, d, J = 8.7, H-2” and 6”). HRESIMS m/z 439.2070 [M+Na]+ (calcd. for C24H32O6Na 439.2097 Δ -2.7 mmu).
Statistical Analysis. Data for the inhibitory activities and cell viability are presented as the mean ± SEM of the three replicates of one representative experiment from two independent experiments. One way analysis of variance (ANOVA) followed by Tukey’s test. Probability (p) values less than 0.05 were considered significant.
ACKNOWLEDGEMENTS
We thank Prof. J. Nathans (John Hopkins University School of Medicine) for the STF/293 cells and Prof. R. T. Moon (University of Washington) for the SuperFOPFlash plasmid. This study was supported by Grants-in-aid for Scientific Research from the Japan Society for the Promotion of Science (JSPS), the Cosmetology Research Foundation, and Sekisui Chemical Innovations Inspired by Nature Research Support Program.
References
1. C. Y. Logan and R. Nusse, Annu. Rev. Cell Dev. Biol., 2004, 20, 781. CrossRef
2. J. Behrens, J. P. von Kries, M. Kuhl, L. Bruhn, D. Wedlich, R. Grosschedl, and W. Birchmeier, Nature, 1996, 382, 638. CrossRef
3. P. Polakis, Curr. Opin. Genetics Dev., 2007, 17, 45. CrossRef
4. H. Clevers and R. Nusse, Cell, 2012, 149, 1192. CrossRef
5. X. Li, T. Ohtsuki, T. Koyano, T. Kowithayakorn, and M. Ishibashi, Chem. Asian J., 2009, 4, 540. CrossRef
6. N. Mori, K. Toume, M. A. Arai, T. Koyano, T. Kowithayakorn, and M. Ishibashi, J. Nat. Med., 2011, 65, 234. CrossRef
7. H. Park, K. Toume, M. A. Arai, T. Koyano, T. Kowithayakorn, and M. Ishibashi, J. Nat. Med., in press.
8. A. Suksamrarn, M. Ponglikitmongkol, K. Wongkrajang, A. Chindaduang, S. Kittidanairak, A. Jankam, B. Yingyongnarongkul, N. Kittipanumat, R. Chokchaisiri, P. Khetkam, and P. Piyachaturawat, Bioorg. Med. Chem., 2008, 16, 6891. CrossRef
9. Y. Qu, F. Xu, S. Nakamura, H. Matsuda, Y. Pongpiriyadacha, L. Wu, and M. Yoshikawa, J. Nat. Med., 2009, 63, 102. CrossRef
10. W. Winuthayanon, K. Suksen, C. Boonchird, A. Chuncharunee, M. Ponglikitmongkol, A. Suksamrarn, and P. Piyachaturawat, J. Agric. Food Chem., 2009, 57, 840. CrossRef
11. A. Sodsai, P. Piyachaturawat, S. Sophasan, A. Suksamrarn, and M. Vongsakul, Int. Immunopharmacol., 2007, 7, 524. CrossRef
12. J. Li, C.-R. Liao, J.-Q. Wei, L.-Z. Chen, F. Zhao, and F. Qiu, Bioorg. Med. Chem. Lett., 2011, 21, 5363. CrossRef
13. L. V. Alegrio, R. Braz-filho, and O. R. Gottlieb, Phytochemistry, 1989, 28, 2359. CrossRef
14. A. Yokosuka, Y. Mimaki, H. Sakagami, and Y. Sashida, J. Nat. Prod., 2002, 65, 283. CrossRef
15. S. D. Rychnovsky, T. I. Richardson, and B. N. Rogers, J. Org. Chem., 1997, 62, 2925. CrossRef
16. D. Shin, K. Kinoshita, K. Koyama, and K. Takahashi, J. Nat. Prod., 2002, 65, 1315. CrossRef
17. H. Kikuzaki, M. Kobayashi, and N. Nakatani, Phytochemistry, 1991, 30, 3647. CrossRef
18. S. Ohta, M. Koyama, T. Aoki, and T. Suga, Bull. Chem. Soc. Jpn, 1985, 58, 2423. CrossRef
19. P. M. Giang, P. T. Son, K. Matsunami, and H. Otsuka, Nat. Prod. Res., 2011, 26, 1195. CrossRef
20. M. S. Ali, Y. Tezuka, S. Awale, A. H. Banskota, and S. Kadota, J. Nat. Prod., 2001, 64, 289. CrossRef
21. H. Shibuya, Y. Hamamoto, Y. Cai, and I. Kitagawa, Chem. Pharm. Bull., 1987, 35, 924. CrossRef
22. T. Masuda, A. Jitoe, and N. Nakatani, Chem. Lett., 1991, 20, 1625. CrossRef
23. D. Hu, N. Ma, Y. Lou, G.-X. Qu, and F. Qiu, Shenyang Yao Ke Da Xue Xue Bao, 2008, 25, 188.
24. C. H. Park, J. Y. Chang, E. R. Hahm, S. Park, H.-K. Kim, and C. H. Yang, Biochem. Biophys. Res. Commun., 2005, 328, 227. CrossRef
25. M.-J. Ryu, M. Cho, J.-Y. Song, Y.-S. Yun, I.-W. Choi, D.-E. Kim, B.-S. Park, and S. Oh, Biochem. Biophys. Res. Commun., 2008, 377, 1304. CrossRef
26. B. Chen, M. E. Dodge, W. Tang, J. Lu, Z. Ma, C.-W. Fan, S. Wei, W. Hao, J. Kilgore, N. S. Williams, M. G. Roth, J. F. Amatruda, C. Chen, and L. Lum, Nat. Chem. Biol., 2009, 5, 100. CrossRef
27. E. Lindhagen, P. Nygren, and R. Larsson, Nat. Protoc., 2008, 3, 1364. CrossRef