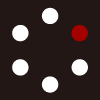
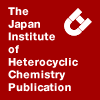
HETEROCYCLES
An International Journal for Reviews and Communications in Heterocyclic ChemistryWeb Edition ISSN: 1881-0942
Published online by The Japan Institute of Heterocyclic Chemistry
e-Journal
Full Text HTML
Received, 21st June, 2013, Accepted, 16th July, 2013, Published online, 23rd July, 2013.
DOI: 10.3987/COM-13-S(S)41
■ Hydrogen Bond Organocatalysis of Benzotriazole in Transamidation of Carboxamides with Amines
Thanh Binh Nguyen,* Ludmila Ermolenko, Marie-Elise Tran Huu Dau, and Ali Al-Mourabit
Department of Chemistry, Institut de Chimie des Substances Naturelles, CNRS, 1, Avenue de la Terrasse, 91190 Gif-sur-Yvette, France
Abstract
A new method of transamidation of carboxamides with amines catalyzed by benzotriazole has been developed.INTRODUCTION
Amide bonds are ubiquitous in living systems and play a key role in bioorganic and medicinal chemistry. Carboxamide group was found to appear in more than 25% of known drugs.1 Amide formation is thus one of the most fundamental reactions in organic synthesis.2 The most common strategy for amide bond formation is the reaction between amines and carboxylic acids in the presence of stoichiometric amounts of activating reagents. For this approach, the first step is the activation of the acid (acid chlorides, acylimidazoles, acylazides, anhydrides, and active esters), followed by aminolysis. Much effort has been made to develop a catalytic direct amidation.3
Alternatively, transamidation reaction between a carboxamide and an amine is an attractive tool in synthetic organic chemistry. However, uncatalyzed transamidation required in general drastic heating conditions.4 Different methods utilizing activating reagents or catalysts have been developed with the objective to decrease the reaction temperature.5 Despite their wide scope, these protocols required at least one of these conditions: (i) energetically favorable systems (ring-opening of four-membered rings;5d,e (ii) intramolecular assistance,5f or both factors); (iii) use of moisture-sensitive and/or activation reagents (up to 2–3 equivalents; borate esters,5a dialkylformamide dialkyl acetals,5b AlCl3,5g AlMe3,5c HCl5i). Examples of transamidation from the Stahl's group provided an elegant possibility of preparing amides under mild conditions.6 However, the reaction requires expensive catalyst and can also result in mixtures of amides. Recently, new copper-7 and cerium-,8 and zirconium-catalyzed9 transamidations have been developed for carboxamides,7-9 and ureas.7 For metal-free catalyzed methods of transamidation, NH2OH⋅HCl (up to 50 mol%),10 and NH3⋅HI (1 equiv)11 were reported to be catalytically active. In reality, because the free bases NH2OH (pKaNH3OH+ = 5.97) and NH3 (pKaNH4+ = 9.21) are less basic than most alkyl amines (for example, pKaBnNH3+ = 9.34, pKaCyclohexylNH3+ = 10.64)12 and because ammonia is more volatile than any amine, the corresponding salts NH2OH⋅HCl and NH3⋅HI would transfer their acid to alkyl amines to provide aliphatic ammonium, which could probably be the principle catalytic species in these two examples.5i In the case of NH3⋅HI, high reactivities with N-alkylamines were observed at the expense of using excess of ethylenediamine - a strong and chelating base - under microwave activation. Very recently, PhI(OAc)2,13 glycine14 and dioxane15 were shown to be capable of catalyzing the transamidation reaction.
We recently reported a highly convenient access to amides by solvent-free boric acid-catalyzed transamidation of carboxamides with amines.16 In conjunction with this work, we became interested in achieving such transformation using a new class of robust, tunable catalysts under practical and mild conditions. In this regard, azoles (imidazoles,17 pyrazoles, triazoles,18 benzimidazoles, benzotriazoles,19 etc.) would be especially attractive candidates. The electronic nature of the azoles is dictated by the number and position of the nitrogen atoms and therefore could determine the effectiveness of the azole catalyst (Scheme 1). Classically, their N-acyl derivatives – azolides – have proved to be effective acylating agents for amines.20 However, it should be kept in mind that this method encounters two intrinsic drawbacks: (i) the formation of azolides from the corresponding acids requires azoles in stoichiometric amounts and an additional step of activation; and (ii) the subsequent aminolysis of azolides will release inevitably the parent azoles as by-products.
