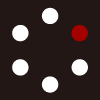
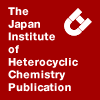
HETEROCYCLES
An International Journal for Reviews and Communications in Heterocyclic ChemistryWeb Edition ISSN: 1881-0942
Published online by The Japan Institute of Heterocyclic Chemistry
e-Journal
Full Text HTML
Received, 26th June, 2013, Accepted, 19th July, 2013, Published online, 23rd July, 2013.
DOI: 10.3987/COM-13-S(S)50
■ Highly Efficient, Enantiocontrolled Total Syntheses of (+)-Heliannuol D and (–)-Helibisabonol A
Yuki Manabe, Makoto Kanematsu, Mayu Osaka, Masahiro Yoshida, and Kozo Shishido*
Graduate School of Pharmaceutical Sciences, University of Tokushima, 1-78-1 Sho-machi, Tokushima 770-8505, Japan
Abstract
Enantiocontrolled total syntheses of (+)-heliannuol D (1) and (−)-helibisabonol A (2) have been accomplished efficiently from a common intermediate 9, derived from the optically pure aryl allyl ether 7 via a chirality transfer through a Lewis acid-mediated Claisen rearrangement and asymmetric dihydroxylation.INTRODUCTION
Heliannuol D (1),1 a representative helianane-type sesquiterpenoid, and helibisabonol A (2),2 an aromatic bisabolene-type sesquiterpenoid, were isolated by Macías and co-workers from the extracts of fresh leaves of Helianthus annuus L. var. SH-222 and dried leaves of Helianthus annuus L. cv. Predovick®, respectively. These natural products have been reported to exhibit allelopathic activity, with potential for becoming lead compounds for a new type of agrochemicals. Furthermore, helibisabonol A (2) is believed to represent the biogenic precursor of 1 and other helianane families.3 The structural features of 1 are a characteristic bicyclic core, an oxepane fused to the aryl ring, and two syn-oriented tertiary stereogenic centers at C7 and C10 on the oxepane ring. The absolute configuration has been established to be (7R,10R) by our enantioselective total synthesis of (−)-1.4a The structure of 2, on the other hand, possesses a hydroquinone moiety and two stereogenic centers at C7 and C10, the absolute configurations of which have been established to be (7R,10R) by our enantioselective total synthesis of (−)-2.5b Although several syntheses of these allelochemicals have been published so far,4,5 more efficient and practical processes have still been required to develop new agrochemicals. In this report, we describe highly efficient, enantiocontrolled total syntheses of (+)-heliannuol D (1) and (–)-helibisabonol A (2) (Figure 1).
RESULTS AND DISCUSSION
During the course of our enantioselective syntheses of heliannuol D (1) and helibisabonol A (2), we have developed two general strategies for the construction of the tertiary benzylic stereogenic center at C7.4a,b,d,5b A lipase-mediated desymmetrization of σ-symmetrical diol 36 provided the acetate 4 in 34% yield with 84% ee and a diastereoselective conjugate addition of a methyl group to the enone 5, which possesses a chiral auxiliary (i.e., the Evans’ oxazolidinone),7 produced 6 in 71% yield with 85% de. While the chiral building blocks 4 and 6 thus prepared have been successfully converted to 1 and 2, a couple of issues, namely slightly lower enantio-(or diastereo-)selectivities and longer reaction steps, for more efficient access to the natural products 1 and 2 have remained to be overcome (Scheme 1).
Recently, we reported a strategy for accessing a chiral building block 8 that can serve for the synthesis of helianane allelochemicals, heliannuols A and K, by using a substrate-controlled chirality transfer in Claisen rearrangement (7 → 8).8 Since the phenol 8 can be obtained in good yield with high enantiomeric excess, it was expected to be useful as a starting chiral building block for the efficient syntheses of 1 and 2. Our retrosynthetic analysis is shown in Scheme 2. For the syntheses of both 1 and 2, we chose the diol 9 as a common intermediate, because it has been successfully converted to heliannuol D (1) by sequential epoxidation, K2CO3-mediated desilylative [7-exo] cyclization of 10, and deprotection of the methoxymethyl (MOM) ether in our laboratories.4d On the other hand, helibisabonol A (2) might be obtained from 9 by a simple acidic hydrolysis. The key diol 9 can be derived from 8 through a three-carbon elongation9 and an asymmetric dihydroxylation10 (Scheme 2).
The chiral building block 8 was prepared from 3-methyl-4-(methoxymethoxy)phenol (11) and (S,E)-1-(benzyloxy)pent-3-en-2-ol (12) as previously described.8 The Mitsunobu reaction between 11 and 12 in the presence of 1,1-(azodicarbonyl)dipiperidine (ADDP) and nBu3P provided a chromatographically separable mixture of 7 (81%, >99% ee; by HPLC analysis) and the regioisomer 13 (7%), which was derived via SN2’ process. The Claisen rearrangement of 7 was carried out with 3 equiv. of Me3Al in hexane at room temperature for 0.5 h; the requisite 8 was obtained in 88% yield (>99% ee; by HPLC analysis), along with 14 (10%), which would be generated via the disfavored boat-like transition state. The phenolic hydroxyl function was protected as the TBS ether to give 15, which was exposed to catalytic hydrogenation conditions to produce the alcohol 16. It was then oxidized under Swern conditions, and the resulting aldehyde was subjected to Kocienski-Julia olefination with the sulfone 1711 to give the trisubstituted alkene 18 in good overall yield. On exposure of 18 to an asymmetric dihydroxylation protocol using AD-mix-β and MeSO2NH2, the diol 9, a common key intermediate, was obtained quantitatively as a single product12 (Scheme 3).
