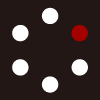
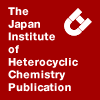
HETEROCYCLES
An International Journal for Reviews and Communications in Heterocyclic ChemistryWeb Edition ISSN: 1881-0942
Published online by The Japan Institute of Heterocyclic Chemistry
e-Journal
Full Text HTML
Received, 29th July, 2013, Accepted, 21st August, 2013, Published online, 27th August, 2013.
DOI: 10.3987/COM-13-S(S)98
■ Rare Earth Triflates/Chlorotrimethylsilane-Promoted One-Pot Synthesis of Enals
Natsuko Kagawa, Yoshiko Sasaki, Shoko Sakaguchi, Ayumi Nagatomo, Hideo Kojima, and Masahiro Toyota*
Team TOYOTA, Graduate School of Science, Osaka Prefecture University, 1-2 Gakuencho, Sakai, Osaka 593-8531, Japan
Abstract
Some heteroaromatic aldehydes were subjected to conditions promoting the rare earth triflates/chlorotrimethylsilane-promoted one-pot synthesis of enals. As a result, diverse α,β-unsaturated aldehydes were obtained in moderate yields.Recently, a wide variety of nucleophilic heterocyclic carbene-catalyzed reactions have been developed, many of which use enals as substrates.1 Therefore, the development of efficient synthetic methodologies to generate diverse enals has received a great deal of attention.2 In this regard, we developed a novel rare earth triflate/chlorotrimethylsilane-promoted one-pot preparation of enals as an alternative protocol for enal synthesis (Scheme 1).3 In this case, triethylamine could be doubly activated by chlorotrimethylsilane and various rare earth lanthanide triflates to become a protected acetaldehyde anion equivalent.
We have continued to improve this one-pot synthesis of enals with the goal of increasing the diversity of possible substrates. In this paper we report some notable results from this investigation. The scope of this one-pot process was assessed with some commercially available heteroaromatic aldehydes 3, and the results are summarized in Table 1. As the data in Table 1 show, all heteroaromatic aldehydes 3 provided desired enals 4 in moderate yields.
When this reaction was performed with benzo[d][1,3]dioxole-5-carbaldehyde (3a) in the presence of a stoichiometric amount of Yb(OTf)3, (E)-3-(benzo[d][1,3]dioxol-5-yl)acrylaldehyde (4a) was isolated in 58% yield (entry 1). In contrast to furan-2-carbaldehyde (1), the reaction with furan-3-caraldehyde (3b) led to a decreased yield (49%) of the homologated product 4b (entry 2). This process is also adaptable to more functionalized aldehydes, such as 3c. (E)-3-(5-(2-Chloro-5-(trifluoromethyl)phenyl)furan-2-yl)-acrylaldehyde (4c) was provided in 59% yield in the presence of 50 mol % of Sc(OTf)3 (entry 3). Thiophene-2-carbaldehyde (3d) was transformed into 4d in 51% yield (entry 4). Substrate 3e gave desired product 4e in 54% yield using 50 mol % of In(OTf)3 (entry 5). Although 1H-pyrrole-2-carbaldehyde (3f) was converted to 4f without protection of the nitrogen, 4f was obtained only in 33% yield (entry 6). Substrate 3g, which has a methyl substituent on nitrogen, provided desired product 4g in 57% yield (entry 7). Finally, 1H-indole-2-carbaldehyde (3h) led to 4h in 66% yield (entry 8). As a group, the reactions proceeded smoothly, and the thermodynamically stable E-enals were generated in moderate yields.
At this stage, it is difficult to predict the optimal lanthanide triflate for the preparation of a specific enal. Therefore, it was necessary to take a trial and error approach with each substrate. The requisite amount of lanthanide triflate also varied with the aldehyde substrate.
Further synthetic applications of this process are under investigation.
EXPERIMENTAL
Unless otherwise noted, all reactions were performed in an oven-dried glassware, sealed with a rubber septum under an atmosphere of argon. Anhydrous CH2Cl2 was purchased from Kanto Chemical Co., Inc. Et3N was distilled from CaH2 prior to use. Unless otherwise mentioned, materials were obtained from commercial suppliers and used without further purification. Flash column chromatography was carried out using Cica 60 N (spherical / 40-50 μm) silica gel. Compounds were visualized using an ultraviolet lamp (254 nm) and /or by staining with p-anisaldehyde (in EtOH) or ammonium molybdate (in 10% H2SO4). IR spectra were measured on a SHIMADZU FT-IR 8300 spectrophotometer. 1H NMR spectra were recorded on Varian 400 MR (400 MHz) spectrometer with CHCl3 or MeOH as an internal standard. 13C NMR spectra were recorded on Varian 400 MR (100 MHz) spectrometer with CHCl3 or MeOH as an internal standard. Mass spectra were recorded on Jeol JMS-AX 700 spectrometer.
