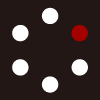
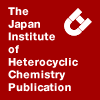
HETEROCYCLES
An International Journal for Reviews and Communications in Heterocyclic ChemistryWeb Edition ISSN: 1881-0942
Published online by The Japan Institute of Heterocyclic Chemistry
e-Journal
Full Text HTML
Received, 27th June, 2013, Accepted, 19th July, 2013, Published online, 29th July, 2013.
DOI: 10.3987/COM-13-S(S)53
■ Study on Hinsberg Thiophene Synthesis of 4,8-Dihydro-4,8-ethanobenzo[1,2-c;4,5-c’]dithiophene
Kazunari Tagawa, Shigeki Mori, Takahiro Nakae, Tetsuo Okujima, and Hidemitsu Uno*
Department of Chemistry and Biology, Graduate School or Science and Engineering, Ehime University, Bunkyo-cho 2-5, Matsuyama 790-8577, Japan
Abstract
The Hinsberg thiophene synthesis using 4,5,6,7-tetrahydro-4,7-ethanothiophene-5,6-dione and diethyl thiodiglycolate afforded a mixture of diethyl 4,8-dihydro-4,8-ethanobenzo[1,2-c;4,5-c’]dithiophene-1,3-dicarboxylate and its dihydrate, although the yields were low. The latter dihydrate was converted to the former dithiophene in a good yield by the treatment with Ac2O and DMAP.INTRODUCTION
A 1,3-diradicaloid system represented by trimethylene methane1 and 1,3-xylylene2 has continuously attracted not only theoretical interest but also practical interest as a candidate for organic light-emitting materials3 and electrode active materials.4 This system must have degenerated or nearly degenerated molecular orbitals, where two electrons exist. Therefore, this system tends to be a diradical with an open-shell electronic state. The energy difference between the ground and the first excited states are quite small. Contrarily to the 1,3-xylylene system, 1,2- and 1,4-xylylenes (o- and p-quinodimethane)5 have a closed-shell, although the compounds are still reactive. When the benzene skeleton of 1,2-xylylene is replaced by the β positions of 5-membered aromatic such as furan, pyrrole, or thiophene, however, the system becomes a non-Kekulé structure of 3,4-dimethylene-furan, pyrrole, or thiophene (1, Figure 1).6 This system was reported to be a singlet biradical system and a useful reactive component for the construction of polycyclic derivatives by the Diels-Alder reaction.7 We have engaged in the preparation of new highly π-conjugated compounds by applying organic synthesis using retro-Diels-Alder reaction of precursor molecules for fusion of stable and easily accessible π systems,8 and showed the usefulness of 4,8-dihydro-4,8-ethanopyrrol[3,4-f]isoindole 2aa, namely bicyclo[2.2.2]octadiene-fused (BCOD-fused) dipyrrole, which was thought to be masked pyrrol[3,4-f]isoindole 3a with the non-Kekulé structure. The BCOD-fused dipyrrole itself did not undergo the selective retro-Diels-Alder reaction giving pyrrol[3,4-f]isoindole,9 although the BCOD-fused dipyrrole moiety was successfully converted to formal pyrrol[3,4-f]isoindole skeleton if it was involved in a large π system.10 Even if the retro-Diels-Alder reaction did not take place selectively due to the high heat formation of the aimed compound,11 photochemical cheletropic decomposition reactions of 7,8-dioxobicyclo[2.2.2]octadiene12 and 7-oxobicyclo[2.2.1]heptadiene13 skeletons have been shown to be a powerful tool for giving the aimed highly conjugated aromatic compounds. We planned to investigate the nature of β,β,β’,β’-benzene-fused dipyrrole 3a and dithiophene 3b generated by the cheletropic decomposition of precursors 2ab, 2ac, 2bb, and 2bc. Before we start to prepare the dithiophene precursor, we have to solve the problem on the construction of another thiophene ring at the BCOD double bond, because we had encountered the difficulty in the preparation of BCOD-fused thiophenes under the Hinsberg conditions (Scheme 1). In the preparation