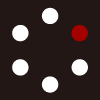
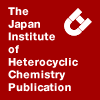
HETEROCYCLES
An International Journal for Reviews and Communications in Heterocyclic ChemistryWeb Edition ISSN: 1881-0942
Published online by The Japan Institute of Heterocyclic Chemistry
e-Journal
Full Text HTML
Received, 5th June, 2013, Accepted, 8th July, 2013, Published online, 16th July, 2013.
DOI: 10.3987/REV-13-SR(S)2
■ Additive Effects on Asymmetric Hydrogenation of N-Heteroaromatics
Takuto Nagano, Atsuhiro Iimuro, Kenta Yamaji, Yusuke Kita, and Kazushi Mashima*
Graduate School of Engineering Science, Osaka University, 1-3 Machikaneyama, Toyonaka, Osaka 560-8531, Japan
Abstract
The multiplication of chiral information is one of the most important tasks in organic chemistry. Chiral catalysis is the most elegant way to achieve this and organic chemists developed a variety of catalytic asymmetric reaction systems, in which the addition of some chemicals effectively improves the performance of transition-metal-catalysis. In this review, we summarize development of additive effects in the asymmetric hydrogenation of N-heteroaromatic compounds based on interactions of additives with catalysts and substrates.1. INTRODUCTION
Optically active amines are ubiquitous functional motifs abundant in many biologically active compounds, and efficient synthetic methods are in high demand for pharmaceutical, agricultural, and fine chemical applications. The homogeneous transition-metal-catalyzed asymmetric hydrogenation of N-heteroaromatic compounds as well as imines, providing chiral cyclic and acyclic amines, are some of the most straightforward atom-economical synthetic protocols. Although there has been remarkable development of homogeneous asymmetric hydrogenation over the last few decades, e.g., chiral complexes of late-transition-metals, such as rhodium, ruthenium, and iridium are highly effective for the asymmetric hydrogenation of various unsaturated bonds such as C=C (favored by Rh species), C=O (favored by Ru species), and C=N bonds (favored by Ir species),1 enantioselective reduction of heteroaromatic compounds has been considered a difficult task presumably because: 1) heteroaromatic compounds are highly stable due to their aromaticity, and thus relatively harsh conditions are needed; 2) heteroatoms involved in the aromatic ring, especially the nitrogen atom, have high coordination ability for catalytic active metals to deactivate or retard the catalytic reaction; and 3) the absence of proximate functional groups that work as directing groups toward a metal center results in low catalytic activity and low enantioselectivity (Scheme 1).2
The addition of some chemicals improves the catalytic performance. Suitable additives drastically increase not only the catalytic activity but also the enantiomeric excess of chiral products. In fact, catalytic activity and enantioselectivity for asymmetric hydrogenation of imines are effectively enhanced by various additives, such as iodine,3,4 iodide salts,5-8 imides,9 benzylamines,10,11 sulfonate salts,12 silver salts,13 TsCl,14 (Boc)2O,15 Brønsted acids16 or bases17 (Scheme 2), and some of these additives have been applied to the asymmetric hydrogenation of N-heteroaromatic compounds to afford chiral cyclic amine products with high yield and high enantioselectivity. Although the precise functions of these additives are not clear in many cases, some mechanisms have been speculated; e.g., additives act to (1) prevent the aggregation of catalytic active species, (2) accelerate catalytic cycle by exchanging the product by substrate
at the metal center, (3) coordinate to the metal center to make the catalyst more active and selective, (4) tune the electronic properties, and (5) generate catalytic active species by oxidizing catalytic metal precursors.18 The asymmetric hydrogenation of imines and heteroaromatic compounds was recently reviewed by Kuwano,19 Kalck,20 Claver,21 Zhou,2 and Yu.22 In this review, we focus on the recent progress of additive effects in the asymmetric hydrogenation of N-heteroaromatic compounds, along with some typical examples of imine hydrogenations based on interactions with catalysts and substrates.
2. ADDITIVE EFFECTS ON CATALYSTS
2.1 Halide and Halogen
Halides and halide salts are used as potent additives for promoting the asymmetric hydrogenation of imines. The first positive effects of KI were reported for Rh-catalyzed asymmetric hydrogenation of imines by Markó et al. in 1984,5 and later by Kutney et al.6 The efficiency of adding iodine for iridium-catalyzed asymmetric hydrogenation of imines was first noted by Blaser and Spindler, and the addition of I2 to an IrI precursor in the presence of a chiral diphosphine ligand dramatically improves both the catalytic activity and enantioselectivity.7 Based on the clear demonstration by Osborn and co-workers that some IrIII complexes bearing iodo ligands and a chelating diphosphine ligand are active catalysts for the asymmetric hydrogenation of imines, it was assumed that iodine is oxidatively added to the IrI species to generate a catalytically active IrIII species.23 More direct evidence was later reported by Togni et al., who prepared an iodine-bridged dinuclear iridium complex 1 with a chelating diphosphine ligand such as Josiphos-derivatives, and used the complex as a catalyst for asymmetric hydrogenation of imines to achieve a high turnover frequency (TOF) (Scheme 3).3
In association with the remarkable effects of iodine for iridium-assisted asymmetric hydrogenation of imines, Zhou et al. reported a similar advantageous application of iodine with an IrI/chiral diphosphine ligand system in the asymmetric hydrogenation of 2-substituted quinolines 4 (Scheme 4).24 Additive effects of halides were also observed for asymmetric hydrogenation of quinolines,25-27 quinoxalines,25,28 and isoquinolines,29 together with non-asymmetric hydrogenation of quinolines.30
As summarized in Table 1, DBDMH and DCDMH also served as halide sources equal to iodine to give IrIII species that hydrogenated 2-methylquinoline (4a) in an enantioselective manner.31 The same additive was also effective for asymmetric hydrogenation of 3,4-disubsituted isoquinolines.29
Preliminary-prepared IrIII complexes bearing halide ligands were reported to work well without any halide additives for the asymmetric hydrogenation of quinoxalines.32 Thus, the functions of iodine and the other halide salts were ascribed to its oxidation ability to turn IrI species A to IrIII species B as a key step to initiate the reduction of N-heteroaromatic compounds (Scheme 5). In the particular case, iodine was assumed to be a source of HI that accelerated the isomerization of 1,4-hydrogenated quinolines to 1,2-hydrogenated quinolines. Interestingly, mechanical studies and theoretical computations suggested that the coexistence of chloride and iodide ligands on a catalytically active complex was necessary for high reactivity and enantioselectivity.
