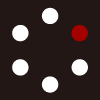
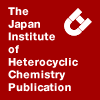
HETEROCYCLES
An International Journal for Reviews and Communications in Heterocyclic ChemistryWeb Edition ISSN: 1881-0942
Published online by The Japan Institute of Heterocyclic Chemistry
e-Journal
Full Text HTML
Received, 25th July, 2013, Accepted, 14th August, 2013, Published online, 16th August, 2013.
DOI: 10.3987/COM-13-S(S)93
■ Chiral Memory Units Based on Dynamic Redox Systems with a Dibenzoxepinone Skeleton: Drastic Change in Racemization Barrier and Electrochemical Bistability
Kazuhisa Wada, Yuna Chiba, Takashi Takeda, Hidetoshi Kawai, Ryo Katoono, Kenshu Fujiwara, and Takanori Suzuki*
Division of Chemistry, Graduate School of Science, Hokkaido University, Kita 10 Nishi 8, Kita-ku, Sapporo, Hokkaido 060-0810, Japan
Abstract
The configuration of helical dicationic dyes 22+ with a dihydrodibenzoxepinone unit is stable, whereas the corresponding neutral electron donors 1 with a tetrahydrophenanthroxepinone skeleton easily undergo ring-flip. Thanks to their electrochemical bistability, electron exchange between 1 and 22+ is prohibited. Thus, the above electrochromic pairs 1/22+ can serve as new members of less well-explored redox-based chiral-memory units.The intermolecular transmission of asymmetric information is the very important event in the field of supramolecular chirality.1 Molecules that act as chiral-memory units play important roles in these phenomenon.2 To ensure this function, the chiral unit should be able to adopt an easily-racemized state ("erasable/writable"-state), but must also be intentionally interconvertible with the non-racemized state ("memorizing"-state) under certain conditions. Switching by heating/cooling is successfully realized in the folded polymer chains with a helical structure3 and some macrocyclic molecules.4 However, much less has been reported on compounds whose interstate-switching5 is achieved by electron transfer ("chiral redox memory") except our recent report based on the "dynamic redox pair"6 of phenanthr[4,5-cde]oxepin-type donor 3a and dibenz[c,e]oxepin-type dication 4a2+.7
During our further studies toward the chiral memory units, we envisaged that more easily-accessible lactone analogues (1/22+) can serve as promising new entries (Scheme 1). Thus, the ring inversion of 4,6,10,11-tetrahydrophenanthr[4,5-cde]oxepin-4-one in 1 would occur easily at room temperature (the "erasable/writable"-state) whereas the ring flip of 5,7-dihydrodibenz[c,e]oxepin-5-one skeleton attached with two cationic chromophores at 1,11-positions in 22+ would be prohibited (the "memorizing"-state). As redox active chromophores, not only spiroacridan/acridinium (a) but also spiroxanthene/xanthenylium (b) were chosen for tuning the electric potentials at which the switching occurs (Table 1).8 Here we report redox properties, configurational stability and electrochromic behavior of newly prepared lactone-based redox pairs (1a,b/2a,b2+), that can be applicable for realizing chiral redox memories.
Sterically congested hexaphenylethane-type molecule 1a was planned to prepare via the corresponding bond-dissociated dication 2a2+, and we first pursue generation of diacridinium 2a2+. Thus, 9,9'-(6,6'-diformylbiphenyl-2,2'-diyl)diacridine (7) was derived from 2,2',6,6'-tetrabromobiphenyl over 3 steps in 52% yield as we reported previously.9 Upon treating 7 with K2CO3 in MeOH, the Tishchenko reaction took place very smoothly10 to give lactone 811 in 70% yield. According to the X-ray analysis,12 the 5,7-dihydrodibenz[c,e]oxepin-5-one skeleton in 8 adopts a deeply twisted geometry as expected, and the torsion angle of C1-C11b-C11a-C11 is as large as 52.4(8)º (Figure 1). When diacridine 8 was treated with MeOTf (25 equiv.) in CH2Cl2 in the presence of 2,6-di-t-butyl-4-methylpyridine (DTBMP) at room temperature, 2a2+(TfO-)2 was obtained as an orange-yellow solid containing a small amount of DTBMP-H+ TfO-, which was directly treated with Zn dust in THF to give desired 1a11 in 49% yield over two steps (Scheme 2). In the preparation of spiroxanthene 1b, the Tishchenko Reaction was again featured as a key step. Thus, 4',5'-dibromodispiro[xanthene-9,9'(9'H,10'H)-phenanthrene-10',9''-xanthene] 913 was converted to the corresponding dialdehyde 1014 as we reported previously, and then 10 was treated with K2CO3 in MeOH to give desired 1b11 in 33% yield (Scheme 3).