of 4,7-dihydro-4,7-ethano-2-benzothiophene (7), we first tried to prepare dicarboxylate derivative 6 by the Hinsberg reaction using thiodiglycolate 5 and bicyclo[2.2.2]oct-4-ene-2,3-dione (4),14 which was prepared by the Diels-Alder reaction of 1,3-cyclohexadiene and ethylene carbonate followed by saponification and oxidation. Generally, the Hinsberg thiophene synthesis was thought to be successfully applicable for the preparation using non-enolizable α-dicarbonyl compounds such as benzil, oxalate diester, glyoxal, and glyoxylate ester.15 Dione 4 is a non-enolizable α-diketone due to the Bredt’s rule. In spite of all of our efforts, however, the aimed thiophene was obtained only in a trace amount. Therefore, we prepared BCOD-fused thiophene 7 from thiolactone 8, which was obtained by the Diels-Alder reaction of 1,3-cyclohexadiene and 2-thiophenone.16 In this paper, we would like to discuss about our effort on the Hinsberg reaction giving BCOD-fused dithiophenes.
RESULTS AND DISCUSSION
We focused our attention on the construction of another thiophene ring at the double bond of BCOD-fused thiophene 7 by the Hinsberg reaction. First, dihydroxylation of the double bond was carried out. Treatment of 7 with a catalytic amount of OsO4 and N-methylmorpholine N-oxide (NMO) in aqueous acetone at room temperature gave a diastereomeric mixture of diol 9 in low yields. Microencapsulated OsO417 gave the best result: exo-9 and endo-9 were obtained in respective yields of 11% and 81%. Oxidation of diol 9 under Swern conditions afforded α-diketone 10 (68%) as a pale yellow oil, which solidified after crystallization with methanol.
Next, the Hinsberg thiophene synthesis using 10 was examined and the results are summarized in Table 1. Diketone 10 was reacted with diethyl thiodiglycolate (5) under the basic conditions of KO-t-Bu in dry THF at various temperatures. However, the aimed product was not obtained at all, although the similar reaction of dimethyl thiodiglycolate with bicyclo[2.2.2]octa-2,3-dione was reported to proceed in a 37% yield.18 As partial saponification was sometimes reported in the Hinsberg reaction,19 the reaction mixture was treated with trimethylsilyldiazomethane (TMSCHN2). The corresponding dimethyl ester of BCOD-fused dithiophene 11b was obtained, although the yield was very low (3%, entry 1). In order to improve the yield, we checked and purified all of the materials used in the reaction. We were using α-diketone 10 after recrystallization from methanol. During this recrystallization, colorless crystals had been obtained from the pale yellow oil of 10. Fortunately, this crystals were suitable for X-ray analysis (vide infra), which revealed that the structure was not α-diketone 10 but its mono-hydrate 10’ (Eq 1). Therefore, we heated the crystals of 10 prior to use until the color changed to yellow. By using the yellow material, the reaction with 5 at 45 °C provided two thiophene fractions after chromatography. The material from the less polar fraction was the aimed BCOD-fused dithiophene 11a (5%) and that from the polar fraction was a simple adduct endo-12 (11%), structure of which was unambiguously determined by X-ray analysis (vide infra). The reaction giving endo-12 occurred from the endo-face of α-diketone 10 and exo-face of thiodiglycolate 5. The reaction at room temperature only gave one thiophene compound, which was proved to be another isomer of adduct 12 (11%). The X-ray analysis revealed the structure as exo-12 (vide infra). The adduct exo-12 was converted to BCOD-fused dithiophene 11a in a 68% yield under acetylation conditions. From the thermogravimetric analysis (10 °C/min) of 11a and 12, no selective fragmentation of ethylene or water was observed below the boiling points (> 200 °C).
