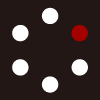
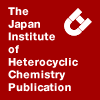
HETEROCYCLES
An International Journal for Reviews and Communications in Heterocyclic ChemistryWeb Edition ISSN: 1881-0942
Published online by The Japan Institute of Heterocyclic Chemistry
e-Journal
Full Text HTML
Received, 25th July, 2013, Accepted, 19th September, 2013, Published online, 30th September, 2013.
DOI: 10.3987/COM-13-S(S)95
■ No Catalyst, Radical Initiator and Photochemical Irradiation Approach to Direct α-Trifluoromethylation of Lithium Enolates
Yuichi Tomita, Toshiaki Iida, Ryota Hashimoto, Yoshimitsu Itoh, and Koichi Mikami*
Department of Applied Chemistry, Graduate School of Science and Technology, Tokyo Institute of Technology, 2-12-1 Ookayama, Meguro-ku, Tokyo 152-8552, Japan
Abstract
Heterocyclic lactam lithium enolates exploit an access to the electrophilic radical α-trifluoromethylation without catalyst/radical initiator/photochemical irradiation.Organofluorine compounds have attracted current interest because of their important applications in material1 and pharmaceutical2 sciences. Fluoromethyl compounds are employed as potent analogues with remarkable physical properties and high physiological activity. Particularly, trifluoromethyl (CF3) groups significantly influence the lipophilicity, membrane permeability, aqueous solubility, and metabolic stability of hydrocarbon leads.3 Therefore, the development of synthetic methods to introduce the CF3 substituent at the α-position of carbonyl compounds has emerged as a central issue in modern organofluorine chemistry.4 However, only few methods have been reported for the trifluoromethylation of metal enolates and enolate equivalents, which are widely employed in organic synthesis.5 Simple and versatile alkylation methods of metal enolates with non-fluorinated alkyl halides are generally not applicable to trifluoromethylation with trifluoromethyl halides, because of the negatively rather than positively polarized trifluoromethyl carbon.6 Recently, a radical initiator (Et3B/O2),7 photochemical irradiation,8-10 and Pd-,11 or Rh-catalyzed12 methods have been reported for the trifluoromethylation of metal enolates and their equivalents. In order to solve the problem of α-trifluoromethylation of enolates with trifluoromethyl halides, we report here a direct and no catalyst/radical initiator/photochemical irradiation approach to the α-trifluoromethylation of carbonyl compounds through modification of our recently reported α-difluoroiodomethylation of lactam lithium enolates13 (Scheme 1).
The direct α-trifluoromethylation of metal enolates derived from carbonyl compounds (1) was first scrutinized on the alkaline metal involving Li+, Na+, and K+ as an enolate cation (Scheme 2). Lithium enolates generated using lithium hexamethyldisilazide (LHMDS) could be employed as the metal enolate source for the direct α-trifluoromethylation (entry 1 and 2). However, LDA did not provide the α-trifluoromethylation product (2a) (entry 5). Unfortunately, sodium and potassium hexamethyldisilazide (NHMDS and KHMDS) gave only poor yields of the α-trifluoromethylation product (2a) (entry 3 and 4). Using LHMDS to generate the lithium enolate, the α-trifluoromethylation product (2a) was obtained in the highest (92%) yield (entry 1). Even when 12-crown-4 was added to the lithium enolate, 51% yield of the α-trifluoromethylation product was obtained (entry 2). In sharp contrast to α-difluoroiodomethylation of lithium enolates,13 the present α-trifluoromethylation reaction was not prohibited by the salt out operation of the lithium cation by the use of 12-crown-4.
Several solvents such as THF, DME, MTBE (methyl tert-butyl ether), Et2O and less polar toluene were examined at -78 ˚C for 4 h (Scheme 3). MTBE, DME, and Et2O gave moderate yields (entry 2, 3, 5). Less polar solvent such as toluene gave only low yiled of the α-trifluoromethylation product (2a) along with the α-difluoroiodomethylation product (3a). Amongst the ethereal solvents thus examined, THF gave the best (92%) yield of the α-trifluoromethylation product (2a) (enrty 2).