Rare Earth Triflates/Chlorotrimethylsilane Promoted One-Pot Synthesis. (E)-3-(Benzo[d][1,3]dioxol-5yl)acrylaldehyde (4a)4 (as a Typical Precedure: run 1). To a suspension of Yb(OTf)3 (207 mg, 0.334 mmol) and TMSCl (0.850 mL, 6.70 mmol) in CH2Cl2 (4 mL) was added dropwise a solution of aldehyde 3a (50.1 mg, 0.330 mmol) and Et3N (1.86 mL, 13.3 mmol) in CH2Cl2 (1 mL) at 0 °C. The mixture was stirred at room temperature for 24 h. Saturated aqueous NaHCO3 solution was added at 0 °C, and then the resulting mixture was stirred at room temperature for 30 min. After separation, the aqueous layer was extracted with CH2Cl2. The combined organic layers were washed with brine and dried over MgSO4. After removal of the solvent, the residue was chromatographed. Elution with a 2:1 mixture of hexane-EtOAc furnished 4a4 (34.1 mg, 58%) as a color less oil. 1H NMR (400 MHz, CDCl3) δ 9.65 (1H, d, J=7.8 Hz), 7.38 (1H, d, J=15.8 Hz), 7.09-7.05 (2H, m), 8.88-6.84 (1H, m), 6.56 (1H, dd, J=15.6 and 7.8 Hz) and 6.04 (2H, s).
(E)-3-(Furan-3-yl)acrylaldehyde (4b).5 1H NMR (400 Mz, CDCl3) δ 9.63 (1H, d, J=8.0 Hz), 7.76 (1H, br s), 7.48 (1H, br s), 7.40 (1H, d, J=15.6 Hz), 6.33 (1H, br s) and 6.45 (1H, dd, J=15.6 and 8.0 Hz).
(E)-3-(5-(2-Chloro-5-(trifluoromethyl)phenyl)furan-2-yl)acrylaldehyde (4c). IR (neat): 1684 cm-1; 1H NMR (400 MHz, CDCl3) δ 9.68 (1H, d, J=7.8 Hz), 8.17-8.12 (1H , m), 7.59 (1H, d, J=7.8 Hz), 7.53-7.47 (1H, m), 7.34 (1H, d, J=3.7 Hz), 7.27 (1H, d, J=15.7 Hz), 6.91 (1H, d, J=3.7 Hz) and 6.73 (1H, dd, J=15.7 and 7.8 Hz); 13C NMR (100 MHz, CDCl3) δ 192.6, 151.7, 150.4, 136.7, 134.1, 131.6, 130.0, 129.7, 128.7, 126.9,125.5, 125.1, 118.4 and 114.9. LRMS m/z: 300 (M+); HRMS m/z: Calcd for C14H8ClF3O2: 300.0165. Found: 300.0165.
(E)-3-(Thiopen-2-yl)acrylaldehyde (4d).5 1H NMR (400 MHz, CDCl3) δ 9.63 (1H, d, J=7.7 Hz), 7.59 (1H, d, J=15.6 Hz), 7.51 (1H, d, J=5.0 Hz), 7.36 (1H, J=3.2 Hz), 7.14-7.09 (1H, m) and 6.52 (1H, dd, J=15.6 and 7.7 Hz).
(E)-3-(Benzo[b]thiophen-3-yl)acrylaldehyde (4e). IR (neat) 1672 cm-1; 1H NMR (400 MHz, CDCl3) δ 9.74 (1H, d, J=7.7 Hz), 8.02 (1H, d, J=8.1 Hz), 7.94-7.89 (2H, m), 7.76 (1H, d, J=16.1 Hz), 7.54-7.42 (2H, m) and 6.84 (1H, dd, J=16.0 and 7.7 Hz); 13C NMR (100 MHz, CDCl3) δ 193.8, 143.9, 140.6, 136.7, 131.4, 130.2, 128.9, 125.4, 125.3, 123.2 and 121.9; LRMS m/z: 188 (M+); HRMS m/z: Calcd for C11H8OS: 188.1295; Found: 188.0296.
(E)-3-(1H-Pyrrol-2-yl)acrylaldehyde (4f).6 1H NMR (400 MHz, CDCl3) δ 9.56 (1H, d, J=7.8 Hz), 8.78 (1H, br s), 7.32 (1H, d, J=15.8 Hz), 7.05 (1H, br s), 6.68 (1H, br s), 6.37-6.33 (1H, m) and 6.32 (1H, dd, J=15.8 and 7.8 Hz).
