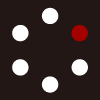
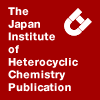
HETEROCYCLES
An International Journal for Reviews and Communications in Heterocyclic ChemistryWeb Edition ISSN: 1881-0942
Published online by The Japan Institute of Heterocyclic Chemistry
e-Journal
Full Text HTML
Received, 30th July, 2013, Accepted, 3rd October, 2013, Published online, 15th October, 2013.
DOI: 10.3987/COM-13-S(S)103
■ Development of a New Synthetic Strategy for Procyanidin Dimer Condensation Using Peracetylated Electrophiles
Sayaka Ishihara, Syoma Doi, Kota Harui, Taisuke Okamoto, Shuhei Okamoto, Joji Uenishi, Takashi Kawasaki, Noriyuki Nakajima,* and Akiko Saito*
Department of Biotechnology, Faculty of Engineering, Toyama Prefectural University, 5180 Kurokawa, Kosugi, Toyama 939-0398, Japan
Abstract
Proanthocyanidins, also known as condensed tannins and/or oligomeric flavonoids, are found in many edible plants and show interesting various biological activities. We report a simple new proanthocyanidin synthesis strategy in which an electrophile derived from a flavan-3-ol peracetate is condensed with 1.5 eq. of a benzylated nucleophile. We demonstrate here the synthesis of procyanidin B1, a (−)-epicatechin-(4β-8)-(+)-catechin dimer, to demonstrate the utility of this method. This strategy is applicable for the synthesis of various commercially available flavan-3-ol and various oligomeric flavonoids.There is currently great interest in the research efforts investigating of compounds that have strong antioxidant activity and superior radical scavenging ability. Furthermore, foods containing active ingredients that can eliminate active oxygen species and free radicals have recently received increased attention. Polyphenols are found in various plants, which are regularly consumed by us. It is widely believed that these polyphenols have a beneficial impact on health, and there are a variety of health foods that contain polyphenols. Furthermore, the scientific investigation of polyphenolic compounds is now increasingly important because of their various strong biological activities. For example, epigallocatechin-3-O-gallate (EGCG), a major polyphenol from green tea, which is a flavan-3-ol, has notably been the focus of intense research for its protective effect against a various types of cancers, including lung, prostate and breast cancers.1 Surface plasmon resonance experiments have demonstrated that the 67-kDa laminin receptor, which is widely expressed at high levels in cancer cell membranes, is an EGCG reseptor2 and is an evidence of EGCG’s selective cytotoxicity against cancer cells.
Proanthocyanidins (condensed tannins or oligomeric flavonoids)3,4 are known to be extremely strong antioxidants; investigating them has become increasingly important because of their various strong biological activities. However, in many cases, proanthocyanidins are obtained as a mixture of various analogs, making purification of each compound difficult. In an elegant contribution, Kozikowski et al.,5 reported the synthesis of oligomeric catechin and epicatechin derivatives.6 We also developed and reported a simple, versatile, and stereoselective method for synthesizing procyanidin oligomers, including (−)-epicatechin (1) and (+)-catechin.7–18 Our synthetic methodology is applicable to variety of procyanidin oligomers, such as 3-O-substituted oligomers. The key step in our method of procyanidin synthesis is the coupling reaction between a nucleophile and a monomeric electrophile using a Lewis acid activator, such as TiCl4, SnCl4 or trimethylsilyl trifluoromethanesulfonate (TMSOTf). We created 2-ethoxyethoxy derivatives on the C-4 position as building blocks for procyanidin synthesis. These condensation reactions proceeded smoothly and afforded a good yield of 4−8 condensed oligomers and stereoselectivity (Scheme 1), but the nucleophile had to be used in four-fold excess to avoid higher oligomer formation. Therefore, we were challenged to develop a new, simple, stereoselective, and regioselective synthetic strategy applicable for the synthesis of a wide-range of procyanidin oligomers. In this report, we describe a new oligomer synthetic strategy that uses a condensation reaction between a peracetyl electrophile and 1.5 equiv. of nucleophiles to give procyanidin dimers (Scheme 2).