RESULTS AND DISCUSSION
To identify efficient transamidation catalysts, we screened several commercially available azoles (10 mol%) for their ability to catalyze the reaction of butyramide 1a with benzylamine 2a under solvent-free conditions (Table 1).
At 80 °C for 24 h, the uncatalyzed transamidation did not occurred (Entry 1). Unsubtituted imidazole (Entry 2) and its derivatives (Entries 3-4) showed only negligible effect. A slight catalytic effect was observed with pyrazole and indazole (Entries 5-6).
We next turned our attention to the use of triazole derivatives. To our delight, simple triazoles showed enhanced catalytic effect (Entries 7-8) compared to pyrazoles. We theorized that the presence of at least two nitrogen atoms bonded together might be responsible for this catalytic effect. The most remarkable conversion was obtained with benzotriazole (Entry 9). Finally, two N-substituted benzotriazoles were tested and displayed considerably lower efficiency (Entries 10-11).
The scope of the benzotriazole catalyzed transamidation was examined by reaction of a number of structurally diverse amides and amines using 10% catalyst (Table 2) on a preparative scale. The reactions of n-butyramide 1a and acetamide 1b proceeded cleanly with aliphatic primary and secondary amines (2a-d, f and g) bearing various substituents at moderate temperatures. The desirable carboxamides were obtained in high yields (Entries 1-4, 6-8). While the reaction of 1a with 2c occurred without incidence, the transamidation of 1a with 2d provided small quantity of ring closing product, probably due to higher temperature required in the latter case. With low nucleophilic aromatic amine 2e, the transamidation required higher temperature to afford the product in useful yields (Entries 5 and 9). Aromatic substrates such as benzamide 1c and p-toluamide 1d also reacted well although heating at higher temperature is required (Entries 10-15). Interestingly, formamide 1e displayed a very high reactivity under the present conditions. With benzylamine 2a, the reaction achieved full conversion even at room temperatute (Entry 16). Reaction with hindered amine such as cyclohexylamine required heating only at 50 °C (Entry 17). At 100 °C, 1e reacts cleanly with aromatic amines (Entries 18-19). These promising results with 1e suggest that 1e could be an attracting alternative formylation reagent using our benzotriazole catalyst.17
The transamidation reactivity of N-monosubstituted (1f',1h') and N,N-disubstituted amides (1f'',1h'') were next evaluated with amines (Entries 20-26). As a general trend, the reactivity of amides is reduced significantly with their N-substitution. Finally, our method can be applied successfully to heterocyclic amides such as nicotinamide 1f and isonicotinamide 1g which could provide new rapid access to biologically important N-substituted nicotinamides starting from readily available unsubstituted nicotinamide.
To fulfil our study, we turn our attention to the application of the method to imides and urea (Table 3). Succinimide 1h and glutarimide 1i were subjected to standard reaction conditions with 2 equiv of amines (Entries 1-3). Complete conversion of the starting materials was observed. Unsubstituted urea 1j can react with different monofunctional aliphatic and aromatic amines (Entries 5-8). Unsurprisingly, the reaction of urea 1j with diamines 2k and 2l leads to the corresponding cyclic 3jk and 3jl ureas in high yields (Entries 9 and 10).
One possible supposition for this catalytic effect is that benzotriazole attacks the starting amide, generating an activated azolide for the second step of aminolysis (Scheme 2). To test this hypothesis, an equimolar mixture of benzotriazole and acetamide was heated at 150 °C for 24 h. Although the formation of gaseous ammoniac could be a thermodynamically favorable factor for this hypothesized reaction, both starting materials were recovered unchanged, excluding this reaction pathway.
We next focused our attention on another mode of activation by formation of cooperative hydrogen bonds as a driving force to explain benzotriazole-catalyzed transamidation. It is possible that benzotriazole activates effectively the starting amide by forming two hydrogen bonds (Scheme 2).21 This intermolecular association by hydrogen bond would allow an easy aminolysis by the primary or secondary amine with loss of ammonia.
This hypothesis is supported by the fact that when imidazole derivatives (Table 1, Entries 2-4) or N-methylated benzotriazoles (Table 1, Entries 10-11) were used, the formation of only one hydrogen bond is possible between the catalyst and the starting amide. Consequently, we consider that the second nitrogen atom in benzotriazole is required to fix the amide and the catalyst closely, resulting in an efficient activation for subsequent aminolysis.