Additive effect of iodine enabled the asymmetric hydrogenation of pyridine derivatives which was identified as difficult substrate for hydrogenation catalyzed by transition metal complex. When an activated-pyridine derivative 6 was hydrogenated by an IrI/(S)-BINAP catalyst in the presence of I2 or iodide anion (nBu4NI), iodine was a better additive for increasing the catalytic activity (Table 2),33 presumably due to the smooth formation of an active IrIII species by oxidizing an IrI precursor by I2. High enantioselectivity was observed using iridium complex 8 bearing chiral P,N-ligand. Oxidation of cationic IrI complex 8 by I2 afforded a more active and enantioselective catalyst.
The addition of iodine to a system of [IrCl(cod)]2 and (S)-MeO-BIPHEP was also essentially required to achieve high catalytic activity and enantioselectivity for asymmetric hydrogenation of pyridine derivative 9 to give the corresponding tetrahydrogenated products 10 (Scheme 6).34 Interestingly, the carbonyl group and C=C double bond, which conjugated with the carbonyl moiety, were kept intact under the reducing conditions.
Chan et al. reported that the addition of suitable amounts of iodine to IrI/P-PHOS (or IrI/DIFLUPRPHOS) increased enantioselectivity and catalytic activity in the hydrogenation of quinolines 4 with high TOF and turnover number (TON).26,35 This catalytic system was applicable for the reduction of pyridine derivatives 9, 7,8-dihydroquinoline-5(6H)-ones, to afford the corresponding tetrahydrogenated products 10 (Scheme 7). Thus, it was concluded that iodine worked as an oxidant of the Ir(I) precursor to produce the Ir(III) species and partial salt formation of the substrates. The choice of halogen source is important to achieve high efficient and selective catalytic system.
2.2 Amines
The first amine additive effects were reported by Tani and coworkers: the addition of catalytic amounts of primary amines, such as benzylamine, butylamine, and aniline, as well as a secondary amine such as N-methylbenzylamine effectively improved catalytic activity for asymmetric hydrogenation of imines catalyzed by a chiral IrI complex, whereas a tertiary amine N,N-dimethylbenzylamine was not effective.10 Quite recently, Mashima, Ohshima, Togni, and co-workers reported the additive effects of aniline derivatives in asymmetric hydrogenation of 2-substituted quinoxalines catalyzed by a halide-bridged chiral iridium dinuclear complex (S)-11a (Table 3).36 An electron-rich secondary aniline derivative, N-methyl-p-methoxyaniline (abbr. MPA) (pKa = 5.93), was the best additive for enriching both reactivity and enantioselectivity. On the other hand, the addition of large amounts of alkyl amines (pKa around 10) significantly delayed the reaction because such amines coordinated tightly to the iridium center to suppress catalytic activity. Notably, quinolones 9 could be smoothly reduced because a nitrogen atom of the corresponding product attached to C(sp2) and kept the pKa value below 10, while isoquinolines and simpler pyridine substrates could not be readily hydrogenated. Controlled mechanistic studies revealed that the Ir-catalyzed hydrogenation of 12 involved two independent catalytic cycles, where the additional aniline derivative played an important role in converting an initial low active catalytic cycle to the other cycle with high activity and enantioselectivity (Scheme 8). In the initial cycle, the reduction proceeded to give half-reduced quinoxaline derivative 14 followed by acid-catalyzed disproportionation of dihydroquinoxaline 14 to afford the quinoxaline 12 and the product amine (S)-13 with low enantioselectivity, and hence the aniline derivative served as a Brønsted base to suppress the low enantioselective disproportionation by trapping acidic protons. Zhou et al. recently reported a highly enantioselective disproportionation of dihydroquinoxaline to give quinoxaline and chiral tetrahydroquinoxaline that caused by an addition of chiral phosphoric acid.37,38 In another highly active catalytic cycle, additive amines acted as amine/amide ligands to generate highly reactive and enantioselective IrIII dihydride species (S)-15, which catalyzed asymmetric hydrogenation via an outer-sphere mechanism.39 Surprisingly, the chiral product of 2-phenylquinoxaline, which was also a kind of aniline derivative, showed the same additive effects to improve not only the catalytic activity but also the enantioselectivity. Accordingly, the reaction rate and enantioselectivity increased exponentially as the reaction proceeded, demonstrating the first example of positive-feedback enhancement of asymmetric hydrogenation rationalized by the proposed dual mechanism.