X-Ray analysis of the products was examined. Single crystals of exo-9, endo-9·1/2dioxane, 10’, 11a, endo-12, and exo-12 were obtained. The Ortep drawings are shown in Figure 2. In the case of 11a, two crystallogaphically independent molecules are found and their structures are similar each other except for the thiophene plane angle. The angle in one molecule is 130.7°, while that in another is 123.8°. Both ester carbonyl groups in both molecules of 11 are directed toward the opposite direction of the thiophene sulfur atom, although the ester carbonyl groups of pyrrole-2-carboxylate derivatives are usually directed toward the nitrogen atom.9
In conclusion, we succeeded in the preparation of BCOD-fused dithiophene based on the Hinsberg reaction, although the yield is unsatisfactory. Easy hydration of the bicyclo[2.2.2]oct-5-ene-2,3-dione skeleton was confirmed by X-ray analysis. Further study including the preparation of photo-compatible precursors is under way.
EXPERIMENTAL
General: Melting points were measured on a Yanagimoto micro-melting point apparatus and are uncorrected. NMR spectra were obtained with an AL-400 spectrometer at the ambient temperature by using CDCl3 as a solvent and tetramethylsilane as an internal standard for 1H and 13C. IR spectra were obtained by a Thermo Scientific Nicolet iS5 FT-IR spectrometer with an iD5 ATR diamond plate. Mass spectra (EI, 70 eV; FAB+, p-nitrobenzyl alcohol) were measured with a JEOL JMS-700. Elemental analyses were performed with a Yanaco MT-5 elemental analyzer at Integrated Center for Sciences. Dehydrated tetrahydrofuran and dichloromethane were purchased from Kanto Chemical Co. and used without further purification. Potassium tert-butoxide was sublimed at 200 °C under a reduced pressure (ca. 13 Pa) and dissolved in dry THF (1.0 mol·L−1). Pyridine was distilled from CaH2 under nitrogen and stored on molecular sieves 4A. Other commercially available materials were used without further purification.
X-Ray measurement: X-Ray measurements of the single crystals were done with Rigaku AFC8S Mercury CCD (1.5 kW Mo sealed tube) at room temperature or Rigaku VariMax Saturn-724 (1.2 kW Mo rotating anode) at −173 °C. Single crystals for X-ray analysis were obtained by the diffusion method. X-Ray diffraction data were processed by CrystalClear 1.6.3 followed by CrystalStructure Ver. 4.0.1.20 Structures were solved by using the processed data with SIR 2004,21 and then refined by Shelxl64 (Shelx-2013).22 Final structures were validated by the Platon CIF check.23
4,5,6,7-Tetrahydro-4,7-ethano-2-benzothiophene-5,6-diol (9): BCOD-fused thiophene 7 (690 mg, 4.25 mmol), N-methylmorpholine N-oxide mono-hydrate (597 mg, 5.10 mmol), and 10% microencapsulated OsO4 (1.05 g, ca. 0.41 mmol) in a mixture of acetone (20 mL) and water (1 mL) were stirred at 45 °C for 6 days. The mixture was filtered through a Celite pad, which was washed with acetone. The filtrate was concentrated and the residue was purified by column chromatography