The diol 9 was then exposed to N-tosylimidazole in the presence of NaH13 to provide the epoxide 10, which without purification was treated with K2CO3 in methanol at 50 °C14 and provided the oxepane 19 in 81% yield for the two steps through deprotection of TBS ether and spontaneous 7-exo cyclization sequence.4d Finally, acidic hydrolysis of 19 with 6 M aqueous hydrochloric acid in THF at room temperature produced heliannuol D (1) in 91% yield, of which the spectroscopic (1H and 13C NMR) properties as well as optical rotation, {[α] = +18.1 (c 0.38, CHCl3); lit.1 [α] = +16 (c 0.1, CHCl3)}, were identical with those of the natural product. Thus, our fourth-generation enantioselective total synthesis of heliannuol D (1) has been accomplished, with the longest linear sequence having ten steps, in 48% yield from the phenol 11. For the synthesis of helibisabonol A (2), the diol 9 was treated with p-TsOH∙H2O in methanol at room temperature to give 2 quantitatively. The spectroscopic (1H and 13C NMR) properties as well as optical rotation, {[α] = −7.8 (c 0.40, acetone); lit.5b [α] = −6.9 (c 0.33, acetone)}, were identical with those of the synthesized helibisabonol A.5b Thus, the second-generation enantioselective total synthesis of helibisabonol A (2) has also been accomplished, with the longest linear sequence having eight steps, in 65% yield from the phenol 11 (Scheme 4).
In conclusion, we have accomplished alternative enantiocontrolled total syntheses of (+)-heliannuol D (1) and (–)-helibisabonol A (2) from a common intermediate 9, which was derived from 8, a chiral building block prepared via a chirality transfer through a Lewis acid-mediated Claisen rearrangement of the optically pure aryl allyl ether 7. In particular, synthesis of heliannuol D was achieved employing a highly efficient K2CO3-mediated desilylative biomimetic [7-exo] cyclization of the epoxide 10 as the key step. These syntheses were efficiently completed with the longest linear sequence having ten steps in 48% yield for 1, and eight steps in 65% for 2, from 3-methyl-4-(methoxymethoxy)phenol (11), respectively. The synthetic route developed herein is general and efficient and would be applicable to the synthesis of other helianane-type sesquiterpenoids.
(2R,3E)-4-[1-(Benzyloxy)pent-3-en-2-yloxy]-1-(methoxymethoxy)-2-methylbenzene (7)8 and (3E)-4-[5-(Benzyloxy)pent-3-en-2-yloxy]-1-(methoxymethoxy)-2-methylbenzene (13)8: To a stirred solution of 3-methyl-4-(methoxymethoxy)phenol (11) (2.02 g, 12.0 mmol), (S,E)-1-(benzyloxy)pent-3-en-2-ol (12) (3.00 g, 15.6 mmol) and nBu3P (4.49 ml, 18.0 mmol) in benzene (34 mL) was added 1,1-(azodicarbonyl)dipiperidine (4.54 g, 18.0 mmol) at 0 °C. After being stirred for 30 min at 60 °C, the reaction mixture was diluted with hexane, and then filtered through a pad of silica gel and concentrated. The residue was chromatographed on silica gel with hexane/AcOEt (33:1 v/v) as eluent to afford aryl allyl ether 7 (3.33 g, 81%, >99% ee) as a colorless oil and the regio isomer 13 (282 mg, 7%) as a colorless oil.
Compound 7: [α] –12.99 (c 3.29, CHCl3); IR (neat) 2917, 1498, 1218, 1152, 1011, 737, 698 cm–1; 1H NMR (400 MHz, CDCl3) δ 1.69 (d, J = 6.4 Hz, 3H), 2.21 (s, 3H), 3.49 (s, 3H), 3.59 (dd, J = 4.4 and 10.4 Hz, 1H), 3.67 (dd, J = 7.2 and 10.8 Hz, 1H), 4.59 (d, J = 12.0 Hz, 1H), 4.64 (d, J = 12.0 Hz, 1H), 4.69 (dt, J = 5.2 and 6.4 Hz, 1H), 5.11 (s, 2H), 5.45 (ddd, J = 1.6, 6.4 and 15.6 Hz, 1H), 5.77 (dq, J = 6.4 and 15.6 Hz, 1H), 6.69 (dd, J = 2.8 and 9.2 Hz, 1H), 6.76 (d, J = 2.4 Hz, 1H), 6.92 (d, J = 8.8 Hz, 1H), 7.31 (m, 5H); 13C NMR (100 MHz, CDCl3) δ 16.4 (CH3), 17.9 (CH3), 55.9 (CH3), 72.9 (CH2), 73.4 (CH2), 78.7 (CH), 95.4 (CH2), 113.9 (CH), 115.3 (CH), 119.3 (CH), 127.5 (CH), 127.6 (CH), 128.1 (CH), 128.3 (CH), 128.6 (C), 129.3 (CH), 138.3 (C), 149.8 (C), 152.9 (C); HRMS (ESI-TOF) m/z calcd for C21H27O4 [M+H]+ 343.1909, found 343.1904; Enantiomeric excess was determined by HPLC analysis [Chiralcel OD column, 1.0% isopropanol/hexane, 1.0 mL/min, λ=254 nm, retention times 22.8 min (S) and 30.6 min (R)].