Tertiary amines act as simple bases to activate a ruthenium complex bearing a chiral trans-chelating diphosphine ligand PhTRAP, by which five-membered N-heteroaromatic compounds were successfully hydrogenated to give the corresponding cyclic amines in an enantioselective manner. N-Protected substrates, 2,3,5-trisubstituted N-Boc-pyrroles 16, were hydrogenated by a Ru/PhTRAP system activated using Et3N as a base (Scheme 9).40 A similar catalytic system using Et3N and N,N,N,N-tetramethyl- guanidine (TMG) efficiently induced the asymmetric hydrogenation of substituted imidazoles 19 and oxazoles 21 giving the corresponding imidazolines 20 and oxazolines 22 with high enantioselectivity (Scheme 10).41 The function of amine can be classified to two categolies; one is simple base and the other is amine/amide ligand.
2.3 Inorganic Base
Inorganic bases are used to generate active metal-hydride species from H2-coordinated metal complex for asymmetric hydrogenation of simple unsaturated bonds.42 Kuwano et al. used Cs2CO3 to activate rhodium and ruthenium complexes of PhTRAP that catalyzed hydrogenations of various five-membered N-heteroaromatic compounds. The addition of Cs2CO3 was essential for achieving high catalytic reactivity and high enantioselectivity for Rh-catalyzed asymmetric hydrogenation of N-acetyl-2-substituted indoles 23 (Table 4).43 In this reaction, Et3N also worked as a base as seen in previous section. Similarly, asymmetric hydrogenation of N-tosyl-3-substituted indole derivatives 25 was improved by Cs2CO3 to give the corresponding chiral indolines 26 (Scheme 11).44 The use of Cs2CO3 to activate a Ru/PhTRAP system
led to the proficient hydrogenation of 2-substituted or 3-substituted N-Boc-protected indole derivatives 27 to give the corresponding indolines 28 (Scheme 12).45
Agbossou-Niedercorn et al. hydrogenated N-Boc indole derivatives 29 using a Rh/Walphos system in the presence of Cs2CO3 (Scheme 13).46 The addition of Cs2CO3, however, caused unwanted side reactions, such as alcoholysis of Boc-protection and transesterification of the substituent, though the side reactions were diminished by optimizing the reaction conditions.
Feringa and de Vries pointed out the competence of additive bases such as Cs2CO3, CsF, and KOAc, as well as Et3N, for asymmetric hydrogenation of N-protected indoles 31 catalyzed by a rhodium complex bearing monodentate phosphoramidite ligand (S)-PipPhos (Scheme 14).47 N-Boc-protection was not sufficient to gain high enantioselectivity in this system. The role of the base was proposed to trap a proton from a cationic
Rh—(η2-H2) species and generate an active Rh-hydride species (Scheme 15). Overall, all five-membered N-heteroaromatic substrates required a suitable protecting group, which was assumed to act as a directing group, at the nitrogen atom to achieve high reactivity and high enantioselectivity. Henschke et al. reported that the asymmetric hydrogenation of quinoxalines was catalyzed in high yield and moderate enantioselectivity by RuCl2(HexaPHEMP)(diamine) complexes upon activation by tBuOK (Scheme 16),48 which was originally developed by Noyori et al.49
3. Additive Effects on Substrates
3.1 Brønsted acid
Some Brønsted acids interact directly with N-heteroaromatic compounds to reduce or break their aromaticity.22 Enantioselective hydrogenation of 2-substituted quinolines was catalyzed by a modified Noyori-type half-metallocene complex 33 of iridium (Scheme 17), in which the addition of 10 mol% of trifluoroacetic acid (TFA) increased both the reaction rate and enantioselectivity.50 Similar additive effects of Brønsted acid were reported by Fan and co-workers, who used a small amount of TfOH (0.1 mol%) to achieve high enantioselectivity in the asymmetric hydrogenation of quinolines catalyzed by a modified Noyori-type Ru complex 34 (Scheme 18).51 The addition of HCl (10 mol%) to a mixture of [IrCl(cod)]2 and a chiral P-OP ligand became an efficient catalyst for the asymmetric hydrogenation of quinolines and
quinoxalines (Scheme 19).52 Additives such as iodine sources and other Brønsted acids did not produce any notable improvement in this case. Mashima et al. reported asymmetric hydrogenation of 2-substituted quinolinium HX (X = Cl, Br, I) salts 35 and 1- or 3-substituted isoquinolinium HX salts 36 by chiral iridium dinuclear complex (S)-11b (Scheme 20).53 The use of salts of the substrate afforded better enantioselectivity than the corresponding neutral substrates. It was noteworthy that the chloro- and bromo-iridium complexes had higher catalytic activity than an iodo-iridium complex, in contrast to the general tendency of the halide effects, iodide anion being better than others.