A benzyl group is the group most commonly used to protect the phenolic functionalities of procyanidin synthesis. However, the large-scale synthesis of benzyl (+)-catechin and (-)-epicatechin is very limited because of the by-products formation at benzyl-protection.19,20 It is well-known that acetylation of flavan-3-ol and proanthocyanidins proceeds smoothly and affords their peracetates in quantitative yield. The electron-withdrawing acetyl moieties reduce reactivity at the C-8 position of the A-ring. Electrophilic C-4-oxyganated peracetates and C-4-halogenated peracetates have been reported and used for proanthocyanidin synthesis.21 We re-examined the synthesis of peracetylated electrophiles and found that C-4-hydoroxylated electrophile (3) could be obtained from C-4-brominated peracetates by heating with NaHCO3 in DMSO (Scheme 3). One-pot bromination with NBS and hydroxylation with NaHCO3 in DMSO proceeded rapidly at 60 °C and afforded 3 with a yield of 57% yield. This reaction is also applicable to the synthesis of electrophiles derived from (+)-catechin and EGCG in approximately 10%–50% yields (data not shown).
Condensation of 3 with 1.5 eq. of (+)-catechin nucleophile (4a), performed in the presence of SnCl4 at 0 °C afforded dimmer product (5a) with a yield of 33%. When TMSOTf was used as a catalyst, 33% isolation yield was obtained. This condensation method could be also adapted for various nucleophiles (4b-4d) derived from (−)-epicatechin, (−)-epigallocatechin, and (−)- epigallocatechin gallate (EGCG) with yields of 19%, 32%, and 26%, respectively, as shown in Scheme 4. The reaction yield are not so good for the instability of an acetyl group, however, only dimeric procyanidin derivative can be obtained under this reaction conditions without higher oligomer formation.
Because of peak broadening in the NMR spectra, the structure determination of 5a was difficult; therefore, the 3”-hydroxygroup of 5a was acetylated to give acetate 6a in quantitative yield (Scheme 4). We have previously experienced peak broadening in NMR spectra during the synthesis of protection-free procyanidin oligomers that have (−)-epicatehin as an upper unit. Surprisingly, the peak-broadening phenomenon observed with 5a was disappeared upon acetylation of only the 3”-hydroxyl moiety. Furthermore, the structure was ascertained by comparing the spectral data of peracetate 6 with those of synthesized authentic samples. Deprotection of the four benzyl groups of 5a under hydrogenation conditions and acetylation using a general procedure yielded peracetate 7 with a yield of 92% yield (Scheme 5). All spectral data agreed with the structure portrayed in Scheme 5. Deprotection of Ac and Bn group from compound 5a by alkaline hydrolysis with K2CO3, followed by hydrogenation using Pd(OH)2/C under H2 atmosphere was achieved to synthesize procyanidin B1 (8) with 56% yield.
Finally, in initial experiments, to confirm the applicability of this condensation reaction for the synthesis of related molecules, we have confirmed that dimeric procyanidin derivatives can be obtained from condensation reactions between 3 and various nucleophiles (5b-5d) derived from (-)-epicatechin, (−)-epigallocatechin, and (−)-EGCG with yields of 19%, 32%, and 26%, respectively.
EXPERIMENTAL
General: All commercially available chemicals for chemical synthesis were used without further purification. All reactions were performed under an argon atmosphere and monitored by thin-layer chromatography with 0.25-mm pre-coated silica gel plates (60F254 Art 5715; Merck, Darmstadt, Germany). Optical rotation was measured with an ATAGO AP-300 spectrometer. 1H-NMR spectra were recorded on a Varian Inova 500 NMR Spectrometer (500 MHz). Chemical shifts were reported in ppm (d) relative to an internal standard (tetramethylsilane). Fast atom bombardment mass spectra at normal (FABMS) and high resolution (FABHRMS) were obtained using a JEOL JMS-AX500 mass spectrometer. HPLC purification was performed on a Mightysil RP-18 GP column (Kanto Chemical Co. Inc., Japan; 250 x 20 mm, 5 mm) using eluents A [0.05% HCO2H in MeCN/H2O (20/80)] and B (0.05% HCO2H in MeCN). Elution was performed with a linear gradient of 20%–100% B in in A over 20 min (flow rate, 2.0 mL/min).