According to the voltammetric analyses in MeCN, 1a undergoes 2e-oxidation at Eox of +0.27 V (irrev.) vs SCE (Figure 2). Under the similar conditions, Eox of 1b is +1.08 V (irrev.), thus we can modify the electron donating ability of 1 by changing the heteroatom in the tricyclic spiro-skeletons as designed. In each case, the reduction peak appeared in the far cathodic region, which corresponds to the reduction process of bond-dissociated dication 2a2+(Ered = −0.07 V; irrev.) or 2b2+(+0.52 V; irrev.). Such a shift in redox peaks is characteristic to the dynamic redox pair undergoing drastic structural changes upon electron transfer.6 Thanks to the electrochemical bistability, there would be no chance of electron-exchange between 1 and 22+, which is one of the most important features in utilizing them as the memory units.
Despite the microscopic irreversibility of electrochemical processes, the voltammograms did not change after many scans, showing that the total redox processes involving interconversion between 1 and 22+ are reversible. In fact, upon treatment of 1a with two equiv. of one-electron oxidant [(4-BrC6H4)3N+●SbCl6-], 2a2+(SbCl6-)211 was isolated as a stable yellow solid in 85% yield. This is also the case of less-donating 1b, and thus 2b2+(SbCl6-)211 was obtained in 70% isolated yield. Upon treatment with Zn dust in THF, these salts regenerated neutral donors 1a and 1b in respective yields of 90 and 91%. Based on the reversible interconversion, the redox pairs of 1a,b/2a,b2+ can serve as novel electrochromic systems. Thus, upon constant-current electrochemical oxidation, a colorless MeCN solution of 1a turned into yellow, and a continuous change of UV-Vis spectrum into that of 2a2+ was observed (Figure 3a). Presence of several isosbestic points indicated clean conversion as well as negligible steady-state concentration of intermediary cation-radical species. The electrolysis of colorless 1b to orange 2b2+ was also followed by UV-Vis spectrum (Figure 3b). It is evident that the absorption bands of the dications 22+ differ depending on the kind of heteroatom in the cationic chromophores.
Finally, the redox control of configurational stability was studied by VT-NMR. The methylene protons of the dihydroxepinone skeleton in spiroacridan 1a in C6D6 appeared as the double doublets at 10 ºC, which gradually coalesced (Tc = 45 ºC) and became a sharp singlet at 70 ºC (Figure 4a). Based on the results, the energy barrier of the ring flip in 1a was calculated to be 16 kcal mol-1, which means easy interconversion of enantiomers at room temperature ("erasable/writable"-state). In the case of spiroxanthene 1b, the same ΔG‡ value (16 kcal mol-1 in CDCl3, Tc = 40 ºC) was determined. These ΔG‡ values are slightly larger than that for the structurally related dihydroxepin 3a (12 kcal mol-1)7 without a carbonyl group in the seven-membered ring, showing that the lactone unit slightly changes the barrier. However, more drastic changes would be observed upon oxidation of 1a,b. In fact, the 1H NMR spectra of dications 2a,b2+ showed no temperature dependence (Figure 4b), and thus the lower limit of energy barrier of 2a2+ was estimated as 21 kcal mol-1 from Tc > 150 ºC in DMSO-d6. Thus, it is highly probable that the configuration of 2a,b2+ is stable enough for their use as "memorizing"-states.
To get more clue to the configurational stability of dications 22+, optical resolution of diacridine 8 was conducted, because this precursor for the dication 2a2+ would have the similar energy barrier to that of 2a2+. Separation by using chiral HPLC (DAICEL CHIRALPAK IA) was found effective. Although the second fraction was contaminated by the antipode due to partial peak overlap, the first fraction [(M)-8] was isolated as optically pure form. The CD spectrum shown in Figure 5 did not change for a long time without any loss of CD intensity, showing that the energy barrier is quite high (ΔG‡ > 25 kcal mol-1).