The formation of iminium salts caused by the Brønsted acid was essential to activate N-unprotected 5-membered N-heteroaromatic substrates, for which Pd(OCOCF3)2/chiral phosphine became a catalyst. Asymmetric hydrogenation of N-unprotected indoles 38 by a palladium catalyst in the presence of one equivalent of chiral Brønsted acid (L-camphorsulfonic acid; L-CSA), which has matched chirality with chiral diphosphine ligand, was reported by Zhou et al. (Scheme 21).54 Protonation of the C=C bond destroyed the aromaticity of indoles, leading to hydrogenation. Achiral sulfonic acid, EtSO3H, also worked
well for asymmetric hydrogenation of 2,5-disubstituted pyrroles 40 to give the corresponding chiral pyrrolines 41 (Scheme 22).55 Pyrroles were first protonated by a Brønsted acid to form the corresponding iminium salts, which were hydrogenated to give enamines followed by isomerization to cyclic imines. For special substrates, 3-(α-hydroxyalkyl)indoles 42, and 3-(α-aminoalkyl)indoles 43, the first step was acid-catalyzed dehydration or deamidation, which provided vinylogous iminium intermediate 45 prior to be hydrogenated by Pd complex (Scheme 23).56
The addition of a Brønsted acid produced clear additive effects; the HCl salt of piperidine became an activator. Feringa et al. reported asymmetric hydrogenation of quinolines catalyzed by an IrI complex with chiral monodentate phosphine in the presence of the HCl salt of piperidine (Scheme 24),57 and asymmetric hydrogenation of quinoxalines was also efficiently achieved by using the same HCl salt of piperidine (Scheme 25).58 Zhou et al. reported similar positive effects of the TfOH salt of piperidine for the iridium-catalyzed asymmetric hydrogenation of quinolines (Scheme 26).59
3.2 Protecting reagents
Introduction of a protecting group at the nitrogen atom is an effective method for the metal-catalyzed reduction of C=N bonds due to the activation of substrates by electronic tuning and the prevention of catalyst poisoning and retarding by coordination of products although deprotection under harsh conditions was required to obtain free cyclic amines. Asymmetric hydrogenation of N-heteroaromatic compounds such as quinolines and isoquinolines was successfully performed using chloroformates (Scheme 27).60
Asymmetric hydrogenation in the presence of equimolar amounts of chloroformates and one equivalent of base gave the corresponding formates, which were hydrogenated to give the corresponding N-protected tetrahydroquinolines 46 and N-protected 1,2-dihydroisoquinolines 48 with high enantioselectivity. The protecting reagents are assumed to avoid poisoning of the catalyst by reducing coordination ability and to facilitate the control of enantioselectivity by the coordination of the attached CO2R group.
Recently, Zhou et al. demonstrated that asymmetric hydrogenation of N-benzyl-pyridinium salts 49 afforded the corresponding chiral N-benzylated piperidines 50 using the Ir/SYNPHOS system as the catalyst (Scheme 28).61 Similar benzylation strategy could be applied to asymmetric hydrogenation of 1- or 3-substituted isoquinolinium salts 51 using Ir/TunePhos system (Scheme 29).62 The counter anion of the substrate and the bulky substituent on the nitrogen atom played critical roles for achieving high reactivity and enantioselectivity; a bromide anion and a bulky and coordinating substituent were found to be optimal. Similarly, hydrogenation of activated N-iminopyridinium ylides smoothly proceeded to give the corresponding piperidine derivatives (Table 2) (vide supra).
4. Outlook
Hydrogenation of N-heteroaromatic compounds using transition metals has been considered difficult due to the aromatic conjugation of these substrates. In the last decade, however, some additives have been developed that dramatically improve the catalytic performance of iridium complexes, as well as rhodium, ruthenium, and palladium complexes, and the effects of these additives are classified as catalyst activators or substrate activators.
The most important function of catalyst activators such as halides, amines and inorganic bases, is to assist the generation of catalytically active species. Halide additives generate catalytically active IrIII species bearing halide ligands, and the choice of halide ligands on the active species is a critical factor for high reactivity and high enantioselectivity. Some amines act as ligands of catalytically active species that hydrogenate unsaturated bonds via outer-sphere mechanism. Organic and inorganic bases assist the generation of highly active species to improve catalytic activity and selectivity.
The function of substrate activators is to reduce or break the aromaticity of substrate. Six-membered N-heteroaromatic compounds are efficiently activated by the formation of the Brønsted acid salts as well as the introduction of protecting groups on the nitrogen atom of substrates. In the case of five-membered N-heterocyclic compounds, substrates are protonated to be cyclic iminium cations, which are easier to be hydrogenated than aromatic form. Some of protecting groups have an extra function as directing groups or bulky substituents to increase reactivity and enantioselectivity.
In the hydrogenation of N-heteroaromatic compounds, substrates and product amines were regarded as catalytic poisons to decrease catalytic activity and enantioselectivity. A recent report for Ir-catalyzed asymmetric hydrogenations of quinoxalines in the presence of amines clearly demonstrated that the pKa value of the coexisting amines was a sensitive factor for determining whether or not N-heteroaromatic compounds could be efficiently hydrogenated. In other words, generated chiral cyclic amines are not always harmful to the catalytic active species; some catalysts are tolerant for the coexistence of amino group, or chiral amines can even be activators in specific case. Thus, asymmetric hydrogenation of unprotected N-heteroaromatics may be achieved by using robust and well-designed catalysts. The previously reported combinations of substrates and catalytic system were limited to specific patterns (e.g. iridium and halide, or ruthenium/rhodium and base). Therefore, investigation of various combinations of metal and additive would be valuable for exploration of more reactive, selective and unique asymmetric hydrogenations. Furthermore, although such reported additive effects were remarkable in the hydrogenation of stable N-heteroaromatic compounds, more detailed mechanistic investigations are required to gain more insight and systematic understandings for designing practical catalyst systems. We hope this perspective review contributes to the outlook of the attractive research field of additives.
ACKNOWLEDGEMENTS
We appreciate for the financial support by The Core Research for Evolutional Science and Technology (CREST), program of the Japan Science and Technology Agency (JST), and a Grant-in-Aid for Scientific Research on Innovative Areas, “Molecular Activation Directed toward Straightforward Synthesis“, from the Ministry of Education, Culture, Sports, Science and Technology (MEXT), Japan. T.N. and A.I. thank a JSPS Reseach Fellowship.