4-Hydroxy-penta-O-acetyl-(−)-epicatechin (3). A mixture of penta-O-acetyl-(−)-epicatechin (2; 1.00 g, 2.00 mmol) and NBS (0.71 g, 4.00 mmol) in the presence of AIBN (32.8 mg, 0.20 mmol) was refluxed in CH2Cl2–CCl4 (1:4, v/v) for 12 h. NaHCO3 (0.50 g, 5.99 mmol) and DMSO (30 mL) were added to the solution and stirred at 60 °C for 1 h. The reaction mixture was quenched with ice water and extracted with EtOAc. The organic phase was washed with water and brine, and then dried with solid Na2SO4. Filtration, concentration, and silica gel column chromatography (Hex:EtOAc, approximately 7:1−1:3) afforded 0.59 g of 3 as a colorless amorphous powder (1.15 mmol, 57%). [α]D24 + 40.8 (c 0.098, CHCl3); 1H NMR (500 MHz, CDCl3) d = 7.25 (1H, d, J = 8.5 Hz), 7.17 (1H, dd, J = 1.5, 8.5 Hz), 7.14 (1H, d, J = 1.5 Hz), 6.39 (1H, br s), 6.30 (1H, br s), 6.15 (1H, br s), 5.62 (1H, t, J = 4.0 Hz), 5.45 (1H, br s), 2.32 (3H, s), 2.304 (3H, s), 2.299 (3H, s), 2.290 (3H, s), 2.04 (3H, s); the hydroxyl group was not observed. FABMS (m/z) 517 ([M+H]+, 10), 516 (10), 499 (18), 415 (32), 397 (23), 372 (20), 306 (100); FABHRMS calcd for C25H25O12 [M+H]+, 517.1346; found: 517.1371.
[4,8]-2,3-cis-3,4-trans-2”,3”-trans-3,5,7,3’4’-Penta-O-acetyl-5”,7”,3”’,4”’-tetra-O-benzyl-(−)-epicatechin-(+)-catechin (5a). To a solution of 3 (130 mg, 0.25 mmol) and 4a (244 mg, 0.38 mmol) in CH2Cl2 (20 mL) was added dropwise SnCl4 (0.50 mL, 0.50 mmol, 1.0 M solution in CH2Cl2) at 0 °C. After stirring for 5 min, the pale yellow reaction mixture was quenched with saturated aqueous NaHCO3. The mixture was extracted with CHCl3 and the organic phase was washed with water and brine, and then dried with solid Na2SO4. Filtration, concentration, and preparative silica gel TLC purification (hexane:EtOAc, 1:1) afforded a 95 mg of 5a (0.082 mmol, 33%) of as a colorless amorphous powder: [α]D24 + 79.2 (c 0.10, CHCl3); NMR signals from a solution of 5a were quite broad, making peak assignment impossible.
[4,8]-2,3-cis-3,4-trans-2”,3”-trans-3,5,7,3’4’3”-Hexa-O-acetyl-5”,7”,3”’,4”’-tetra-O-benzyl-(−)-epicatechin-(+)-catechin (6a). A solution of 5a (13.1 mg, 0.011 mmol) in CH2Cl2 (5 mL) was acetylated by a general procedure using Ac2O.7-17 After stirring for 30 min at room temperature, the reaction mixture was quenched with water and extracted with CHCl3. The organic phase was washed with water and brine and dried with MgSO4. Filtration, concentration, and chromatography on a short silica gel column (hexane/EtOAc, 1:2) afforded 13.3 mg of 6a (0.011 mmol, 98%) as a colorless amorphous powder: [α]D24 -49.2 (c 0.061, CHCl3); 1H NMR (500 MHz, CDCl3, 0.75:0.75 mixture of rotational isomers) major isomer: 7.47−6.88 (18H, m), 6.84 (0.75H, d, J = 8.0 Hz), 6.69 (0.75H, s), 6.59 (0.75H, d, J = 8.0 Hz), 6.32 (0.75H, s), 