The effect of lithium amide source was the key in the present α-trifluoromethylation (Scheme 4). Furthermore, the molarity of lithium amides affects the ratio of the α-trifluoromethylation product (2)/the α-difluoroiodomethylation product (3). One equivalent of the lithium amide selectively leads to the formation of the α-trifluoromethylation product (2) except for the case of LDA (entry 1 and 3 vs. 5). Intriguingly, two equivalents of LTMP provided only α-difluoroiodomethylation product (3) in 70% isolated yield without formation of the α-trifluoromethylation product (2) (entry 4). The result suggests that more electron donating and less sterically demanding dialkyl amides such as LTMP provide tight mixed aggregate with the lithium enolates14 than the less electron donating and more sterically demanding bis(silyl) amides such as LHMDS.
In view of the asymmetric photocatalytic perfluoroalkylation of aldehydes reported by MacMillan,9 the effect of photochemical irradiation was further investigated on the present no catalyst/radical initiator trifluoromethylation of lithium enolates (Scheme 5). However with or without photochemical irradiation, Boc-protected lactam substrates afforded a similar level of good yields for the α-trifluoromethylation products (2) up to 92% yields.
The following reaction mechanism could involve in the present trifluoromethylation of lithium enolates with trifluoromethyl iodide that should be different from our previous mechanistic rationale for the α-difluoromethylation of lithium enolates with the same trifluoromethyl iodide via Li–F interaction.13 Single electron transfer (SET) to trifluoromethyl iodide8-10,16 from the lithium enolates would be operative to generate the electrophilic trifluoromethyl radical via abstraction of iodide with Lewis acidic lithium cation (Scheme 6).8e Through addition of the trifluoromethyl radical to the lithium enolates, a ketyl radical intermediate would be generated and then react with another trifluoromethyl iodide molecule to produce the trifluoromethyl radical along with the formation of the α-trifluoromethyl product (2) and lithium iodide.8e
The reaction conditions were optimized to construct even sterically congested quaternary17 carbon centers (Table 1). The electrophilic radical trifluoromethylation shows a substrate applicability that exhibits electronic and steric scope. In Boc-protected six-membered lactam (1a) gave the α-trifluoromethylation product (2a) in 92% yield. In five-membered lactam, the α-trifluoromethylation product (2b) was specifically obtained along with high (92%) chemical yield in a similar manner to the six-membered lactam. A relatively electron-donating Boc protecting group thus leads to the high yields for the α-trifluoromethylation product (2), because of the facile SET from the electron rich Boc-protected enolates. Cbz-protected lactams (1c and d) also gave a similar but slightly lower level of chemical yields for the α-trifluoromethylation products (2c and d) up to 61% yield. In contrast, a relatively electron-withdrawing benzoyl-protecting group (1e) provides low yield for the α-trifluoromethylation product (2e) in 18% yield.
In conclusion, this novel methodology exploits an access to electrophilic radical trifluoromethylation without catalyst/radical initiator/photochemical irradiation to devise a direct approach to the trifluoromethylation of heterocyclic lactam lithium enolates. Fortunately, high levels of trifluoromethylation efficiency could be established for these substrates to afford the desired products with good overall efficiency. This novel methodology is a compliment to MacMillan’s photocatalytic perfluoroalkyl radical approach to aldehydes. Further investigation on our asymmetric version is now under way for wide substrate scope.
References
1. Reviews: (a) P. Kirsch, Modern Fluoroorganic Chemistry, Wiley-VCH, Weinheim, 2013; CrossRef (b) T. Hiyama, K. Kanie, T. Kusumoto, Y. Morizawa, and M. Shimizu, Organofluorine Compounds: Chemistry and Applications, Springer, Berlin, 2000; CrossRef (c) K. Uneyama, Organofluorine Chemistry, Wiley-Blackwell, Oxford, 2006; (d) R. D. Chambers, Fluorine in Organic Chemistry, Wiley-Blackwell, Oxford, 2004.