on silica gel (EtOAc/hexane = 3/2) to afford 94 mg (0.48 mmol, 11%) of exo-9 and 676 mg (3.44 mmol, 81%) of endo-9. exo-9: colorless crystals, mp 141-142 °C; IR νmax 3445, 3331, 2946, 1072, 780 cm−1; 1H NMR δ 1.23 (m, 2H), 2.15 (m, 2H), 2.88 (br s, 2H), 3.18 (br. s, 2H), 3.74 (br. s, 2H), 6.96 (s, 2H); 13C NMR δ 18.5, 38.3, 66.8, 116.6, 141.9. Crystal data: Formula: C10H12O2S, 0.35 × 0.30 × 0.20 mm, orthorhombic, space group Pbca, a = 10.562(3), b = 6.6132(19), c = 25.465(7) Å, V = 1778.6(9) Å3, T = 298 K, Z = 8, ρcalcd = 1.466 g·cm−3, μ = 0.323 mm−1, F(000) = 832. 6478 diffractions measured, 2036 unique, 1633 observed [I > 2σ(I)]; Requiv = 0.0253, R1 = 0.0466 [I > 2σ(I)], wR2 = 0.1197 (all); GOF = 1.099. CCDC No. 945208. endo-9: colorless crystals, mp 146-148 °C; IR νmax 3237, 3094, 2943, 1061, 793 cm−1; 1H NMR δ 1.46 (m, 2H), 1.71 (m, 2H), 2.24 (m, 2H), 3.22 (br s, 2H), 4.02 (m, 2H), 7.07(s, 2H); 13C NMR δ 23.1, 39.0, 70.1, 118.9, 139.1; MS (FAB+) m/z 197 (M++1). Anal. Calcd for C10H12O2S: C, 61.20; H, 6.16. Found: C, 60.98; H, 6.35%. Crystal data: Formula: C10H12O2S·1/2C4H8O2, 0.20 × 0.13 × 0.10 mm, triclinic, space group P−1, a = 8.346(3), b = 8.765(3), c = 9.383(3) Å, α = 69.77(2)°, β = 67.12(2)°, γ = 66.44(2)°, V = 564.8(4) Å3, T = 100 K, Z = 2, ρcalcd = 1.413 g·cm−3, μ = 0.275 mm−1, F(000) = 256. 7016 diffractions measured, 2585 unique, 2099 observed [I > 2σ(I)]; Requiv = 0.0372, R1 = 0.0320 [I > 2σ(I)], wR2 = 0.0780 (all); GOF = 1.059. CCDC No. 945205.
4,5,6,7-Tetrahydro-4,7-ethano-2-benzothiophene-5,6-dione (10): To a stirred solution of dimethyl sulfoxide (5.0 mL, 70 mmol) in CH2Cl2 (100 mL) at −60 °C was added trifluoroacetic anhydride (10 mL, 72 mmol) under argon atmosphere. After 10 min, diol 9 (920 mg, 4.69 mmol) in 10 mL of CH2Cl2 was added and the mixture was further stirred at −60 °C for 1 h. Diisopropylethylamine (29 mL) was added at −60 °C, and the mixture was then warmed to room temperature. The solution was washed with 2-M HCl, water, and brine, dried over Na2SO4, and concentrated in vacuo. The crude mixture was purified by column chromatography on silica gel (20% EtOAc/hexane) to afford 621mg (3.2 mmol; 68%) the title compound 10 as a yellow oil: 1H NMR δ 1.99 (m, 2H), 2.23 (m, 2H), 4.12 (m, 2H), 7.23 (s, 2H); 13C NMR δ 23.2, 49.6, 120.7, 134.4, 191.2; IR (CDCl3) νmax 1757, 1735 cm−1; MS (EI) m/z 192 (M+, 9), 135 (100). HRMS (EI) calcd for C10H8O2S: 192.0245. Found: 192.0248. 10’: The same NMR spectra as 10 were obtained probably due to dehydration catalyzed by a trace amount of acid in CDCl3. IR νmax 3101, 2949, 2873, 1731, 1673, 1574 cm−1. Crystal data: Formula: C10H10O3S, 0.40 × 0.20 × 0.20 mm, monoclinic, space group C2/c, a = 16.993(19), b = 13.142(13), c = 10.183(11) Å, β = 123.276(10)°, V = 1901(4) Å3, T = 298 K, Z = 8, ρcalcd = 1.469 g·cm−3, μ = 0.316 mm−1, F(000) = 880. 3702 diffractions measured, 2162 unique, 918 observed [I > 2σ(I)]; Requiv = 0.0342, R1 = 0.0592 [I > 2σ(I)], wR2 = 0.1580 (all); GOF = 1.001. CCDC No. 945204.