6.26 (0.75H, s), 6.26 (0.75H, s), 5.79 (0.75H, s), 5.51 (0.75H, s), 5.38 (0.75H, s), 5.12−4.93 (6H, m), 4.62 (0.75H, s), 4.21 (0.75H, d, J = 9.5 Hz), 3.23 (0.75H, dd, J = 7.0, 17.0 Hz), 2.60 (0.75H, dd, J = 8.5, 17.0 Hz), 2.19-1.95 (13.5H, m); minor isomer: 7.47−6.88 (6.5H, m), 6.49 (0.25H, s), 6.33 (0.25H, s), 6.18 (0.25H, s), 5.36 (0.25H, s), 5.28 (0.25H, s), 5.12−4.93 (2.25H, m), 4.70 (0.25H, d, J = 10.5 Hz), 4.51 (0.25H, s), 4.46 (0.25H, d, J = 10.5 Hz), 3.22−3.18 (0.25H, m), 2.75 (0.25H, dd, J = 8.5, 16.5 Hz), 2.19−1.95 (4.5H, m); 13C NMR (125 MHz, CDCl3) major isomer: 169.8, 169.2, 168.9, 168.4, 168.00, 167.98, 156.5, 155.42, 155.37, 154.2, 148.7, 148.62, 148.59, 148.0, 141.9, 141.6, 137.5, 137.3, 136.9, 136.78, 136.70, 128.7-127.1 (Cx6), 128.7, 128.5, 128.4, 128.3, 127.3, 127.1, 127.0, 124.3, 123.0, 121.8, 120.5, 114.7, 113.6, 113.1, 108.24, 108.18, 106.6, 104.1, 91.1, 78.9, 74.1, 71.3, 71.2, 70.74, 70.66, 70.0, 69.0, 33.2, 27.0, 21.0, 20.8, 20.7, 20.6, 20.5, 20.0; minor isomer: 169.8, 169.2, 169.0, 168.4, 168.1, 168.0, 156.8, 156.4-148.6 (Cx7), 141.8, 141.2, 137.5-136.7 (Cx4), 136.1, 130.0, 128.7-127.1 (Cx6), 128.04, 127.99, 127.8, 127.5, 127.4, 127.2, 124.3, 122.8, 121.7, 120.2, 114.6, 114.0, 113.6, 108.9, 108.2, 107.4, 102.2, 91.9, 78.6, 74.5, 71.7, 71.6, 71.3-70.7 (Cx3), 70.0, 68.7, 29.7, 25.8, 21.2, 21.0-20.5 (Cx3), 20.3, 20.2.
[4,8]-2,3-cis-3,4-trans:2”,3”-trans-Deca-O-acetyl-(−)-epicatechin-(+)-catechin (7). A solution of 5a (7.20 mg, 0.0063 mmol) in 11 mL of THF/MeOH/H2O (20:1:1) was hydrogenated over 20% Pd(OH)2/C (2 mg) for 12 h at room temperature. Filtration and concentration afforded a pale brown solid, which was acetylated using a general procedure to give 5.8 mg of 7 (0.0058 mmol, 92%) as an amorphous solid. All spectral data of 7 agreed with those of the synthetic compound previously reported.11
Procyanidin B1 (8). To a solution of 5a (18.1 mg, 0.016 mmol) in CH2Cl2−MeOH (1:1) was added K2CO3 (13.1 mg, 0.095 mmol) at 0 °C, and the mixture was stirred for 30 min. The reaction mixture was quenched with saturated aqueous NH4Cl solution and extracted with EtOAc. After concentration, the mixture was dissolved with 11 mL of THF:MeOH:H2O (20:1:1) and hydrogenated over 20% Pd(OH)2/C (2 mg) for 3 h at room temperature. Filtration and concentration afforded a pale brown solid that was purified by HPLC purification to give 5.2 mg of pure 8 (0.090 mmol, 56%) as an amorphous solid. All spectral data obtained for 8 agreed with those of the synthesized compound previously reported.11
ACKNOWLEDGEMENTS
This work was supported by BRAIN: Program for Promotion of Basic and Applied Research for Innovations in Bio-oriented Industry (to A. Saito) and JSPS KAKENHI Grant Number 23780123 (Grant-in-Aid for Young Scientist (B) to A. Saito).