In summary, we designed polycyclic lactone-based redox pairs 1a,b/2a,b2+ having spiroacridan/acridinium and spiroxanthene/xanthenylium chromophores as new entries into less well-developed category of redox-based chiral memory units. They were prepared by using the Tishchenko reaction as a key step. The electrochemical bistability characteristic to the dynamic redox systems was confirmed also in 1a,b/2a,b2+ by the large shift of redox peaks in the voltammograms. Despite microscopic irreversibility, the redox cycles between 1a,b are 2a,b2+ are reversible as demonstrated by good isolated-yield interconversion as well as electrochromic behavior exhibiting isosbestic points. The drastic change in racemization barrier of 1a,b/2a,b2+ was indicated based on the VT-NMR analyses. In this way, we now conclude that the newly prepared redox pairs 1a,b/2a,b2+ are promising candidates for feature use of chiral redox memory units.
ACKNOWLEDGEMENTS
This work was supported by the Grant-in-Aid for Scientific Research on Innovative Areas: "Organic Synthesis Based on Reaction Integration" and by the Grant-in-Aid for Challenging Exploratory Research on "Maximum Function on Minimum Skeleton (MFMS)" (No. 25620050) from MEXT, Japan. K.W. thanks JSPS research fellowship for young scientists (23-4640). This work was also supported by the Cooperative Research Program of “Network Joint Research Center for Materials and Devices”. We are grateful to Prof. Takanori Fukushima at Chemical Resources Laboratory, Tokyo Institute of Technology.
References
1. R. Purrello, Nat. Mater., 2003, 2, 216; CrossRef A. R. A. Palmans and E. W. Meijer, Angew. Chem. Int. Ed., 2007, 46, 8948; CrossRef G. A. Hembury, V. V. Borovkov, and Y. Inoue, Chem. Rev., 2008, 108, 1. CrossRef
2. T. Ishi-i, M. Crego-Calama, P. Timmerman, D. R. Reinhoudt, and S. Shinkai, J. Am. Chem. Soc., 2002, 124, 14631; CrossRef R. D. Rasberry, X. Wu, B. N. Bullock, M. D. Smith, and K. D. Shimizu, Org. Lett., 2009, 11, 2599. CrossRef
3. E. Yashima, K. Maeda, and Y. Okamoto, Nature, 1999, 399, 449; CrossRef M. Ishikawa, K. Maeda, Y. Mitsutsuji, and E. Yashima, J. Am. Chem. Soc., 2004, 126, 732; CrossRef N. Ousaka, Y. Inai, and R. Kuroda, J. Am. Chem. Soc., 2008, 130, 12266; CrossRef T. Miyabe, Y. Hase, H. Iida, K. Maeda, and E. Yashima, Chirality, 2009, 21, 44. CrossRef
4. Y. Mizuno, T. Aida, and K. Yamaguchi, J. Am. Chem. Soc., 2000, 122, 5278; CrossRef R. Katoono, H. Kawai, K. Fujiwara, and T. Suzuki, Chem. Commun., 2005, 5154; CrossRef J. Setsune, A. Tsukajima, N. Okazaki, J. M. Lintuluoto, and M. Lintuluoto, Angew. Chem. Int. Ed., 2009, 48, 771; CrossRef R. Randazzo, A. Mammana, A. D’Urso, R. Lauceri, and R. Purrello, Angew. Chem. Int. Ed., 2008, 47, 9879; CrossRef R. Randazzo, A. Mammana, A. D’Urso, R. Lauceri, and R. Purrello, Angew. Chem. Int. Ed., 2009, 48, 1351. CrossRef
5. J. W. Canary, Chem. Soc. Rev., 2009, 38, 747; CrossRef H. Goto and E. Yashima, J. Am. Chem. Soc., 2002, 124, 7943. CrossRef
6. T. Suzuki, E. Ohta, H. Kawai, K. Fujiwara, and T. Fukushima, Synlett, 2007, 851; CrossRef T. Suzuki, H. Higuchi, T. Tsuji, J. Nishida, Y. Yamashita, and T. Miyashi, Chemistry of Nanomolecular Systems. Chapter 1: Dynamic Redox Systems, Springer, Heidelberg, 2003, pp. 3–24; T. Suzuki, J. Nishida, and T. Tsuji, Angew. Chem., Int. Ed. Engl., 1997, 36, 1329; CrossRef . CrossRef
7. T. Suzuki, K. Wada, Y. Ishigaki, Y. Yoshimoto, E. Ohta, H. Kawai, and K. Fujiwara, Chem. Commun., 2010, 46, 4100. CrossRef
8. T. Suzuki, J. Nishida, and T. Tsuji, Chem. Commun., 1998, 2193. CrossRef
9. T. Suzuki, Y. Yoshimoto, K. Wada, T. Takeda, H. Kawai, and K. Fujiwara, Heterocycles, 2010, 80, 149. CrossRef
10. T. Seki, T. Nakajo, and M. Onaka, Chem. Lett., 2006, 35, 824; CrossRef Typical procedure: A suspension of dialdehyde 7 (150 mg, 266 μmol) and K2CO3 (220 mg, 1.59 mmol) in MeOH 6 mL was stirred at 27 ˚C for 15.5 h. After diluted with water, the whole mixture was extracted with CHCl3. The combined organic layers were washed with water and brine, and dried over anhydrous Na2SO4. After filtration, the crude reaction mixture was concentrated under reduced pressure. The residue was purified by recrystallization from EtOH to give lactone 8 (64.7 mg) as a yellow solid. Mother liquor was purified by flash chlomatography on silica gel (CHCl3 : EtOAc = 1 : 1, 0.5% v/v Et3N) to give 8 (40.3 mg) as a yellow solid in a total yield of 70%.