Compound 13: [α] +26.31 (c 0.68, CHCl3); IR (neat) 2926, 2363, 1498, 1218, 1151, 1075, 1010, 738, 698 cm–1; 1H NMR (500 MHz, acetone-d6) δ 1.36 (d, J = 6.5 Hz, 3H), 2.17 (s, 3H), 3.42 (s, 3H), 3.99 (dd, J = 5.5 and 12.5 Hz, 1H), 4.02 (dd, J = 5.5 and 12.5 Hz, 1H), 4.42 (d, J = 12.0 Hz, 1H), 4.45 (d, J = 12.0 Hz, 1H), 4.83 (quint., J = 6.0 Hz, 1H), 5.10 (s, 2H), 5.79 (dd, J = 5.5 and 16.0 Hz, 1H), 5.84 (dt, J = 5.0 and 15.5 Hz, 1H), 6.70 (dd, J = 3.0 and 9.0 Hz, 1H), 6.77 (d, J = 2.5 Hz, 1H), 6.93 (d, J = 9.0 Hz, 1H), 7.25-7.34 (m, 5H); 13C NMR (125 MHz, CDCl3) δ 16.4 (CH3), 17.9 (CH3), 55.9 (CH3), 72.9 (CH2), 73.4 (CH2), 78.7 (CH), 95.4 (CH2), 113.9 (CH), 115.3 (CH), 119.3 (CH), 127.5 (CH), 127.6 (CH), 128.1 (CH), 128.3 (CH), 128.6 (C), 129.3 (CH), 138.3 (C), 149.8 (C), 152.9 (C); HRMS (ESI-TOF) m/z calcd for C21H27O4 [M+H]+ 343.1909, found 343.1904.
(2R,3E)-2-[5-(Benzyloxy)pent-3-en-2-yl]-4-(methoxymethoxy)-5-methylphenol (8)8 and (2S,3Z)-2-[5-(Benzyloxy)pent-3-en-2-yl]-4-(methoxymethoxy)-5-methylphenol (14)8: To a stirred solution of aryl allyl ether 7 (500 mg, 1.46 mmol) in hexane (10 mL) was added Me3Al (1.08 M in hexane, 4.0 mL, 4.38 mmol) at 0 °C. After being stirred for 30 min at room temperature, the reaction mixture was diluted with Et2O. The resultant mixture was quenched with water, and then filtered through a pad of Celite. The residue upon workup was chromatographed on silica gel with hexane/AcOEt (9:1 v/v) as eluent to afford phenol 8 (441 mg, 88%, >99% ee) as a colorless oil and the Z-isomer 14 (48.5 mg, 10%) as a colorless oil.
Compound 8: [α] −3.83 (c 1.20, CHCl3); IR (neat) 3375, 2960, 2928, 1513, 1453, 1398, 1148, 1004, 740, 698 cm–1; 1H NMR (400 MHz, CDCl3) δ 1.38 (d, J = 6.8 Hz, 3H), 2.18 (s, 3H), 3.49 (s, 3H), 3.66 (quint., J = 7.2 Hz, 1H), 4.02 (d, J = 6.0 Hz, 2H), 4.50 (s, 2H), 4.60 (s, OH, D2O exchangeable, 1H), 5.09 (s, 2H), 5.71 (ddt, J = 1.2, 6.0 and 15.6 Hz, 1H), 5.94 (dd, J = 6.0 and 15.6 Hz, 1H), 6.61 (s, 1H), 6.82 (s, 1H), 7.36 (m, 5H); 13C NMR (100 MHz, CDCl3) δ 15.8 (CH3), 19.3 (CH3), 36.1 (CH), 56.0 (CH3), 70.6 (CH2), 71.9 (CH2), 95.8 (CH2), 114.9 (CH), 118.3 (CH), 125.7 (CH), 126.8 (C), 127.6 (CH), 127.8 (CH), 128.3 (CH), 128.8 (CH), 138.0 (CH), 138.2 (C), 148.1 (C), 149.5 (C); HRMS (ESI-TOF) m/z calcd for C21H27O4 [M+H]+ 343.1909, found 343.1902; Enantiomeric excess was determined by HPLC analysis [Chiralcel AD column, 10% isopropanol/hexane, 1.0 mL/min, λ =254 nm, retention times 22.1 min (R) and 25.3min (S)].