Hinsberg reaction of 10
Entry 1: To a stirred solution of 10 (620 mg, 3.2 mmol) and diethyl 2,2’-thiodiglycolate (0.60 mL, 3.3 mmol) in THF (3 mL) was added dropwise a 1-M solution of KO-t-Bu in dry THF (9 mL) and then the mixture was refluxed for 2 h. After being cooled to room temperature, the reaction was quenched by addition of a 1-M HCl solution. The mixture was extracted with EtOAc. The organic layer was washed with water and brine, dried over Na2SO4, and concentrated in vacuo. The mixture was dissolved with a mixture of MeOH and CH2Cl2, and then trimethylsilyldiazomethane in hexane (10 mL, 6.0 mmol) was added. After 10 min, the reaction was quenched with acetic acid. The mixture was extracted with CH2Cl2. The organic extract was washed with a saturated NaHCO3 solution, water, and brine, dried over Na2SO4, and concentrated in vacuo. The residue was purified with column chromatography on silica gel (CH2Cl2/Hexane 1:1) to afford 35 mg (0.097 mmol, 3%) of dimethyl 4,8-dihydro-4,8-ethano- benzo[1,2-c;4,5-c’]dithiophene-1,3-dicarboxylate (11b).
Entry 2: To a solution of 10 (220 mg, 1.14 mmol) and diethyl 2,2’-thiodiglycolate (0.27 mL, 1.49 mmol) in 3 mL of THF was added dropwise a THF solution (3.3 mL) of 1-M KO-t-Bu and the mixture stirred at 45 °C for 2 h. After being cooled to room temperature, the reaction was quenched by addition of a 1-M HCl solution. The mixture was extracted with EtOAc. The organic extract was washed with water and brine, dried over Na2SO4, and concentrated in vacuo. The residue was purified by column chromatography on silica gel with EtOAc/hexane (1/1) to afford 22 mg (0.061 mmol, 5%) of diethyl 4,8-dihydro-4,8-ethanobenzo[1,2-c;4,5-c’]dithiophene-1,3-dicarboxylate (11a) and 53 mg (0.13 mmol, 11%) of diethyl (1R*,3S*,3aR*,4R*,8S*,8aR*)-3a,8a-dihydroxy-1,3,3a,4,8,8a-hexahydro-4,8-ethano- benzo[1,2-c;4,5-c’]dithiophene-1,3-dicarboxylate (endo-12).
Entry 3: To a solution of 10 (220 mg, 1.14 mmol) and diethyl 2,2’-thiodiglycolate (0.27 mL, 1.49 mmol) in 3 mL of THF was added dropwise a THF solution (3.3 mL) of 1-M KO-t-Bu and the mixture was stirred at room temperature for 2 h. The reaction was quenched by addition of a 1-M HCl solution. The mixture was extracted with EtOAc. The organic extract was washed with water and brine, dried over Na2SO4, and concentrated in vacuo. The residue was purified by column chromatography on silica gel with EtOAc/hexane (1/1) to afford 55 mg (0.14 mmol, 12%) of diethyl (1R*,3S*,3aR*,4S*,8R*,8aR*)- 3a,8a-dihydroxy-1,3,3a,4,8,8a-hexahydro-4,8-ethanobenzo[1,2-c;4,5-c’]dithiophene-1,3-dicarboxylate (exo-12).