References
1. R. Noyori, Asymmetric Catalysis in Organic Synthesis, Wiley, New York, 1994, Chapter 2, pp. 16-94; J. M. Brown, R. L. Halterman, T. Ohkuma, R. Noyori, H.-U. Blaser, and F. Spindler in Comprehensive Asymmetric Catalysis, Vol. 1 (ed. by E. N. Jacobsen, A. Pfaltz, and H. Yamamoto), Springer, Berlin, 1999, pp. 122-266; S. J. Roseblade and A. Pfaltz, Acc. Chem. Res., 2007, 40, 1402; CrossRef G. Shang, W. Li, and X. Zhang, in Catalytic Asymmetric Synthesis, 3rd ed. (ed. by I. Ojima), Wiley, Hoboken, 2010, Chapter 7, pp. 343-436; CrossRef D. J. Ager, C. J. Cobley, P. H. Moran, H.-U. Blaser, M. Lotz, F. Spindler, Y. Chi, W. Tang, X. Zhang, S. H. L. Kok, T. T.-L. Au-Yeung, H. Y. Cheung, W. S. Lam, S. S. Chan, A. S. C. Chan, M. van den Berg, B. L. Feringa, A. J. Minnaard, A. Pfaltz, S. Bell, J. M. Brown, T. Ohkuma, R. Noyori, A. Mortreux, A. Karim, A. J. Blacker, J. G. de Vries, L. Lefort, and M. Thommen, in Handbook of Homogeneous Hydrogenation, (ed. by J. G. de Vries, and C. J. Elsevier), Wiely-VCH, Weinheim, 2007, Part 4, pp. 745-1324; K. Püntener, M. Scalone, W. Bonrath, R. Karge, T. Netcher, F. Roessler, and F. Spindler, in Asymmetric Catalysis on Industrial Scale: Challenges, Approaches and Solutions, 2nd ed. (ed. by H. U. Blaser, and H. J. Federsel), Wiley-VCH, Weinheim, 2010. Chapter 2-3, pp. 13-38; CrossRef P. Etayo and A. Vidal-Ferran, Chem. Soc. Rev., 2013, 42, 728. CrossRef
2. D.-S. Wang, Q.-A. Chen, S.-M. Lu, and Y.-G. Zhou, Chem. Rev., 2012, 112, 2557. CrossRef
3. A. Togni, Angew. Chem. Int. Ed., 1996, 35, 1475; CrossRef D. Xiao and X. Zhang, Angew. Chem. Int. Ed., 2001, 40, 3425. CrossRef
4. R. Dorta, D. Broggini, R. Stoop, H. Rüegger, F. Spindler, and A. Togni, Chem. Eur. J., 2004, 10, 267; CrossRef C. Moessner and C. Bolm, Angew. Chem. Int. Ed., 2005, 44, 7564; CrossRef M. Chang, W. Li, and X. Zhang, Angew. Chem. Int. Ed., 2011, 50, 10679; CrossRef K. Gao, C.-B. Yu, D.-S. Wang, and Y.-G. Zhou, Adv. Synth. Catal., 2012, 354, 483. CrossRef
5. S. Vastag, J. Bakos, S. Torös, N. E. Takach, R. B. King, B. Heil, and L. Markó, J. Mol. Catal., 1984, 22, 283. CrossRef
6. G.-J. Kang, W. R. Cullen, M. D. Fryzuk, B. R. James, and J. P. Kutney, J. Chem. Soc., Chem. Commun., 1988, 1466. CrossRef
7. F. Spindler, B. Pugin, and H.-U. Blaser, Angew. Chem. Int. Ed., 1990, 29, 558. CrossRef
8. T. Morimoto, N. Nakajima, and K. Achiwa, Chem. Pharm. Bull., 1994, 42, 1951; CrossRef T. Morimoto, N. Nakajima, and K. Achiwa, Synlett, 1995, 748; CrossRef K. Satoh, M. Inenaga, and K. Kanai, Tetrahedron: Asymmetry, 1998, 9, 2657; CrossRef B. Pugin, H. Landert, F. Spindler, and H.-U. Blaser, Adv. Synth. Catal., 2002, 344, 974; CrossRef E. Guiu, B. Muñoz, S. Castillón, and C. Claver, Adv. Synth. Catal., 2003, 345, 169; CrossRef J.-H. Xie, P.-C. Yan, Q.-Q. Zhang, K.-X. Yuan, and Q.-L. Zhou, ACS Catal., 2012, 2, 561.