Compound 14: [α] +154.1 (c 2.59, CHCl3); IR (neat) 3365, 2960, 2927, 1513, 1454, 1398, 1190, 1149, 1007, 738, 699 cm–1; 1H NMR (400 MHz, CDCl3) δ 1.32 (d, J = 7.2 Hz, 3H), 2.18 (s, 3H), 3.50 (s, 3H), 3.93 (dd, J = 6.0 and 11.2 Hz, 1H), 4.03 (dq, J = 2.8 and 6.8 Hz, 1H), 4.23 (dd, J = 4.4 and 11.2 Hz, 1H), 4.58 (d, J = 12.0 Hz, 1H), 4.62 (d, J = 12.0 Hz, 1H), 5.10 (s, 2H), 5.55 (dd, J = 8.4 and 10.8 Hz, 1H), 5.60 (dt, J = 6.4 and 10.8 Hz, 1H), 6.42 (s, OH, D2O exchangeable, 1H), 6.64 (s, 1H), 6.87 (s, 1H), 7.37 (m, 5H); 13C NMR (100 MHz, CDCl3) δ 15.8 (CH3), 19.8 (CH2), 31.5 (CH), 56.0 (CH2), 65.1 (CH3), 72.8 (CH3), 95.9 (CH3), 113.5 (CH), 118.9 (CH), 122.9 (CH), 127.0 (C), 127.0 (C), 127.9 (CH), 128.2 (CH), 128.5 (CH), 137.3 (C), 140.0 (CH), 148.8 (C), 149.5 (C); HRMS (ESI-TOF) m/z calcd for C21H27O4 [M+H]+ 343.1909, found 343. 1895.
(2R,3E)-{2-[5-(Benzyloxy)pent-3-en-2-yl]-4-(methoxymethoxy)-5-methylphenoxy}(tert-butyl)-dimethylsilane (15): To a stirred solution of phenol 8 (200 mg, 0.584 mmol) in CH2Cl2 (3.25 mL) were added imidazole (159 mg, 2.34 mmol), TBSCl (211 mg, 1.40 mmol) and 4-DMAP (14.3 mg, 0.117 mmol) at 0 °C. After being stirred for 30 min at room temperature, the resultant mixture was quenched with H2O and extracted with AcOEt. The combined extracts were washed with brine and the residue upon workup was chromatographed on silica gel with hexane/AcOEt (5:1 v/v) as eluent to afford TBS ether 15 (258 mg, 97%) as a colorless oil; [α] +14.6 (c 1.08, CHCl3); IR (neat) 2929, 1501, 1391, 1193, 1151, 1012, 838, 780 cm–1; 1H NMR (400 MHz, CDCl3) δ 0.21 (s, 3H), 0.22 (s, 3H), 1.00 (s, 9H), 1.28 (d, J = 6.8 Hz, 3H), 2.17 (s, 3H), 3.47 (s, 3H), 3.87 (sext., J = 6.0 Hz, 1H), 4.01 (d, J = 5.6 Hz, 2H), 4.49 (s, 2H), 5.08 (s, 2H), 5.61 (ddd, J = 1.6, 5.6 and 15.6 Hz, 1H), 5.90 (dd, J = 5.6 and 15.6 Hz, 1H), 6.57 (s, 1H), 6.80 (s, 1H), 7.28-7.34 (m, 5H); 13C NMR (100 MHz, CDCl3) δ −4.1 (CH3), 16.1 (CH3), 18.3 (C), 20.1 (CH3), 25.9 (CH3), 34.4 (CH), 56.0 (CH3), 71.0 (CH2), 71.8 (CH2), 93.4 (C), 95.7 (CH2), 100.6 (C), 114.5 (CH), 120.7 (CH), 124.9 (CH), 126.0 (C), 127.5 (C), 127.8 (C), 128.3 (C), 133.9 (C), 138.7 (CH), 147.1 (C); HRMS (ESI-TOF) m/z calcd for C27H41O4Si [M+H]+ 457.2774, found 457.2754.