2. Reviews: (a) I. Ojima, Ed. Fluorine in Medicinal Chemistry and Chemical Biology, Blackwell, Oxford, 2009; (b) J.-P. Begue and D. Bonnet-Delpon, Bioorganic and Medicinal Chemistry of Fluorine, Wiley, Hoboken, 2008; (c) V. A. Soloshonok, K. Mikami, T. Yamazaki, J. T. Welch, and J. F. Honek, Current Fluoroorganic Chemistry, ACS Symp. Series 949, 2006.
3. Reviews: (a) S. Purser, P. R. Moore, S. Swallow, and V. Gouverneur, Chem. Soc. Rev., 2008, 37, 320; CrossRef (b) K. L. Kirk, Org. Process Res. Dev., 2008, 12, 305; CrossRef (c) K. Muller, C. Faeh, and F. Diederich, Science, 2007, 317, 1881. CrossRef
4. Reviews: (a) J. Nie, H.-C. Guo, D. Cahard, and J.-A. Ma, Chem. Rev., 2011, 111, 455; CrossRef (b) D. Cahard and J.-A. Ma, J. Fluorine Chem., 2007, 128, 975; CrossRef (c) D. Cahard and J.-A. Ma, Chem. Rev., 2004, 104, 6119; CrossRef (d) K. Mikami, Y. Itoh, and M. Yamanaka, Chem. Rev., 2004, 104, 1. CrossRef
5. Review on catalytic enantioselective aldol reactions: E. M. Carreira, A. Fettes, and C. Marti, Org. React., 2006, 67, 1.
6. Reviews: (a) J. E. Huheey, J. Phys. Chem., 1965, 69, 3284; CrossRef (b) M. Yoshida, N. Kamigata, H. Sawada, and M. Nakayama, J. Fluorine Chem., 1990, 49, 1; CrossRef (c) D. O’Hagan, Chem. Soc. Rev., 2008, 37, 645. CrossRef
7. For examples: (a) K. Miura, M. Taniguchi, K. Nozaki, K. Oshima, and K. Utimoto, Tetrahedron Lett., 1990, 31, 6391; CrossRef (b) K. Miura, Y. Takeyama, K. Oshima, and K. Utimoto, Bull. Chem. Soc. Jpn., 1991, 64, 1542; CrossRef (c) K. Iseki, T. Nagai, and Y. Kobayashi, Tetrahedron: Asymmetry, 1994, 5, 961; CrossRef (d) J.-A. Ma and D. Cahard, J. Org. Chem., 2003, 68, 8726; CrossRef (e) Y. Itoh and K. Mikami, Org. Lett., 2005, 7, 649; CrossRef (f) Y. Itoh and K. Mikami, Org. Lett., 2005, 7, 4883; CrossRef (g) Y. Itoh and K. Mikami, Tetrahedron, 2006, 62, 7199, and references cited therein. CrossRef
8. Single electron transfer from ketone-derived pyrrolidine enamines to trifluoromethyl iodide has been proposed: (a) D. Cantacuzene and R. Dorme, Tetrahedron Lett., 1975, 16, 2031; CrossRef (b) D. Cantacuzene, C. Wakselman, and R. Dorme, J. Chem. Soc., Perkin Trans. 1, 1977, 1365; CrossRef (c) I. Rico, D. Cantacuzene, and C. Wakselman, Tetrahedron Lett., 1981, 22, 3405; CrossRef (d) C. Wakselman, J. Fluorine Chem., 1992, 59, 367; CrossRef For leading references on single electron transfer from ketone-derived metal enolates and their derivatives to trifluoromethyl iodide: (e) Y. Itoh, K. N. Houk, and K. Mikami, J. Org. Chem., 2006, 71, 8918 and references cited therein. CrossRef
9. (a) D. A. Nicewicz and D. W. C. MacMillan, Science, 2008, 322, 77; CrossRef (b) D. A. Nagib, M. E. Scott, and D. W. C. MacMillan, J. Am. Chem. Soc., 2009, 131, 10875; CrossRef (c) H.-W. Shih, M. N. Vander Wal, R. L. Grange, and D. W. C. MacMillan, J. Am. Chem. Soc., 2010, 132, 13600; CrossRef (d) P. V. Pham, D. A. Nagib, and D. W. C. MacMillan, Angew. Chem. Int. Ed., 2011, 50, 6119. CrossRef
10. For reviews on photoredox catalysis, see: (a) T. P. Yoon, M. A. Ischay, and J. Du, Nature Chem., 2010, 2, 527; CrossRef (b) J. M. R. Narayanam and C. R. J. Stephenson, Chem. Soc. Rev., 2011, 40, 102. CrossRef