In summary, we have identified benzotriazole as an efficient organocatalyst for transamidation reaction. This catalyst can be applied efficiently in the preparation of diversely substituted amides bearing different functional groups under mild conditions. The presence of two contiguous nitrogen atoms in the catalyst is of crucial importance. Overall, this method represents an operationally simple and an easily scalable. Further studies on transamidation reaction are currently investigated in our group and will be reported in due course.
EXPERIMENTAL
General procedure for transamidation reaction. A mixture of amide (5 mmol), amine (5 mmol) and benzotriazole (60 mg, 0.5 mmol) was stirred in a tube at indicated temperature for indicated reaction time (See Tables 1-5). After being cooled to room temperature, the crude reaction mixture was purified by recrystallization or silica gel column chromatography (dissolved in dichloromethane, eluted with dichloromethane and ethyl acetate).
N-Benzylbutyramide (3aa).9 Purification of the crude mixture by column chromatography (CH2Cl2:AcOEt 98:2) afforded the product as a white powder. 1H NMR (500 MHz, CDCl3) δ 7.36-7.27 (m, 5H), 5.85 (broad s, 1H), 4.46 (d, J = 5.8 Hz, 2H), 2.20 (t, J = 7.3 Hz, 2H), 1.70 (sextuplet, J = 7.3 Hz, 2H), 0.97 (t, J = 7.3 Hz, 3H). 13C NMR (125 MHz, CDCl3) δ 173.1, 138.7, 128.7, 127.8, 127.5, 43.6, 38.7, 19.3, 13.9.
N-(4-Hydroxybutyl)butyramide (3ab). Purification of the crude mixture by column chromatography (CH2Cl2:AcOEt 1:1) afforded the product as brown oil. 1H NMR (300 MHz, CDCl3) δ 6.33 (br. s, 1H), 3.84 (br. s, 1H), 3.67 (m, 2H), 3.28 (m, 2H), 2.17 (t, J = 8.6 Hz, 2H), 1.64 (m, 6H), 0.94 (t, J = 8.6 Hz, 3H). 13C NMR (75 MHz, CDCl3) δ 174.0, 62.3, 39.7, 39.1, 29.8, 26.4, 19.2, 13.5. HRMS (ESI+) m/z [M+H]+ calcd for C8H18NO2 160.1338, found 160.1330.
N-(2-Hydroxyethyl)butyramide (3ac).22 Purification of the crude mixture by column chromatography (CH2Cl2:AcOEt 1:1) afforded the product as brown oil. 1H NMR (300 MHz, CDCl3) δ 6.72 (br. s, 1H), 4.11 (br. s, 1H), 3.71 (t, J = 5.7 Hz, 2H), 3.41 (m, 2H), 2.21 (t, J = 7.3 Hz, 2H), 1.67 (m, 2H), 0.94 (t, J = 7.3 Hz, 3H). 13C NMR (75 MHz, CDCl3) δ 174.5, 62.4, 42.6, 38.7, 19.5, 13.7.
N-(2-Hydroxy-1-phenylethyl)butyramide (3ad).23 Purification of the crude mixture by kugelrohr removal of volatiles (120 °C, 0.1 mmHg) followed by column chromatography (heptane:AcOEt 1:1) afforded the product as brown oil which crystallized slowly on standing. 1H NMR (300 MHz, CDCl3) (two rotamers 9:1) δ 7.93 (d, J = 8.1 Hz, 1H), 7.15-7.00 (m, 5H), 6.57 (br. s, 1H), 5.49 (m, 1H), 4.92 (m, 2H), 2.53 (br. s, 1H), 2.13 (t, J = 7.7 Hz, 2H), 1.56 (m, 2H), 0.81 (t, J = 7.3 Hz, 3H). 13C NMR (75 MHz, CDCl3) (two rotamers) δ 172.9, 138.0, 129.1, 128.3, 127.7, 126.6, 124.3, 119.8, 109.4, 53.2, 52.4, 38.5, 19.0, 13.8.