(4R)-4-[2-(tert-Butyldimethylsilyloxy)-5-(methoxymethoxy)-4-methylphenyl]pentan-1-ol (16): A suspension of benzyl ether 15 (463 mg, 1.17 mmol) and Pd-C (46.3 mg, 10% w/w) in THF (9.26 mL) was stirred under 5 atm of hydrogen gas. After being stirred for 15 h at room temperature, the reaction mixture was filtered through a pad of Celite and concentrated. The residue was chromatographed on silica gel with hexane/AcOEt (4:1 v/v) as eluent to afford alcohol 16 (360 mg, 95%) as a colorless oil; [α] +10.7 (c 1.02, CHCl3); IR (neat) 3361, 2930, 2858, 1502, 1472, 1391, 887, 838 cm–1; 1H NMR (400 MHz, CDCl3) δ 0.20 (s, 3H), 0.21 (s, 3H), 1.00 (s, 9H), 1.17 (d, J = 6.0 Hz, 3H), 1.17-1,29 (m, 1H), 1.40-1.63 (m, 5H), 2.17 (s, 3H), 3.02-3.20 (m, 1H), 3.50 (s, 3H), 3.58 (t, J = 6.0 Hz, 1H), 5.09 (d, J = 6.4 Hz, 1H), 5.13 (d, J = 6.4 Hz, 1H), 6.56 (s, 1H), 6.82 (s, 1H); 13C NMR (100 MHz, CDCl3) δ −4.2 (CH3), −4.1 (CH3), 16.0 (CH3), 18.2 (C), 21.3 (CH3), 25.8 (CH3), 30.9 (CH2), 31.4 (CH), 33.3 (CH2), 56.0 (CH3), 63.1 (CH2), 95.6 (CH2), 113.6 (CH), 120.6 (CH), 125.4 (C), 135.4 (C), 147.4 (C), 149.8 (C); HRMS (ESI-TOF) m/z calcd for C20H36O4SiNa [M+Na]+ 391.2281, found 391.2272.
(2R)-tert-Butyl[4-(methoxymethoxy)-5-methyl-2-(6-methylhept-5-en-2-yl)phenoxy]dimethylsilane (18): To a stirred solution of oxalyl chloride (0.248 mL, 2.93 mmol) in CH2Cl2 (7.00 mL) was added DMSO (0.333 mL, 4.69 mmol) at −78 °C, and then a solution of alcohol 16 (360 mg, 0.978 mmol) in CH2Cl2 (3.00 mL) was added dropwise. After being stirred for 30 min at −78 °C, Et3N (0.825 mL, 5.87 mmol) was added and then stirring was continued for 10 min at the same temperature. The reaction mixture was allowed to warm to room temperature, and the stirring was continued for 30 min. The resultant mixture was diluted with H2O and extracted with Et2O. The combined extracts were washed with brine. The residue upon workup was the corresponding aldehyde (430 mg), a yellow oil, which was used to the next reaction without further purification; 1H NMR (400 MHz, CDCl3) δ 0.19 (s, 3H), 0.22 (s, 3H), 1.00 (s, 9H), 1.19 (d, J = 7.2 Hz, 3H), 1.81-1.91 (m, 2H), 2.17 (s, 3H), 2.25-2.39 (m, 2H), 3.15 (sext, J = 7.2 Hz, 1H), 3.45 (s, 3H), 5.10 (s, 2H), 6.57 (s, 1H), 6.81 (s, 1H), 9.68 (t, J = 1.2 Hz, 1H).
To a stirred solution of HMDS (0.51 ml, 2.45 mmol) in THF (15.0 mL) was added nBuLi (1.6 M in hexane, 1.53 mL, 2.45 mmol) at 0 °C. After being stirred for 15 min at 0 °C, the solution was cooled to −78 °C, and then a solution of sulfone 17 (617 mg, 2.45 mmol) in THF (5 mL) was added dropwise. After being stirred for 30 min at −78 °C, a solution of crude aldehyde (0.978 mmol) in THF (5 mL) was added at −78 °C, and then stirring was continued for 10 min at the same temperature. The resultant mixture was allowed to warm to room temperature. After being stirred for 40 min at the room temperature, the reaction mixture was quenched with H2O and extracted with Et2O. The combined extracts were washed with brine, and the residue upon workup was chromatographed on silica gel hexane/AcOEt (15:1 v/v) as eluent to afford the trisubstituted alkene 18 (379 mg, 99% for two steps) as a colorless oil; [α] −19.2 (c 1.14, CHCl3); IR (neat) 2957, 2928, 1501, 1391, 1255, 1215, 1193, 1151 cm–1; 1H NMR (400 MHz, CDCl3) δ 0.20 (s, 3H), 0.21 (s, 3H), 1.01 (s, 9H), 1.13 (d, J = 6.8 Hz, 3H), 1.48-1.60 (m, 2H), 1.54 (s, 3H), 1.66 (s, 3H), 1.93 (quint, J = 7.2 Hz, 2H), 2.17 (s, 3H), 3.11 (sext, J = 6.8 Hz, 1H), 3.50 (s, 3H), 5.08-5.12 (m, 1H), 5.10 (s, 2H), 6.55 (s, 1H), 6.82 (s, 1H); 13C NMR (100 MHz, CDCl3) δ −4.2 (CH3), −4.1 (CH3), 16.0 (CH3), 17.6 (CH3), 18.2 (C), 21.2 (CH3), 25.7 (CH3), 25.8 (CH3), 26.2 (CH2), 31.2 (CH), 37.2 (CH2), 56.0 (CH3), 95.8 (CH2), 113.7 (CH), 120.5 (CH), 124.8 (CH), 125.2 (C), 131.1 (C), 136.0 (C), 147.4 (C), 149.9 (C); HRMS (ESI-TOF) m/z calcd for C23H41O3Si [M+H]+ 393.2825, found 393.2823.