11. Data for 1a: 1H NMR (300 MHz, CDCl3) δ 7.90 (1H, dd, 1.8 Hz, 7.1 Hz) 7.44 (1H, dd, J =1.8 Hz, 6.6 Hz), 7.19-7.35 (4H, m), 6.99 (4H, ddd, J = 1.8 Hz, 8.3 Hz, 8.3 Hz), 6.79 (1 H, d, J = 7.9 Hz), 6.38-6.64 (7H, m), 6.20 (2H, br-s), 5.77 (1H, d, 7.3 Hz), 5.71 (1H, d, J = 7.2 Hz), 5.39 (1H, d, J = 12.5 Hz), 5.22 (1H, d, J = 12.5 Hz), 2.73 (3H, s), 2.72 (3H, s); IR (KBr) υ cm-1 3069, 2961, 2871, 2820, 1720, 1591, 1475, 1427,1383, 1363, 1324, 1280, 1245, 1169, 1135, 1101, 1059, 1039, 896, 863, 793, 750, 699, 657; LR-MS (EI) m/z 594 (M+, BP), 579 ([M-CH3]+, 98), 535 ([M-CH3-CO2]+, 9.7), 446 (11), 368 (12), 297 (M2+, 21), 278 (74), 185 (13), 171 (11), 157 (16), 129 (29), 97 (24), 83 (28), 57 (51).
Data for 1b: 1H NMR (300 MHz, CDCl3) δ 7.99 (1H, ddd, J = 1.8 Hz, 4.4 Hz, 9.0 Hz), 7.54 (1H, td, J = 1.4 Hz, 7.2 Hz), 7.33-7.37 (3H, m), 7.22-7.28 (2H, m), 7.19 (2H, br-s), 7.04 (2H, br-s), 6.82 (2H, br-s), 6.71 (1H, br-s), 6.61 (4H, br-s), 6.46 (2H, br-s), 5.72 (2H, br-s), 5.42 (1H, br-d, J = 9.5 Hz), 5.27 (1H, br-d, J = 9.5 Hz); IR (KBr) υ cm-1 3066, 2923, 2855, 1713, 1598, 1571, 1477, 1442, 1374, 1309, 1281, 1241, 1132, 1100, 1038, 900, 867, 775, 751, 707, 651; LR-MS (EI) m/z = 568 (M+, bp).
Data for 2a2+(SbCl6-)2: 1H NMR (300 Hz, CD3CN) δ 8.46 (2H, dd, J = 7.9 Hz, 8.8 Hz) 8.29 (1H, dd, J = 1.5 Hz, 6.4 Hz, 9.3 Hz), 8.26 (1H, dd, J = 1.5 Hz, 6.8 Hz), 8.07-8.14 (1H, m), 8.08 (2H, dd, J = 1.5 Hz, 7.9 Hz), 8.02 (1H, br-d, J = 8.8 Hz), 8.00 (2H, dd, J = 1.1 Hz, 7.9 Hz), 7.63-7.72 (1H, m), 7.68 (2H, dd, J = 7.9 Hz, 7.9 Hz), 7.59 (2H, dd, J = 7.9 Hz, 7.9 Hz), 7.54 (1H, dd, J = 1.1 Hz, 8.6 Hz), 7.32 (1H, ddd, J = 1.1 Hz, 6.4 Hz, 8.8 Hz), 7.27 (1H, ddd, J = 1.1 Hz, 6.4 Hz, 8.6 Hz), 7.13 (1H, dd, J = 1.5 Hz, 7.9 Hz), 7.06 (1H, dd, J = 1.5 Hz, 7.9 Hz), 6.41 (1H, dd, J = 1.1 Hz, 8.6 Hz), 6.29 (1H, br-d, J = 8.8 Hz), 5.66 (1H, d, J = 12.6 Hz), 5.50 (1H, d, J = 12.6 Hz), 4.54 (3H, s), 4.52 (3H, s). IR (KBr) ν cm-1 3083, 2957, 1727, 1608, 1578, 1548, 1460, 1445, 1370, 1278, 1192, 1099, 1026, 1015, 765, 707, 653, 602.