N-(4-Methoxyphenyl)butyramide (3ae).9 Purification of the crude mixture by column chromatography (CH2Cl2:AcOEt 95:5) afforded the product as dark solid. 1H NMR (300 MHz, CDCl3), δ 7.60 (br. s, 1H), 7.44 (d, J = 8.7 Hz, 2H), 6.84 (d, J = 8.7 Hz, 2H), 3.80 (s, 3H), 2.32 (t, J = 7.7 Hz, 2H), 1.76 (m, 2H), 1.01 (t, J = 7.3 Hz, 3H). 13C NMR (75 MHz, CDCl3), δ 171.5, 156.3, 131.2, 121.9, 114.1, 55.5, 39.4, 19.1, 13.7.
N-(4-Methoxyphenyl)acetamide (3be).24 Purification of the crude mixture by column chromatography (CH2Cl2:AcOEt 9:1) afforded the product as brown powder. 1H NMR (300 MHz, CDCl3), δ 7.41 (m, 3H), 6.86 (d, J = 8.3 Hz), 2H; 3.80 (s, 3H), 2.16 (s, 3H). 13C NMR (75 MHz, CDCl3), δ 168.4, 156.4, 130.9, 122.1, 114.3, 55.8, 24.4.
N-(4-Methoxybenzyl)acetamide (3bf).9 Purification of the crude mixture by column chromatography (CH2Cl2:AcOEt 80:20) afforded the product as a white powder.
1H NMR (300 MHz, CDCl3), δ 7.24 (d, J = 8.8 Hz, 2H), 6.88 (d, J = 8.8 Hz, 2H), 5.86 (br. s, 1H), 4.37 (d, J = 5.8 Hz, 2H), 3.81 (s, 3H), 2.03 (s, 3H). 13C NMR (75 MHz, CDCl3), δ 169.9, 159.2, 130.5, 129.4, 114.2, 55.8, 43.6, 23.3.
1-Morpholinoethanone (3bg).9 Purification of the crude mixture by column chromatography (CH2Cl2:AcOEt 8:2) afforded the product as colorless oil. 1H NMR (300 MHz, CDCl3), δ 3.65 (m, 4H), 3.60 (m, 2H), 2.08 (s, 3H). 13C NMR (75 MHz, CDCl3), δ 169.2, 66.8, 66.6, 46.8, 41.7, 21.0.
N-Benzylbenzamide (3ca).25 Purification of the crude mixture by column chromatography (CH2Cl2:AcOEt 8:2) afforded the product as colorless oil. 1H NMR (500 MHz, CDCl3), δ 7.76 (d, J = 8.8 Hz, 2H), 7.48-7.24 (m, 8H), 6.52 (broad s, 1H), 4.63 (d, J = 5.8 Hz, 2H). 13C NMR (125 MHz, CDCl3), δ 167.7, 138.4, 134.5, 131.6, 128.8, 128.6, 127.9, 127.6, 127.2, 44.2.
N-Phenethylbenzamide (3ch).26 Purification of the crude mixture by column chromatography (CH2Cl2:AcOEt 9:1) afforded the product as white crystals. 1H NMR (300 MHz, CDCl3) δ 7.78-7.22 (m, 10H), 6.27 (br. s, 1H), 3.77 (dd, J = 13.3, 7.6 Hz, 2H), 2.98 (t, J = 13.3 Hz, 3H). 13C NMR (75 MHz, CDCl3) δ 167.6, 139.0, 134.7, 131.4, 128.9, 128.8, 128.6, 126.8, 126.6, 41.1, 35.7.
N-(4-Hydroxybutyl)-4-methylbenzamide (3db). Purification of the crude mixture by kugelrohr removal of volatiles (120 °C, 0.1 mmHg) followed by column chromatography (heptane:AcOEt 1:1) afforded the product as brown oil. 1H NMR (300 MHz, CDCl3) δ 7.70 (d, J = 8.0 Hz, 2H), 7.20 (d, J = 8.0 Hz, 2H), 6.83 (br. s, 1H), 3.70 (t, J = 6.2 Hz, 2H), 3.49 (dd, J = 13.0, 6.2 Hz, 2H), 2.40 (s, 3H), 1.69 (m, 4H). 13C NMR (75 MHz, CDCl3) δ 167.8, 141.8, 129.4, 127.1, 125.5, 62.3, 39.8, 30.1, 26.4, 21.6. HRMS (ESI+) m/z [M+H]+ calcd for C12H18NO2 208.1338, found 208.1345.