11a: colorless crystals, mp 157−159 °C; IR νmax 1698, 1317, 1239, 1128, 779 cm−1; 1H NMR δ 1.39 (t, J = 7.2 Hz, 6H), 1.79 (m, 4H), 4.36 (q, J = 7.2 Hz, 4H), 5.21 (br s, 2H), 6.96 (s, 2H); 13C NMR δ 14.5, 26.7, 36.6, 61.5, 115.7, 126.2, 143.4, 152.7, 162.2; MS (FAB+) m/z 363 (M++1), 334 (M+−C2H4). HRMS (FAB+) m/z calcd for C18H18O4S2+H+: 363.0725. Found 363.0719. Crystal data: Formula: C18H18O4S2, 0.40 × 0.04 × 0.20 mm, monoclinic, space group P21/c, a = 11.704(3), b = 14.049(4), c = 24.174(6) Å, β = 117.560(5)°, V = 3523.9(15) Å3, T = 293 K, Z = 8, ρcalcd = 1.366 g·cm−3, μ = 0.320 mm−1, F(000) = 1520. 21741 diffractions measured, 8059 unique, 5461 observed [I > 2σ(I)]; Requiv = 0.0307, R1 = 0.0634 [I > 2σ(I)], wR2 = 0.1543 (all); GOF = 1.106. CCDC No. 945207.
11b: colorless crystals, mp 197-198 °C; IR νmax 1701, 1579, 1500, 1434, 1242, 806 cm−1; 1H NMR δ 1.79 (m, 4H), 3.90 (s, 6H), 5.20 (m, 2H), 6.96 (s, 2H); 13C NMR δ 26.71, 36.62, 52.42, 115.79, 126.00, 143.29, 152.79, 162.58; HRMS (FAB+) m/z calcd for C16H15O4S2+H+: 335.0406. Found: 335.0409.
endo-12: colorless crystals, mp 129−131 °C; IR νmax 3371, 1724, 1443, 1025 cm−1; 1H NMR δ 1.30 (m, 2H), 1.32 (t, J = 7.1 Hz, 6H), 2.18 (m, 2H), 3.44 (s, 2H), 3.57 (m, 2H), 4.25 (br s, 2H) 4.26 (q, J = 7.1 Hz, 4H), 7.08 (s, 2H); 13C NMR δ 14.4, 19.7, 41.7, 57.2, 62.3, 83.1, 118.7, 140.5, 168.5; MS (FAB+) m/z 399 (M++1). HRMS (FAB+) m/z calcd for C18H22O6S2+H+: 399.0936. Found: 399.0941. Anal. Calcd for C18H22O6S2: C, 54.25; H, 5.56. Found: C, 54.24; H, 5.54%. Crystal data: Formula: C18H22O6S2, 0.80 × 0.60 × 0.60 mm, monoclinic, space group P21/c, a = 8.966(4), b = 18.697(3), c = 11.4781(19) Å, β = 90.923(11)°, V = 1923.8(10) Å3, T = 293 K, Z = 4, ρcalcd = 1.376 g·cm−3, μ = 0.307 mm−1, F(000) = 840. 8662 diffractions measured, 4375 unique, 3383 observed [I > 2σ(I)]; Requiv = 0.0223, R1 = 0.0549 [I > 2σ(I)], wR2 = 0.1496 (all); GOF = 1.094. CCDC No. 946728.
exo-12: colorless crystals, mp 188−189 °C; IR νmax 3438, 3095, 2956, 1724, 1137, 1084, 1025 cm−1; 1H NMR δ 1.33 (t, J = 7.1 Hz, 6H), 1.60 (m, 2H), 2.03 (m, 2H), 2.92 (s, 2H, OH), 3.59 (s, 2H), 4.26 (q, J = 7.1 Hz, 4H), 4.28 (br s, 2H), 7.08 (s, 2H); 13C NMR δ 14.4, 21.8, 41.1, 55.2, 62.3, 85.6, 119.1, 139.6, 167.3; MS (EI) m/z 398 (M+), 251, 205, 137, 135. HRMS (EI) m/z calcd for C18H22O6S2: 398.0858. Found: 398.0843. Anal. Calcd for C18H22O6S2: C, 54.25; H, 5.56. Found: C, 53.93; H, 5.08%. Crystal data: Formula: C18H22O6S2, 0.20 × 0.12 × 0.10 mm, monoclinic, space group P21/c, a = 9.7686(17), b = 10.4882(18), c = 18.862(4) Å, β = 111.112(3)°, V = 1802.8(6) Å3, T = 100 K, Z = 4, ρcalcd = 1.468 g·cm−3, μ = 0.328 mm−1, F(000) = 840. 29009 diffractions measured, 4136 unique, 3549 observed [I > 2σ(I)]; Requiv = 0.0367, R1 = 0.0302 [I > 2σ(I)], wR2 = 0.0772 (all); GOF = 1.051. CCDC No. 945206.