(3R,6R)-6-[2-(tert-Butyldimethylsilyloxy)-5-(methoxymethoxy)-4-methylphenyl]-2-methylheptane-2,3-diol (9): To a stirred solution of AD-mix-β (319 mg, 0.407 mmol) and CH3SO2NH2 (19.4 mg, 0.204 mmol) in tBuOH/H2O (1/1, 2 mL) was added alkene 18 (80.0 mg, 0.204 mmol) at 0 °C. After being stirred at room temperature for 15 h, the reaction mixture was quenched with Na2SO3 (0.15 ml, 1.73 mmol) at 0 °C. The resultant mixture was stirred for 1 h at room temperature before being extracted with AcOEt. The combined extracts were washed with 1 M aqueous KOH and brine, and the residue upon workup was chromatographed on silica gel with hexane/AcOEt (3:2 v/v) as eluent to afford diol 9 (86.9 mg, quant.) as a colorless oil; [α] +1.0 (c 1.26, CHCl3); IR (neat) 3422, 2859, 1502, 1463, 1391, 1255, 1194, 1151, 1075, 1012 cm–1; 1H NMR (400 MHz, CD3OD) δ 0.20 (s, 3H), 0.22 (s, 3H), 1.03 (s, 9H), 1.06 (s, 3H), 1.10 (s, 3H), 1.16 (d, J = 6.8 Hz, 3H), 1.25-1.34 (m, 1H), 1.45-1.56 (m, 2H), 1.80-1.89 (m, 1H), 2.15 (s, 3H), 3.13-3.19 (m, 1H), 3.19-3.29 (m, 1H), 3.47 (s, 3H), 5.10 (dd, J = 6.4 and 8.2 Hz, 2H), 6.59 (s, 1H), 6.88 (s, 1H); 13C NMR (100 MHz, CDCl3) δ −4.2 (CH3), −4.1 (CH3), 16.0 (CH3), 18.2 (C), 21.3 (CH3), 23.1 (CH3), 25.8 (CH3), 26.4 (CH3), 30.0 (CH2), 31.9 (CH), 34.6 (CH2), 56.0 (CH3), 73.0 (C), 78.9 (CH), 95.6 (CH2), 113.6 (C), 120.7 (C), 125.5 (C), 135.5 (C), 147.4 (C), 149.9 (C); HRMS (ESI-TOF) m/z calcd for C23H42O5NaSi [M+Na]+ 449.2699, found 449.2710.
2-(2R,5R)-(7-Methoxymethoxy-5,8-dimethyl-2,3,4,5-tetrahydrobenzo[b]oxepin-2-yl)propan-2-ol (19): To a stirred suspension of NaH (14.0 mg, 0.352 mmol) in THF (0.40 mL) was added a solution of diol 9 (30.0 mg, 70.3 µmol) in THF (0.40 mL) at 0 °C. After being stirred for 30 min, the reaction mixture was cooled to −78 °C, and then N-tosylimidazole (18.8 mg, 84.4 µmol) was added at the same temperature. After being stirred for 4 h at room temperature, the reaction mixture was treated with saturated aqueous NH4Cl and extracted with AcOEt. The combined extracts were washed with brine, and the residue upon workup was the corresponding epoxide 10 (32.3 mg), a colorless oil, which was used to the next reaction without further purification; 1H NMR (400 MHz, CDCl3) δ 0.20 (s, 3H), 0.21 (s, 3H), 1.00 (s, 9H), 1.17 (d, J = 7.2 Hz, 3H), 1.19 (s, 3H), 1.26 (s, 3H), 1.40-1.65 (m, 3H), 1.68-1.83 (m, 1H), 2.17 (s, 3H), 2.69 (t, J = 6.4 Hz, 1H), 3.17 (sext, J = 7.2 Hz, 1H), 3.50 (s, 3H), 5.10 (s, 2H), 6.56 (s, 1H), 6.82 (s, 1H).
To a stirred solution of crude epoxide 10 (70.3 µmol) in MeOH (2.30 mL) was added K2CO3 (97.2 mg, 0.703 mmol) at room temperature. After being stirred for 4 h at 50 °C, the reaction mixture was diluted with Et2O. The resultant mixture was quenched with H2O, and extracted with Et2O. The combined extracts were washed with brine, and the residue upon workup was chromatographed on silica gel with hexane/AcOEt (7:3 v/v) as eluent to afford O-MOM-heliannuol D (19) (16.8 mg, 81% for two steps) as a colorless oil; [α] +19.0 (c 0.40, CHCl3); IR (neat) 3450, 2928, 1500, 1452, 1397, 1212, 1197, 1180, 1151, 1067, 1020 cm–1; 1H NMR (400 MHz, CDCl3) δ 1.28 (s, 3H), 1.28 (s, 3H), 1.29 (d, J = 6.8 Hz, 3H), 1.70-1.81 (m, 2H), 1.87-1.94 (m, 1H), 2.00-2.11 (m, 1H), 2.17 (s, 3H), 2.64 (brs, OH, D2O exchangeable, 1H), 2.93-2.97 (m, 1H), 3.30 (d, J = 11.2 Hz, 1H), 3.50 (s, 3H), 5.14 (dd, J = 6.8 and 12.0 Hz, 2H), 6.75 (s, 1H), 6.80 (s, 1H); 13C NMR (100 MHz, CDCl3) δ 15.7 (CH3), 18.6 (CH3), 24.5 (CH3), 25.6 (CH2), 26.1 (CH3), 31.8 (CH2), 38.9 (CH), 56.0 (CH3), 72.6 (C), 90.5 (CH), 95.2 (CH2), 115.6 (CH), 123.5 (CH), 126.2 (C), 137.8 (C), 151.5 (C), 152.6 (C); HRMS (ESI-TOF) m/z calcd for C17H27O4 [M+H]+ 295.1909, found 295.1916.