9. T. Morimoto and K. Achiwa, Tetrahedron: Asymmetry, 1995, 6, 2661; CrossRef T. Morimoto, N. Suzuki, and K. Achiwa, Tetrahedron: Asymmetry, 1998, 9, 183; CrossRef G. Zhu and X. Zhang, Tetrahedron: Asymmetry, 1998, 9, 2415. CrossRef
10. K. Tani, J.-i. Onouchi, T. Yamagata, and Y. Kataoka, Chem. Lett., 1995, 24, 955. CrossRef
11. P. Marcazzan, B. O. Patrick, and B. R. James, Organometallics, 2003, 22, 1177. CrossRef
12. J. M. Buriak and J. A. Osborn, Organometallics, 1996, 15, 3161. CrossRef
13. C. Li and J. Xiao, J. Am. Chem. Soc., 2008, 130, 13208; CrossRef S.-y. Shirai, H. Nara, Y. Kayaki, and T. Ikariya, Organometallics, 2009, 28, 802. CrossRef
14. F. Berhal, Z. Wu, Z. Zhang, T. Ayad, and V. Ratovelomanana-Vidal, Org. Lett., 2012, 14, 3308. CrossRef
15. F. Chen, T. Wang, Y. He, Z. Ding, Z. Li, L. Xu, and Q.-H. Fan, Chem. Eur. J., 2011, 17, 1109; CrossRef F. Chen, Z. Ding, J. Qin, T. Wang, Y. He, and Q.-H. Fan, Org. Lett., 2011, 13, 4348; CrossRef F. Chen, Z. Ding, Y. He, J. Qin, T. Wang, and Q.-H. Fan, Tetrahedron, 2012, 68, 5248; CrossRef R. Ikeda and R. Kuwano, Molecules, 2012, 17, 6901. CrossRef
16. C. Li, C. Wang, B. Villa-Marcos, and J. Xiao, J. Am. Chem. Soc., 2008, 130, 14450; CrossRef S. Zhou, S. Fleischer, K. Junge, and M. Beller, Angew. Chem. Int. Ed., 2011, 50, 5120; CrossRef M. Ružič, A. Pečavar, D. Prudič, D. Kralj, C. Scriban, and A. Zanotti-Gerasa, Org. Process Res. Dev., 2012, 16, 1293; CrossRef K. Gao, C.-B. Yu, W. Li, Y.-G, Zhou, and X. Zhang, Chem. Commun., 2011, 47, 7845; CrossRef K. Gao, B. Wu, C.-B. Yu, Q.-A. Chen, Z.-S. Ye, and Y.-G. Zhou, Org. Lett., 2012, 14, 3890. CrossRef
17. K. Abdur-Rashid, A. J. Lough, and R. H. Morris, Organometallics, 2001, 20, 1047; CrossRef C. J. Cobley and J. P. Henschke, Adv. Synth. Catal., 2003, 345, 195; CrossRef X.-b. Jiang, A. J. Minnaard, B. Hessen, B. L. Feringa, A. L. L. Duchateau, J. G. O. Andrien, J. A. F. Boogers, and J. G. de Vries, Org. Lett., 2003, 5, 1503; CrossRef P. Cheruku, T. L. Church, and P. G. Andersson, Chem. Asian J., 2008, 3, 1390; CrossRef K. Kutlescha, T. Irrgang, and R. Kempe, Adv. Synth. Catal., 2010, 352, 3126; CrossRef R. Soni, F. K. Cheung, G. C. Clarkson, J. E. D. Martins, M. A. Graham, and M. Wills, Org. Biomol. Chem., 2011, 9, 3290. CrossRef
18. E. M. Vogl, H. Gröger, and M. Shibasaki, Angew. Chem. Int. Ed., 1999, 38, 1570. CrossRef
19. R. Kuwano, Heterocycles, 2008, 76, 909. CrossRef
20. A. Fabrello, A. Bachelier, M. Urrutigoȉty, and P. Kalck, Coord. Chem. Rev., 2010, 254, 273. CrossRef
21. N. Fleury-Brégeot, V. de la Fuente, S. Castillón, and C. Claver, ChemCatChem, 2010, 2, 1346. CrossRef
22. Z. Yu, W. Jin, and Q. Jiang, Angew. Chem. Int. Ed., 2012, 51, 6060. CrossRef
23. Y. N. C. Chan, D. Meyer, and J. A. Osborn, J. Chem. Soc., Chem. Commun., 1990, 869; CrossRef Y. N. C. Chan and J. A. Osborn, J. Am. Chem. Soc., 1990, 112, 9400. CrossRef
24. W.-B. Wang, S.-M. Lu, P.-Y. Yang, X.-W. Han, and Y.-G. Zhou, J. Am. Chem. Soc., 2003, 125, 10536. CrossRef
25. L. Qiu, F. Y. Kwong, J. Wu, W. H. Lam, S. Chan, W.-Y. Yu, Y.-M. Li, R. Guo, Z. Zhou, and A. S. C. Chan, J. Am. Chem. Soc., 2006, 128, 5955. CrossRef
26. W.-J. Tang, J. Tan, L.-J. Xu, K.-H. Lam, Q.-H. Fan, and A. S. C. Chan, Adv. Synth. Catal., 2010, 352, 1055. CrossRef