Data for 2b2+(SbCl6-)2: 1H NMR (300 MHz, CD3CN) δ 8.54 (1H, d, J = 7.2 Hz), 8.49 (1H, d, J = 7.2 Hz), 8.36 (2H, dd, J = 3.9 Hz, 7.8 Hz), 8.27 (1H, d, J = 3.9 Hz), 8.23 (2H, dd, J = 2.4 Hz, 6.6 Hz), 8.14 (1H, d, J = 7.5 Hz), 7.79-7.87 (4H, m), 7.70-7.77 (2H, m), 7.54-7.64 (4H, m), 7.29 (1H, d, J = 7.9 Hz), 7.23 (1H, d, J = 7.9 Hz), 6.80 (1H, d, J = 8.6 Hz), 6.70 (1H, d, J = 8.6 Hz), 5.74 (1H, d, J = 12.8 Hz), 5.57 (1H, d, J = 12.8 Hz); IR (KBr) ν cm-1 3075, 2954, 2925, 2855, 1728, 1621, 1598, 1577, 1537, 1502, 1367, 751.
Data for 8: 1H NMR (300 MHz, CDCl3) δ 8.08 (1H, d, J = 8.8 Hz), 8.03 (1H, d, J = 8.8 Hz), 7.95 (1H, dd, J = 1.5 Hz, 7.9 Hz), 7.69 (1H, dd, J = 1.3 Hz, 7.9 Hz), 7.61 (2H, br-d, J = 7.9 Hz), 7.57 (1H, br-d, J = 6.0 Hz), 7.41 (2H, dd, J = 7.9 Hz, 7.9 Hz), 7.40 (1H, dd, J = 1.1 Hz, 7.9 Hz), 7.29-7.37 (3H, m), 7.23 (2H, d, J = 10.3 Hz), 7.14 (1H, ddd, J = 0.9 Hz, 6.4 Hz, 8.8 Hz), 7.03 (1H, dd, J = 1.5 Hz, 7.9 Hz), 6.98 (1H, dd, J = 1.3 Hz, 7.9 Hz), 6.70-6.77 (2H, m), 5.79 (1H, d, J = 8.8 Hz), 5.68 (1H, d, J = 8.4 Hz), 5.57 (1H, d, J = 11.7 Hz), 5.36 (1H, d, J = 11.7 Hz); IR (KBr) υ cm-1 3063, 1728, 1626, 1608, 1554, 1538, 1517, 1460, 1436, 1407, 1370, 1353, 1263, 1227, 1083, 1013, 856, 818, 795, 751, 690, 649, 639, 629, 618, 612, 603 cm-1; LR-MS (FD) m/z 564 (M+, BP).
12. Crystal data of 8•CHCl3: C41H25N2O2Cl3, M 564.63, triclinic P-1, a = 9.506(10) Å, b = 12.48(2) Å, c = 14.54 (2) Å, α = 109.84(2) ˚, β = 93.32 (2) ˚, γ = 98.47(2) ˚, V = 1594.1(2) Å3, Z = 2, Dc = 1.42 gcm-3, independent reflection 6844 (all), 4304 (2σ), T = 153 K, R = 9.7%, CCDC 951650.
13. T. Suzuki, R. Yamamoto, H. Higuchi, E. Hirota, M. Ohkita, and T. Tsuji, J. Chem. Soc., Perkin Trans. 2, 2002, 1937.
14. T. Suzuki, S. Tanaka, H. Higuchi, H. Kawai, and K. Fujiwara, Tetrahedron Lett., 2004, 45, 8563. CrossRef