(E)-3-(1-Methyl-1H-pyrrol-2-yl)acrylaldehyde (4g).5 1H NMR (400 MHz, CDCl3) δ 9.56 (1H, d, J=7.8 Hz), 7.32 (1H, d, J=15.6 Hz), 6.85 (1H, d, J=1.8 Hz), 6.77-6.74 (1H, m), 6.45 (1H, dd, J=15.6 and 7.8 Hz), 6.25-6.22 (1H, m) and 3.76 (3H, s).
(E)-3-(1H-Indol-3-yl)acrylaldehyde (4h).7 1H NMR (400 MHz, CD3OD) δ 9.43 (1H, d, J=8.0 Hz), 7.82-7.78 (2H, m), 7.73 (1H, br s), 7.44-7.41 (1H, m), 7.24-7.15 (2H, m) and 6.67 (1H, dd, J=15.4 and 8.0 Hz).
References
1. A selected review: V. Nair, R. S. Menon, A. T. Biju, C. R. Sinu, R. R. Paul, A. Jose, and V. Sreekumar, Chem. Soc. Rev., 2011, 40, 5336; CrossRef Some recent examples: (a) T. Li, J. Zhu, D. Wu, X. Li, S. Wang, H. Li, J. Li, and W. Wang, Chem. Eur. J., 2013, 19, 9147; CrossRef (b) N. A. White, D. D. DiRocco, and T. Rovis, J. Am. Chem. Soc., 2013, 135, 8504; CrossRef (c) W. W. Y. Leong, X. Chen, and Y. R. Chi, Green Chem., 2013, 15, 1505; CrossRef Some representative reaction examples of aryl and/or heteroaryl enals: (a) R. Infante, Y. Hernandez, J. Nieto, and C. Andres, Eur. J. Org. Chem., 2013, 4863; CrossRef (b) V. Gupta, S. Sudhir V., T. Mandal, and C. Schneider, Angew. Chem. Int. Ed., 2012, 51, 12609; CrossRef (c) W. Li, X. Li, T. Ye, W. Wu, X. Liang, and J. Ye, Tetrahedron Lett., 2011, 52, 2715; CrossRef (d) X. Luo, Z. Zhou, F. Yu, X. Li, X. Liang, and J. Ye, Chem. Lett., 2011, 40, 518; CrossRef (e) Z. Chen, X. Yu, and J. Wu, Chem. Commun., 2010, 46, 6356. CrossRef
2. One-step synthetic methodologies for enals: (a) P. Valenta, N. A. Drucker, J. W. Bode, and P. J. Walsh, Org. Lett., 2009, 11, 2117; CrossRef (b) P. K. Mahata, O. Barun, H. Ila, and H. Junjappa, Synlett, 2000, 1345; CrossRef (c) L. Duhamel, J. Gralak, and B. Ngono, J. Organomet. Chem., 1989, 363, C4; CrossRef (d) C. W. Spanglar and R. K. McCoy, Synth. Commun., 1988, 18, 51; CrossRef (e) Y. Huang, L. Shi, and J. Yang, Tetrahedron Lett., 1985, 26, 6447; CrossRef (f) T. Hirao, Y. Fujihara, S. Tsuno, Y. Ohshiro, and T. Agawa, Chem. Lett., 1984, 13, 367; CrossRef (g) H. J. Bestmann, K. Roth, and M. Ettlinger, Chem. Ber., 1982, 115, 161; CrossRef (h) S. F. Martin, Synthesis, 1979, 633; CrossRef E. J. Corey, D. Enders, and M. G. Bock, Tetrahedron Lett., 1976, 17, 7. CrossRef
3. N. Kagawa, Y. Sasaki, H. Kojima, and M. Toyota, Tetrahedron Lett., 2010, 51, 482. CrossRef
4. T. Yamada, S. Sakaguchi, and Y. Ishii, J. Org. Chem., 2005, 70, 5471. CrossRef
5. K. Lu, T. Luo, Z. Xiang, Z. You, R. Fathi, J. Chen, and Z. Yang, J. Comb. Chem., 2005, 7, 958. CrossRef
6. Y. Badar, W. J. S. Lockley, T. P. Toube, B. C. L. Weedon, and L. R. Guy Valadon, J. Chem. Soc., Perkin Trams. 1, 1973, 1416. CrossRef
7. S.-K. Xiang, B. Zhang, L.-H. Zhang, Y. Cui, and N. Jiao, Chem. Commun., 2011, 47, 8097. CrossRef