27. S.-M. Lu, X.-W. Han, and Y.-G. Zhou, Adv. Synth. Catal., 2004, 346, 909; CrossRef K. H. Lam, L. Xu, L. Feng, Q.-H. Fan, F. L. Lam, W.-h. Lo, and A. S. C. Chan, Adv. Synth. Catal., 2005, 347, 1755; CrossRef L. Xu, K. H. Lam, J. Ji, J. Wu, Q.-H. Fan, W.-H. Lo, and A. S. C. Chan, Chem. Commun., 2005, 1390; CrossRef M. T. Reetz and X. Li, Chem. Commun., 2006, 2159; CrossRef M. Jahjah, M. Alame, S. Pellet-Rostaing, and M. Lemaire, Tetrahedron: Asymmetry, 2007, 18, 2305; CrossRef C. Deport, M. Buchotte, K. Abecassis, H. Tadaoka, T. Ayad, T. Ohshima, J.-P. Genêt, K. Mashima, and V. Ratovelomanana-Vidal, Synlett, 2007, 2743; CrossRef S. H. Chan, K. H. Lam, Y.-M. Li, L. Xu, W. Tang, F. L. Lam, W. H. Lo, W. Y. Yu, Q. Fan, and A. S. C. Chan, Tetrahedron: Asymmetry, 2007, 18, 2625; CrossRef Z.-J. Wang, G.-J. Deng, Y. Li, Y.-M. He, W.-J. Tang, and Q.-H. Fan, Org. Lett., 2007, 9, 1243; CrossRef W.-J. Tang, S.-F. Zhu, L.-J. Xu, Q.-L. Zhou, Q.-H. Fan, H.-F. Zhou, K. Lam, and A. S. C. Chan, Chem. Commun., 2007, 613; CrossRef X.-B. Wang and Y.-G. Zhou, J. Org. Chem., 2008, 73, 5640; CrossRef S.-M. Lu and C. Bolm, Adv. Synth. Catal., 2008, 350, 1101; CrossRef M. Eggenstein, A. Thomas, J. Theuerkauf, G. Franciò, and W. Leitner, Adv. Synth. Catal., 2009, 351, 725; CrossRef F.-R. Gou, W. Li, X. Zhang, and Y.-M. Liang, Adv. Synth. Catal., 2010, 352, 2441; CrossRef M. Rubio and A. Pizzano, Molecules, 2010, 15, 7732; CrossRef D.-S. Wang, J. Zhou, D.-W. Wang, Y.-L. Guo, and Y.-G. Zhou, Tetrahedron Lett., 2010, 51, 525; CrossRef D.-Y. Zhang, D.-S. Wang, M.-C. Wang, C.-B. Yu, K. Gao, and Y.-G. Zhou, Synthesis, 2011, 2796; CrossRef A. M. Maj, I. Suisse, C. Méliet, C. Hardouin, and F. Agbossou-Niedercorn, Tetrahedron Lett., 2012, 53, 4747. CrossRef
28. W. Tang, L. Xu, Q.-H. Fan, J. Wang, B. Fan, Z. Zhou, K.-h. Lam, and A. S. C. Chan, Angew. Chem. Int. Ed., 2009, 48, 9135. CrossRef
29. L. Shi, Z.-S. Ye, L.-L. Cao, R.-N. Guo, Y. Hu, and Y.-G. Zhou, Angew. Chem. Int. Ed., 2012, 51, 8286. CrossRef
30. S.-M. Lu, X.-W. Han, and Y.-G. Zhou, J. Organomet. Chem., 2007, 692, 3065. CrossRef
31. D.-W. Wang, X.-B. Wang, D.-S. Wang, S.-M. Lu, Y.-G. Zhou, and Y.-X. Li, J. Org. Chem., 2009, 74, 2780. CrossRef
32. D. Cartigny, T. Nagano, T. Ayad, J.-P. Genêt, T. Ohshima, K. Mashima, and V. Ratovelomanana-Vidal, Adv. Synth. Catal., 2010, 352, 1886. CrossRef
33. C. Y. Legault and A. B. Charette, J. Am. Chem. Soc., 2005, 127, 8966. CrossRef
34. X.-B. Wang, W. Zeng, and Y.-G. Zhou, Tetrahedron Lett., 2008, 49, 4922. CrossRef
35. W. Tang, Y. Sun, L. Xu, T. Wang, Q. Fan, K.-H. Lam, and A. S. C. Chan, Org. Biomol. Chem., 2010, 8, 3464. CrossRef
36. T. Nagano, A. Iimuro, R. Schwenk, T. Ohshima, Y. Kita, A. Togni, and K. Mashima, Chem. Eur. J., 2012, 18, 11578. CrossRef
37. Q.-A. Chen, D.-S. Wang, Y.-G. Zhou, Y. Duan, H.-J. Fan, Y. Yang, and Z. Zhang, J. Am. Chem. Soc., 2011, 133, 6126. CrossRef
38. For applications of chiral phosphoric acid and chiral phosphoramide to asymmetric transfer hydrogenation of C=N bonds, see M. Rueping, A. P. Antonchick, and T. Theissmann, Angew. Chem. Int. Ed., 2006, 45, 3683; CrossRef M. Rueping and A. P. Antonchick, Angew. Chem. Int. Ed., 2007, 46, 4562; CrossRef M. Rueping, T. Theissmann, S. Raja, and J. W. W. Bats, Adv. Synth. Catal., 2008, 350, 1001; CrossRef M. Rueping and T. Theissmann, Chem. Sci., 2010, 1, 473; CrossRef M. Rueping, E. Sugiono, and F. Schoepke, Synlett, 2010, 852; CrossRef M. Rueping, M. Stoeckel, E. Sugiono, and T. Theissmann, Tetrahedron, 2010, 66, 6565; CrossRef M. Rueping, C. Brinkmann, A. P. Antonchick, and I. Atodiresei, Org. Lett., 2010, 12, 4604; CrossRef M. Rueping, F. Tato, and F. R. Schoepke, Chem. Eur. J., 2010, 16, 2688; CrossRef M. Rueping, T. Theissmann, M. Stoeckel, and A. P. Antonchick, Org. Biomol. Chem., 2011, 9, 6844; CrossRef C. Metallinos, F. Barrett, and S. Xu, Synlett, 2008, 720; CrossRef Q.-S. Guo, D.-M. Du, and J. Xu, Angew. Chem. Int. Ed., 2008, 47, 759. CrossRef