N-(2-Hydroxyethyl)-4-methylbenzamide (3dc).27 Purification of the crude mixture by kugelrohr removal of volatiles (120 °C, 0.1 mmHg) followed by column chromatography (heptane:AcOEt 1:1) afforded the product as brown oil. 1H NMR (300 MHz, CDCl3) δ 7.70 (d, J = 8.5 Hz, 2H), 7.21 (d, J = 8.5 Hz, 2H), 6.99 (br. s, 1H), 3.82 (m, 2H), 3.61 (m, 2H), 2.39 (s, 3H). 13C NMR (75 MHz, CDCl3) δ 168.8, 142.2, 131.3, 129.3, 127.3, 62.1, 43.1, 21.7.
N-Benzylformamide (3ea).16 Purification of the crude mixture by column chromatography (CH2Cl2:AcOEt 98:2) afforded the product as a white powder. 1H NMR (300 MHz, CDCl3), two rotamers. δ 8.24 [s, d (J = 12.0 Hz), 1H], 7.50-7.20 (m, 5H), 6.38 (m, 1H), [4.48 (d, J = 6.0 Hz), 4.42 (d, J = 6.0 Hz), 2H]. 13C NMR (75 MHz, CDCl3), two rotamers. δ 165.0, 161.3, 137.6, 128.9, 128.8, 128.0, 127.8, 127.7, 127.0, 45.6, 42.3.
N-(4-Methoxyphenyl)formamide (3ee).16 Purification of the crude mixture by column chromatography (CH2Cl2:AcOEt 95:5) afforded the product as brown powder. 1H NMR (300 MHz, CDCl3), two rotamers. δ [9.13 (d, J = 11.8 Hz), 8.81 (br. s), 1H], 8.53 (d, J = 11.8 Hz), 8.26 (d, J = 2.0 Hz), 1H], (7.47, 7.04, 6.85, 3m, 4H), (3.77, 3.74, 2s, 3H). 13C NMR (75 MHz, CDCl3), two rotamers. δ 163.6, 159.9, 157.5, 156.6, 130.3, 129.9, 122.1, 121.4, 116.9, 114.9, 114.2, 55.5, 55.4.
N-Cyclohexylformamide (3ei).16 Purification of the crude mixture by column chromatography (CH2Cl2:AcOEt 9:1) afforded the product as yellow solid. 1H NMR (300 MHz, CDCl3) δ 8.12 (s, 1H), 8.06 (s, 1H), 6.20 (broad s, 1H), 5.98 (broad s, 1H), 3.89-3.75 (m, 1H) and 3.35-3.22 (m, 1H), 1.94-1.08 (m, 10H). 13C NMR (75 MHz, CDCl3) δ 163.8, 160.5, 51.2, 47.2, 34.8, 33.1, 25.5, 25.1, 24.9.
N-p-Tolylformamide (3ej).28 Purification of the crude mixture by column chromatography (CH2Cl2:AcOEt 95:5) afforded the product as yellow solid. 1H NMR (300 MHz, CDCl3), two rotamers (1:1) δ 8.97, 8.18 (2 br. s, 1H), [8.66 (d, J = 12.0 Hz), 8.33 (s), 1H], 7.48 (d, J = 8.5.0 Hz, 1H), 7.16-6.38 (m, 2H), 7.02 (d, J = 8.5.0 Hz, 1H), 2.37, 2.33 (2s, 3H). 13C NMR (75 MHz, CDCl3), two rotamers δ 163.3, 159.5, 135.0, 134.8, 130.3, 129.7, 120.4, 119.3, 20.9, 20.8.
N-Phenethylnicotinamide (3fh).29 Purification of the crude mixture by column chromatography (CH2Cl2:AcOEt 9:1) afforded the product as a white powder. 1H NMR (300 MHz, CDCl3) δ 8.88 (s, 1H), 8.58 (s, 1H), 8.05 (m, 1H), 7.71 (m, 7H), 3.69 (dd, J = 12.8, 6.9 Hz, 2H), 2.92 (t, J = 6.9 Hz, 2H). 13C NMR (75 MHz, CDCl3) δ 166.0, 151.8, 147.9, 138.9, 135.4, 129.1, 126.6, 123.6, 41.6, 35.8.
N-Hexylnicotinamide (3fj).30 Purification of the crude mixture by column chromatography (CH2Cl2:AcOEt 9:1) afforded the product as a white powder. 1H NMR (300 MHz, CDCl3) δ 8.94 (m, 1H), 8.52 (br. s, 1H), 8.05 (m, 1H), 7.76 (m, 1H), 7.23 (m, 1H), 3.33 (dd, J = 13.3, 6.7 Hz, 2H), 1.51 (m, 2H), 1.19 (m, 6H), 0.76 (t, J = 6.6 Hz, 3H). 13C NMR (75 MHz, CDCl3) δ 165.8, 151.9, 148.3, 135.4, 130.9, 123.6, 40.3, 31.4, 29.4, 26.7, 22.5, 14.0.