Conversion of exo-12 to 11a: To a stirred solution of exo-12 (50 mg, 0.13 mmol) in pyridine (2 mL) was added acetic anhydride (0.25 mL, 2.6 mmol) followed by a few crystals of DMAP. The mixture was heated at 110 °C for 13 h. The mixture was cooled and water was added. The mixture was extracted with CH2Cl2. The organic extract was washed with an aqueous saturated solution of NaHCO3, water, and brine, dried over Na2SO4, and concentrated in vacuo. The residue was purified by column chromatography on silica gel (EtOAc/hexane = 1/1) to afford 11a (31 mg 0.086 mmol, 68%) as colorless crystals.
ACKNOWLEDGEMENTS
This work is partially supported by Grant-in-Aids for the Scientific Research (23350020) from the Japanese Ministry of Education, Culture, Sports, Science and Technology.
References
1. S. Yamago and E. Nakamura, Org. React., 2002, 61, 1; E. Nakamura and S. Yamago, Acc. Chem. Res., 2002, 35, 867. CrossRef
2. W. C. Lineberger and W. T. Borden, Phys. Chem. Chem. Phys., 2011, 13, 11792; CrossRef A. Nicolaides and H. Tomioka, J. Photosci., 2003, 10, 165.
3. Y. Matsui, D. Kawahara, E. Ohta, and H. Ikeda, Phys. Chem. Chem. Phys., 2013, 15, 7064. CrossRef
4. Y. Morita, S. Suzuki, K. Sato, and T. Takui, Nature Chem., 2011, 3, 197; CrossRef Y. Morita, S. Nishida, T. Murata, M. Moriguchi, A. Ueda, M. Satoh, K. Arifuku, K. Sato, and T. Takui, Nature Mater., 2011, 10, 947. CrossRef
5. L. A. Errede and M. Szwarc, Q. Rev. Chem. Soc., 1958, 12, 301; CrossRef J. L. Segra and N. Martin, Chem. Rev., 1999, 99, 3199; CrossRef K. M. Vaeth and K. F. Jensen, Adv. Mater., 1999, 11, 814; CrossRef and 818. CrossRef
6. T. Suzuki, K. Kubomura, and H. Takayama, J. Chem. Soc., Perkin Trans. 1, 1997, 251; CrossRef H. W. Gschwend and H. Haider, J. Org. Chem., 1972, 37, 59; CrossRef W.-D. Liu, C.-C. Chi, I.-F. Pai, A.-T. Wu, and W.-S. Chung, J. Org. Chem., 2002, 67, 9267. CrossRef
7. K. J. Stone, M. M. Greenberg, S. C. Blackstock, and J. A. Berson, J. Am. Chem. Soc., 1989, 111, 3659. CrossRef
8. A recent example, see: P. Kim, S. Ham, J. Oh, H. Uoyama, H. Watanabe, K. Tagawa, H. Uno, and D. Kim, Phys. Chem. Chem. Phys., 2013, 15, 10612. CrossRef
9. H. Uno, S. Ito, M. Wada, H. Watanabe, M. Nagai, A. Hayashi, T. Murashima, and N. Ono, J. Chem. Soc., Perkin Trans. 1, 2000, 4347. CrossRef