39. R. Noyori and S. Hashiguchi, Acc. Chem. Res., 1997, 30, 97; CrossRef K.-J. Haak, S. Hashiguchi, A. Fujii, T. Ikariya, and R. Noyori, Angew. Chem. Int. Ed., 1997, 36, 285; CrossRef M. Yamakawa, H. Ito, and R. Noyori, J. Am. Chem. Soc., 2000, 122, 1466; CrossRef C. A. Sandoval, T. Ohkuma, K. Muñiz, and R. Noyori, J. Am. Chem. Soc., 2003, 125, 13490; CrossRef S. Gladiali and E. Alberico, Chem. Soc. Rev., 2006, 35, 226. CrossRef
40. R. Kuwano, M. Kashiwabara, M. Ohsumi, and H. Kusano, J. Am. Chem. Soc., 2008, 130, 808. CrossRef
41. R. Kuwano, N. Kameyama, and R. Ikeda, J. Am. Chem. Soc., 2011, 133, 7312. CrossRef
42. N. Arai, N. Utsumi, Y. Matsumoto, K. Murata, K. Tsutsumi, and T. Ohkuma, Adv. Synth. Catal., 2012, 354, 2089. CrossRef
43. R. Kuwano, K. Sato, T. Kurokawa, D. Karube, and Y. Ito, J. Am. Chem. Soc., 2000, 122, 7614. CrossRef
44. R. Kuwano, K. Kaneda, T. Ito, K. Sato, T. Kurokawa, and Y. Ito, Org. Lett., 2004, 6, 2213; CrossRef R. Kuwano, M. Kashiwabara, K. Sato, T. Ito, K. Kaneda, and Y. Ito, Tetrahedron: Asymmetry, 2006, 17, 521. CrossRef
45. R. Kuwano and M. Kashiwabara, Org. Lett., 2006, 8, 2653. CrossRef
46. A. M. Maj, I. Suisse, C. Méliet, and F. Agbossou-Niedercorn, Tetrahedron: Asymmetry, 2010, 21, 2010. CrossRef
47. N. Mršić, T. Jerphagnon, A. J. Minnaard, B. L. Feringa, and J. G. de Vries, Tetrahedron: Asymmetry, 2010, 21, 7. CrossRef
48. C. J. Cobley and J. P. Henschke, Adv. Synth. Catal., 2003, 345, 195; CrossRef J. P. Henschke, M. J. Burk, C. G. Malan, D. Herzberg, J. A. Peterson, A. J. Wildsmith, C. J. Cobley, and G. Casy, Adv. Synth. Catal., 2003, 345, 300. CrossRef
49. T. Ohkuma, H. Ooka, S. Hashiguchi, T. Ikariya, and R. Noyori, J. Am. Chem. Soc., 1995, 117, 2675. CrossRef
50. Z. W. Li, T.-L. Wang, Y.-M. He, Z.-J. Wang, Q.-H. Fan, J. Pan, and L.-J. Xu, Org. Lett., 2008, 10, 5265. CrossRef
51. Z.-J. Wang, H.-F. Zhou, T.-L. Wang, Y.-M. He, and Q.-H. Fan, Green Chem., 2009, 11, 767; CrossRef H. Zhou, Z. Li, Z. Wang, T. Wang, L. Xu, Y. He, Q.-H. Fan, J. Pan, L. Gu, and A. S. C. Chan, Angew. Chem. Int. Ed., 2008, 47, 8464. CrossRef
52. J. L. Nùñez-Rico, H. Fernández-Pérez, J. Benet-Buchholz, and A. Vidal-Ferran, Organometallics, 2010, 29, 6627.
53. H. Tadaoka, D. Cartigny, T. Nagano, T. Gosavi, T. Ayad, J.-P. Genêt, T. Ohshima, V. Ratovelomanana-Vidal, and K. Mashima, Chem. Eur. J., 2009, 15, 9990; CrossRef A. Iimuro, K. Yamaji, S. Kandula, T. Nagano, Y. Kita, and K. Mashima, Angew. Chem. Int. Ed., 2013, 52, 2046. CrossRef
54. D. S. Wang, Q.-A. Chen, W. Li, C.-B. Yu, Y.-G. Zhou, and X. Zhang, J. Am. Chem. Soc., 2010, 132, 8909. CrossRef
55. D.-S. Wang, Z.-S. Ye, Q.-A. Chen, Y.-G. Zhou, C.-B. Yu, H.-J. Fan, and Y. Duan, J. Am. Chem. Soc., 2011, 133, 8866. CrossRef
56. D.-S. Wang, J. Tang, Y.-G. Zhou, M.-W. Chen, C.-B. Yu, Y. Duan, and G.-F. Jiang, Chem. Sci., 2011, 2, 803; CrossRef Y. Duan, M.-W. Chen, Q.-A. Chen, C.-B. Yu, and Y.-G. Zhou, Org. Bimol. Chem., 2012, 10, 1235. CrossRef
57. N. Mršić, L. Lefort, J. A. F. Boogers, A. J. Minnaard, B. L. Feringa, and J. G. de Vries, Adv. Synth. Catal., 2008, 350, 1081. CrossRef
58. N. Mršič, T. Jerphagnon, A. J. Minnaard, B. L. Feringa, and J. G. de Vries, Adv. Synth. Catal., 2009, 351, 2549. CrossRef
59. D.-S. Wang and Y.-G. Zhou, Tetrahedron Lett., 2010, 51, 3014. CrossRef
60. S.-M. Lu, Y.-Q. Wang, X.-W. Han, and Y.-G. Zhou, Angew. Chem. Int. Ed., 2006, 45, 2260. CrossRef
61. Z.-S. Ye, M.-W. Chen, Q.-A. Chen, L. Shi, Y. Duan, and Y.-G. Zhou, Angew. Chem. Int. Ed., 2012, 51, 10181. CrossRef
62. Z.-S. Ye, R.-N. Guo, X.-F. Cai, M.-W. Chen, L. Shi, and Y.-G. Zhou, Angew. Chem. Int. Ed., 2013, 52, 3685. CrossRef