(+)-Heliannuol D (1)4: To a stirred solution of MOM ether 19 (9.5 mg, 32.3 µmol) in THF (0.2 mL) was added 6 M aqueous HCl (0.5 mL) at room temperature. After being stirred for 3 h at room temperature, the reaction mixture was extracted with Et2O. The combined extracts were washed with water and brine, and the residue upon workup was chromatographed on silica gel with hexane/AcOEt (7:3 v/v) as eluent to afford concentrated in vacuo to afford heliannuol D (1) (7.4 mg, 91%) as a colorless crystalline solid; mp 157-158 °C (lit.4f,4h 161-162 °C); [α] +18.1 (c 0.38, CHCl3); IR (neat) 3365, 2931, 1508, 1417, 1192, 1020 cm–1; 1H NMR (400 MHz, CDCl3) δ 1.28 (s, 6H), 1.28 (d, J = 7.2 Hz, 3H), 1.70-1.79 (m, 2H), 1.88-1.92 (m, 1H), 1.99-2.09 (m, 1H), 2.16 (s, 3H), 2.70 (brs, OH, D2O exchangeable, 1H), 2.90 (brs, 1H), 3.30 (d, J = 10.8 Hz, 1H), 4.55 (brs, OH, D2O exchangeable, 1H), 6.54 (s, 1H), 6.73 (s, 1H); 13C NMR (125 MHz, CDCl3) δ 15.3 (CH3), 18.6 (CH3), 24.4 (CH3), 25.5 (CH3), 26.1 (CH2), 31.8 (CH2), 38.5 (CH), 72.7 (C), 90.5 (CH), 115.8 (CH) 122.1 (C), 123.5 (CH), 138.1 (C), 149.6 (C), 151.7 (C); HRMS (ESI-TOF) m/z calcd for C15H23O3 [M+H]+ 251.1647, found 251.1643.
(−)-Helibisabonol A (2)5b: To a stirred solution of diol 9 (18.2 mg, 42.7 µmol) in MeOH (0.9 mL) was added p-TsOH・H2O (20.3 mg, 0.107 mmol) at room temperature. After being stirred for 5 h, the reaction mixture was quenched with saturated aqueous NaHCO3 and extracted with AcOEt. The combined extracts were washed with brine, and the residue upon workup was chromatographed on silica gel with hexane/AcOEt (1:1 v/v) as eluent to afford helibisabonol A (2) (11.9 mg, quant.) as a colorless oil; [α] –7.8 (c 0.40 in acetone); IR (neat): 3367, 2963, 1703, 1419, 1193 cm–1; 1H NMR (500 MHz, acetone-d6) δ 1.06 (s, 6H), 1.14 (d, J=7.0 Hz, 3H), 1.22-1.31 (m, 1H), 1.50 (dddd, J=15.5, 10.0, 5.5 and 2.0 Hz, 1H), 1.58 (dddd, J=13.5, 10.0, 7.5 and 5.5 Hz, 1H), 1.78 (dddd, J=13.5, 10.0, 7.0 and 5.5 Hz, 1H), 2.08 (s, 3H), 3.11 (sext, J=7.0 Hz, 1H), 3.27 (s, D2O exchangeable, 1H), 3.27 (ddd, J=10.0, 5.0 and 2.0 Hz, 1H), 3.59 (d, J=5.0 Hz, D2O exchangeable, 1H), 6.55 (s, 1H), 6.60 (s, 1H), 7.26 (s, D2O exchangeable, 1H), 7.36 (s, D2O exchangeable, 1H); 13C NMR (125 MHz, acetone-d6) δ 15.7 (CH3), 21.4 (CH3), 24.7 (CH3), 25.8 (CH3), 30.0 (CH2), 32.3 (CH), 35.8 (CH2), 72.7 (C), 79.5 (CH), 113.7 (CH), 118.3 (CH), 122.2 (C), 132.4 (C), 147.8 (C), 149.1 (C); HRMS (ESI-TOF) m/z calcd for C15H25O4 [M+H]+ 269.1753, found 269.1758.
ACKNOWLEDGEMENT
This work was supported financially by a Grant-in-Aid for Scientific Research from the Japan Society for the Promotion of Sciences (JSPS) and a Grant-in-Aid from the Program for the Promotion of Basic and Applied Research for Innovations in the Bio-oriented Industry (BRAIN).