10. M. Nakamura, H. Tahara, K. Takahashi, T. Nagata, H. Uoyama, D. Kuzuhara, S. Mori, T. Okujima, H. Yamada, and H. Uno, Org. Biomol. Chem., 2012, 10, 6840; CrossRef H. Uoyama, K. S. Kim, K. Kuroki, J.-Y. Shin, T. Nagata, T. Okujima, H. Yamada, N. Ono, D. Kim, and H. Uno, Chem. Eur. J., 2010, 16, 4063; CrossRef H. Uno, M. Hashimoto, and A. Fujimoto, Heterocycles, 2009, 77, 887; CrossRef H. Uno, K. Nakamoto, K. Kuroki, A. Fujimoto, and N. Ono, Chem. Eur. J., 2007, 13, 5773. CrossRef
11. H. Uoyama, C. Chenxin, H. Tahara, Y. Shimizu, H. Hagiwara, Y. Hanasaki, H. Yamada, T. Okujima, and H. Uno, Heterocycles, 2010, 80, 1187. CrossRef
12. S. S. Zade and M. Bendikov, Angew. Chem. Int. Ed., 2010, 49, 4012; CrossRef H. Uno, Y. Yamashita, M. Kikuchi, H. Watanabe, H. Yamada, T, Okujima, T. Ogawa, and N. Ono, Tetrahedron Lett., 2005, 46, 1981; CrossRef H. Yamada, Y. Yamashita, M. Kikuchi, H. Watanabe, T. Okujima, H. Uno, T. Ogawa, K. Ohara, and N. Ono, Chem. Eur. J., 2005, 11, 6212. CrossRef
13. M. Watanabe, K.-Y. Chen, Y. J. Chang, and T. J. Chow, Acc. Chem. Res., 2013, ASAP DOI: 10.1021/ar400002y; CrossRef A. Seike, K. Yamagami, Y. Kakitani, M. Kuwajima, H. Uoyama, S. Nagaoka, T. Nakae, S. Mori, T. Okujima, and H. Uno, RSC Adv., 2013, 3, 3006.
14. T. Kobayashi and S. Kobayashi, Eur. J. Org. Chem., 2002, 2066. CrossRef
15. D. E. Wolf and K. Folkers, Org. React., 1951, 6, 410.
16. Y. Simizu, Z. Shen, S. Ito, H. Uno, J. Daub, and N. Ono, Tetrahedron Lett., 2002, 43, 8485. CrossRef
17. I. Yamada and R. Noyori, Chemtracts, 2000, 13, 620.
18. A. Wakamiya, D. Yamazaki, T. Nishinaga, T. Kitagawa, and K. Komatsu, J. Org. Chem., 2003, 68, 8305. CrossRef
19. H. Wynberg and H. J. Kooreman, J. Am. Chem. Soc., 1965, 87, 1739. CrossRef
20. CrystalClear Ver. 1.3.6 and CrystalStructure Ver. 4.0.1: Rigaku (3-9-12 Akishima, Tokyo, Japan) and Rigaku/MSC (9009 New Trails Dr., The Woodlands, TX 77381 USA), (2006).
21. SIR2004: A package for crystal structure solution and refinement, Istituto di Gristallografia, Italy; M. C. Burla, R. Caliandro, M. Camalli, B. Carrozzini, G. L. Cascarano, L. De Caro, C. Giacovazzo, G. Polidori, and R. Spagna, J. Appl. Cryst., 2005, 38, 381. CrossRef
22. Shelxl64 (Shelx-2013), Program for the refinement of crystal structures from diffraction data, University of Gottingen, Gottingen, Germany; "A short history of SHELX". G. M. Sheldrick, Acta Cryst., 2008, A64, 112.
23. PLATON, A. L. Spek (2010), A Multipurpose Crystallographic Tool, Utrecht University, Utrecht, The Netherlands; A. L. Spek J. Appl. Cryst., 2009, D65, 148.