References
1. C. S. Yang, P. Maliakai, and X. Meng, Annu. Rev. Pharmacol. Toxicol., 2002, 42, 25. CrossRef
2. H. Tachibana, K. Koga, Y. Fujimura, and K. Yamada, Nat. Struct. Mol. Biol., 2004, 11, 380. CrossRef
3. J. B. Harborne, The Flavonoids: Advances in research from 1986. Chapman and Hall: London, 1993.
4. J. B. Harborne and H. Baxter, The Handbook of Natural Flavonoids, John Wiley & Sons, NY (1999).
5. (a) W. Tuckmantel, A. P. Kozikowski, and L. J. Romanczyk, J. Am. Chem. Soc., 1999, 121, 12073; CrossRef (b) A. P. Kozikowski, W. Tuckmantel, and C. George, J. Org. Chem., 2000, 65, 5371; CrossRef (c) A. P. Kozikowski, W. Tuckmantel, G. Boettcher, and L. J. Romanczyk Jr., J. Org. Chem., 2003, 68, 1641. CrossRef
6. (a) K. Ohmori, N. Ushimaru, and K. Suzuki, Proc. Natl. Acad. Sci. U.S.A., 2004, 101, 12002; CrossRef (b) I. Tarascou, K. Barathieu, Y. Andre, I. Pianet, E. Dufourc, and E. Fouquet, Eur. J. Org. Chem., 2006, 5367; CrossRef (c) P. K. Sharma, A. Kolchinski, H. A. Shea, J. J. Nair, Y. Gou, L. J. Romanczyk Jr., and H. H. Schmitz, Org. Process Res. Dev., 2007, 11, 422; CrossRef (d) Y. Mohri, M. Sagehashi, T. Yamada, Y. Hattori, K. Morimura, T. Kamo, M. Hirota, and H. Makabe, Tetrahedron Lett., 2007, 48, 5891; CrossRef (e) K. Oyama, M. Kuwano, M. Ito, K. Yoshida, and T. Kondo, Tetrahedron Lett., 2008, 49, 3176; CrossRef (f) A. C. Matthew, S. L. Bonnet, and J. H. van der Westhuizen, Org. Lett., 2008, 10, 3865; CrossRef (g) Y. Mohri, M. Sagehashi, T. Yamada, Y. Hattori, Y. Morimura, K. Hamauzu, T. Kamo, M. Hirota, and H. Makabe, Heterocycles, 2009, 79, 549; CrossRef (h) G. Watanabe, K. Ohmori, and K. Suzuki, Chem. Commun., 2013, 5210. CrossRef
7. A. Saito, N. Nakajima, A. Tanaka, and M. Ubukata, Biosci. Biotechnol. Biochem., 2002, 66, 1764. CrossRef
8. A. Saito, N. Nakajima, A. Tanaka, and M. Ubukata, Tetrahedron, 2002, 58, 7829. CrossRef
9. A. Saito, N. Nakajima, A. Tanaka, and M. Ubukata, Tetrahedron Lett., 2003, 44, 5449. CrossRef
10. A. Saito, N. Nakajima, A. Tanaka, and M. Ubukata, Heterocycles, 2003, 61, 287. CrossRef
11. A. Saito, N. Nakajima, M. Matsuura, A. Tanaka, and M. Ubukata, Heterocycles, 2004, 62, 479. CrossRef
12. A. Saito, A. Tanaka, M. Ubukata, and N. Nakajima, Synlett, 2004, 1069. CrossRef
13. A. Saito, A. Tanaka, M. Ubukata, and N. Nakajima, Synlett, 2004, 2040. CrossRef
14. A. Saito, Y. Doi, N. Matsuura, A. Tanaka, M. Ubukata, and N. Nakajima, Bioorg. Med. Chem., 2004, 12, 4783. CrossRef
15. A. Saito, M. Emoto, A. Tanaka, Y. Doi, K. Shoji, Y. Mizushina, H. Ikawa, H. Yoshida, N. Matsuura, and N. Nakajima, Tetrahedron, 2004, 60, 12043. CrossRef
16. A. Saito, Y. Mizushina, H. Ikawa, H. Yoshida, Y. Doi, A. Tanaka, and N. Nakajima, Bioorg. Med. Chem., 2005, 13, 2759. CrossRef
17. H. Sakuda, A. Saito, Y. Mizushina, H. Ikawa, H. Yoshida, A. Tanaka, and N. Nakajima, Heterocycles, 2006, 67, 175. CrossRef
18. A. Saito, Y. Mizushina, A. Tanaka, and N. Nakajima, Tetrahedron, 2009, 65, 7422. CrossRef
19. S. Nakayama, K. Oyama, T. Kondo, and K. Yoshida, Heterocycles, 2007, 73, 451. CrossRef
20. P. K. Sharma, A. Kolchinski, H. A. Shea, J. J. Nair, Y. Gou, L. J. Jr. Romanczyk, and H. H. Schmitz, Org. Process Res. Dev., 2007, 11, 422. CrossRef
21. (a) J. Michaud, J. Masquelier, and A. M. Roudge, Annales Pharmaceutiques Françaises, 1973, 31, 385; (b) J. A. Steenkamp, J. C. S. Malan, and D. Ferreira, J. Chem. Soc., Perkin Trans. 1, 1988, 2179. CrossRef