11. T. Ishihara, M. Kuroboshi, and Y. Okada, Chem. Lett., 1986, 1895.
12. [RhCl(PPh3)3]/Et2Zn: (a) K. Sato, A. Tarui, M. Omote, A. Ando, and I. Kumadaki, Synthesis, 2010, 1865; CrossRef (b) K. Sato, T. Yuki, R. Yamaguchi, T. Hamano, A. Tarui, M. Omote, I. Kumadaki, and A. Ando, J. Org. Chem., 2009, 74, 3815; CrossRef (c) K. Sato, T. Yuki, A. Tarui, I. Kumadaki, and A. Ando, Tetrahedron Lett., 2008, 49, 3558; CrossRef (d) K. Sato, T. Yuki, R. Yamaguchi, T. Hamano, A. Tarui, M. Omote, I. Kumadaki, and A. Ando, J. Fluorine Chem., 2008, 129, 51; CrossRef (e) Y. Tomita, Y. Itoh, and K. Mikami, Chem. Lett., 2008, 1080; CrossRef 86th Annual Meeting of Chem. Soc. Jpn., 1J2-16, March 2006.
13. K. Mikami, Y. Tomita, and Y. Itoh, Angew. Chem. Int. Ed., 2010, 49, 3819. CrossRef
14. (a) B. L. Lucht and D. B. Collum, Acc. Chem. Res., 1999, 32, 1035; CrossRef (b) D. B. Collum, A. J. McNeil, and A. Ramirez, Angew. Chem. Int. Ed., 2007, 46, 3002; CrossRef (c) V. Snieckus, Chem. Rev., 1990, 90, 879; CrossRef (d) D. Seebach, Angew. Chem., Int. Ed. Engl., 1988, 27, 1624, and references therein. CrossRef
15. Li–F interaction plays an important role in regioselective deprotonation: M. Schlosser, Ed. Organometallics in Synthesis-A Manual, Wiley, Chichester, 1994, p. 1. CrossRef
16. (a) C. R. Burkholder, W. R. Dolbier, Jr., and M. Medebielle, J. Org. Chem., 1998, 63, 5385; CrossRef (b) C. R. Burkholder, W. R. Dolbier, Jr., and M. Medebielle, J. Fluorine Chem., 2001, 109, 39; CrossRef (c) S. A.-Mohand, N. Takeuchi, M. Medebielle, and W. R. Dolbier, Jr., Org. Lett., 2001, 3, 4271; (d) V. A. Petrov, Tetrahedron Lett., 2001, 42, 3267. CrossRef
17. Leading review on the construction of quaternary carbon centers: (a) M. Shimizu, Angew. Chem. Int. Ed., 2011, 50, 5998; CrossRef (b) For a recent example: K. Kikushima, J. C. Holder, M. Gatti, and B. M. Stoltz, J. Am. Chem. Soc., 2011, 133, 6902. CrossRef