N-Phenethylisonicotinamide (3gh). Purification of the crude mixture by column chromatography (CH2Cl2:AcOEt 9:1) afforded the product as a white powder. 1H NMR (300 MHz, CDCl3) δ 8.67 (m, 2H), 7.53 (m, 2H), 7.29 (m, 5H), 6.63 (br. s, 1H), 3.75 (dd, J = 12.8, 6.9 Hz, 2H), 2.95 (t, J = 6.9 Hz, 2H). 13C NMR (75 MHz, CDCl3) δ 165.5, 150.6, 138.5, 128.8, 121.3, 41.5, 35.3. HRMS (ESI+) m/z [M+H]+ calcd for C14H15N2O 227.2817, found 227.2830.
N-Hexylisonicotinamide (3gj).31 Purification of the crude mixture by column chromatography (CH2Cl2:AcOEt 9:1) afforded the product as a white powder. 1H NMR (300 MHz, CDCl3) δ 8.61 (m, 2H), 7.62 (m, 2H), 7.29 (br. s, 1H), 3.38 (dd, J = 13.3, 6.7 Hz, 2H), 1.55 (m, 2H), 1.26 (m, 6H), 0.83 (t, J = 6.6 Hz, 3H). 13C NMR (75 MHz, CDCl3) δ 165.6, 150.4, 142.1, 121.4, 40.7, 31.8, 29.6, 27.0, 22.6, 14.1.
N1,N4-Dibenzylsuccinamide (3ha).32 Purification of the crude mixture by washing with CH2Cl2 afforded the product as a white powder. 1H NMR (300 MHz, CDCl3) δ 8.35 (t, J = 5.2 Hz, 2H), 7.27 (m, 10H), 4.27 (d, J = 5.2 Hz, 4H), 2.43 (s, 4H). 13C NMR (75 MHz, CDCl3) δ 171.2, 139.5, 128.2, 127.1, 126.6, 40.0, 30.7.
N1,N4-Dicyclohexylsuccinamide (3hi).33 Purification of the crude mixture by washing with CH2Cl2 afforded the product as gray powder. 1H NMR (300 MHz, CDCl3) δ 7.66 (m, 2H), 3.49 (m, 2H), 2.53 (4H), 1.72-1.40 (m, 10H), 1.31-0.99 (m, 10H). 13C NMR (75 MHz, CDCl3) δ 170.8, 47.7, 33.0, 31.7, 25.9, 25.0.
N1,N5-Dibenzylglutaramide (3ia).32 Purification of the crude mixture by washing with CH2Cl2 afforded the product as a white powder. 1H NMR (300 MHz, CDCl3) δ 8.34 (br. s, 2H), 7.35-7.15 (m, 10H), 4.28 (d, J = 5.8 Hz, 4H), 2.17 (t, J = 7.9 Hz, 4H), 1.79 (m, 2H). 13C NMR (75 MHz, CDCl3) δ 171.2, 140.1, 128.8, 127.4, 127.2, 42.5, 35.3, 22.1.
1,3-Dibenzylurea (3ja’).34 Purification of the crude mixture by trituration (H2O and heptane) afforded the product as white crystals. 1H NMR (300 MHz, CDCl3) δ 7.35-7.20 (m, 10 H), 6.46 (t, J = 5.8 Hz, 2H), 4.25 (d, J = 5.8 Hz, 10 H). 13C NMR (75 MHz, CDCl3) δ 158.5, 141.4, 128.7, 127.5, 127.0, 43.7.
1,3-Bis(4-Methoxyphenyl)urea (3je).35 Purification of the crude mixture by trituration (H2O and heptane) afforded the product as pale violet crystals. 1H NMR (300 MHz, CDCl3) δ 8.37 (broad s, 2H), 7.34 (d, J = 8.2 Hz, 4H), 6.86 (d, J = 8.2 Hz, 4H), 3.73 (s, 6H). 13C NMR (75 MHz, CDCl3) δ 155.1, 153.7, 133.5, 120.6, 114.7, 55.9.