References
1. F. A. Macías, J. M. G. Molinillo, R. M. Varela, A. Torres, and F. R. Fronczek, J. Org. Chem., 1994, 59, 8261. CrossRef
2. F. A. Macías, A. Torres, J. M. G. Galindo, R. M. Varela, J. A. Alvarez, and J. M. G. Molinillo, Phytochemistry, 2002, 61, 687. CrossRef
3. For a review, see: J. R. Vyvyan, Tetrahedron, 2002, 58, 1631. CrossRef
4. Heliannuol D: for enantioselective syntheses, see (a) K. Takabatake, I. Nishi, M. Shindo, and K. Shishido, J. Chem. Soc., Perkin Trans. 1, 2000, 1807; CrossRef (b) H. Kishuku, T. Yoshimura, T. Kakehashi, M. Shindo, and K Shishido, Heterocycles, 2003, 61, 125; CrossRef (c) J. Zhang, X. Wang, W. Wang, W. Quan, X. She, and X. Pan, Tetrahedron, 2007, 63, 6990; CrossRef (d) M. Osaka, M. Kanematsu, M. Yoshida, and K. Shishido, Tetrahedron; Asymmetry, 2010, 21, 2319; CrossRef for a formal enantioselective synthesis, see (e) J.-Q. Li, X. Quan, and P. G. Anderson, Chem. Eur. J., 2012, 18, 10609; CrossRef for racemic syntheses, see (f) J. R. Vyvyan and R. E. Looper, Tetrahedron Lett., 2000, 41, 1151; CrossRef (g) K. Tuhina, D. R. Bhowmik, and R. V. Venkateswaran, Chem. Commun., 2002, 634; CrossRef (h) F. A. Macías, D. Chinchilla, J. M. G. Molinillo, D. Marín, R. M. Varela, and A. Torres, Tetrahedron, 2003, 59, 1679; CrossRef (i) F. Doi, T. Ohta, T. Ogamino, T. Sugai, K. Higashinakasu, K. Yamada, H. Shigemori, K. Hasegawa, and S. Nishiyama, Phytochemistry, 2004, 65, 1405; CrossRef (j) S. K. Sabui and R. V. Venkateswaran, Tetrahedron Lett., 2004, 45, 983; CrossRef (k) S. K. Sabui and R. V. Venkateswaran, Tetrahedron Lett., 2004, 45, 2047; CrossRef (l) M. Osaka, M. Kanematsu, M. Yoshida, and K. Shishido, Heterocycles, 2010, 80, 1003. CrossRef
5. Helibisabonol A: for enantioselective syntheses, see (a) H. M. Sirat, N. M. Hong, and M. H. Jauri, Tetrahedron Lett., 2007, 48, 457; CrossRef (b) A. Miyawaki, M. Osaka, M. Kanematsu, M. Yoshida, and K. Shishido, Tetrahedron, 2011, 67, 6753; CrossRef for a racemic synthesis, see (c) F. A. Macías, D. Martín, D. Chinchilla, and J. M. G. Molinillo, Tetrahedron Lett., 2002, 43, 6417. See also ref. 4e for a formal enantioselective synthesis. CrossRef
6. For reviews, see: (a) K. Shishido and T. Bando, J. Mol. Catal. B: Enzym., 1998, 5, 183; CrossRef (b) K. Shishido, Chem. Ind., 2004, 55, 623; (c) T. Kamei, S. Morimoto, and K. Shishido, J. Synth. Org. Chem. Jpn., 2006, 64, 1021. CrossRef
7. X. Qian, K. C. Russell, L. W. Boteju, and V. J. Hruby, Tetrahedron, 1995, 51, 1033 and references cited therein. CrossRef
8. M. Kanematsu, K. Soga, Y. Manabe, S. Morimoto, M. Yoshida, and K. Shishido, Tetrahedron, 2011, 67, 4758 and references cited therein. CrossRef
9. A. Miyawaki, Y. Manabe, M. Yoshida, and K. Shishido, Tetrahedron Lett., 2012, 53, 1236. CrossRef
10. For a review, see: H. C. Kolb, M. S. VanNieuwenhze, and K. B. Sharpless, Chem. Rev., 1994, 94, 2483. CrossRef
11. (a) C. Meyers and E. M. Carreira, Angew. Chem. Int. Ed., 2003, 42, 694; CrossRef (b) C. Marti and E. M. Carreira, J. Am. Chem. Soc., 2005, 127, 11505. CrossRef
12. The absolute configuration of a newly generated stereogenic center was determined by the Kusumi-Mosher method. The stereochemical homogeneity of 9 was confirmed by the 1H NMR of the MTPA ester and the 13C NMR.
13. D. R. Hicks and B. Fraser-Reid, Synthesis, 1974, 203. CrossRef
14. When the reaction was conducted at room temperature, a longer reaction time (68 h) was needed and the yield of 19 was comparable (76%).