1,3-Diphenethylurea (3jh).36 Purification of the crude mixture by trituration (H2O and heptane) afforded the product as white crystals. 1H NMR (300 MHz, CDCl3) δ 7.34-7.14 (m, 10H), 4.67 (t, J = 6.3 Hz, 2H), 3.39 (dd, J = 13.3, 6.3 Hz, 2H), 2.77 (t, J = 6.3 Hz, 2H). 13C NMR (75 MHz, CDCl3) δ 158.2, 139.2, 128.8, 128.6, 41.7, 36.6.
Tetrahydropyrimidin-2(1H)-one (3jk).37 1H NMR (300 MHz, DMSO-d6) δ 6.02 (s, 2H), 3.08 (m, 4H), 1.68 (m, 2H). 13C NMR (75 MHz, DMSO-d6) δ 156.3, 39.8, 21.7.
1-(2-Aminobenzyl)urea (3jl). Purification of the crude mixture by trituration with water and heptane afforded the product as pale yellow solid. 1H NMR (300 MHz, CDCl3) δ 7.02-6.90 (m, 2H), 6.60 (m, 1H), 6.49 (m, 1H), 6.33 (t, J = 6.1 Hz, 1H), 5.57 (broad s, 2H), 5.14 (broad s, 2H), 4.04 d, J = 6.1 Hz, 2H). 13C NMR (75 MHz, CDCl3) δ 159.8, 147.1, 129.7, 128.5, 116.1, 115.4, 40.6. HRMS (ESI+) m/z [M+H]+ calcd for C8H12N3O 166.0980, found 166.0967.
ACKNOWLEDGEMENTS
We thank ICSN-CNRS for financial support.
References
1. A. K. Ghose, V. N. Viswanadhan, and J. J. Wendoloski, J. Comb. Chem., 1999, 1, 55. CrossRef
2. (a) J. M. Humphrey and A. R. Chamberlin, Chem. Rev., 1997, 97, 2243; CrossRef (b) R. C. Larock, Comprehensive Organic Transformation, VCH: New York, 1999; (c) C. A. G. N. Montalbetti and V. Falque, Tetrahedron, 2005, 61, 10827; CrossRef (d) E. Valeur and M. Bradley, Chem. Soc. Rev., 2009, 38, 606; CrossRef (e) C. L. Allen and J. M. J. Williams, Chem. Soc. Rev., 2011, 40, 3405. CrossRef
3. For examples, see (a) R. Al-Zoubi, O. Marion, and D. G. Hall, Angew. Chem. Int. Ed., 2008, 47, 2876; CrossRef (b) D. G. Hall, M. Olivier, and R. Al-Zoubi, WO2009/030022, 2009.
4. (a) M. E. Smith and H. Adkins, J. Am. Chem. Soc., 1938, 60, 657; CrossRef (b) L. F. Beste and R. C. Houtz, J. Polym. Sci., 1952, 8, 395; CrossRef (c) I. K. Miller, J. Polym. Sci. Part A, 1976, 14, 1403; (d) F. Zaragoza-Dçrwald and G. von Kiedrowski, Synthesis, 1988, 917. CrossRef
5. For representative examples of transamidation, see: (a) P. Starkov and T. D. Sheppard, Org. Biomol. Chem., 2011, 9, 1320; CrossRef (b) T. A. Dineen, M. A. Zajac, and A. G. Myers, J. Am. Chem. Soc., 2006, 128, 16406; CrossRef (c) S. Çalimsiz and M. A. Lipton, J. Org. Chem., 2005, 70, 6218; CrossRef (d) A. Klapars, S. Parris, K. W. Anderson, and S. L. Buchwald, J. Am. Chem. Soc., 2004, 126, 3529; CrossRef (e) A. Vasudevan, C. I. Villamil, and S. W. Djuric, Org. Lett., 2004, 6, 3361; CrossRef (f) M J. Lasri, E. Gonzalez-Rosende, and J. Sepulveda-Arques, Org. Lett., 2003, 5, 3851; CrossRef (g) E. Bon, D. C. B. Bigg, and G. Bertrand, J. Org. Chem., 1994, 59, 4035; CrossRef (h) H. H. Wasserman, G. O. Berger, and K. R. Cho, Tetrahedron Lett., 1982, 23, 465; CrossRef (i) A. Galat and G. Elion, J. Am. Chem. Soc., 1943, 65, 1566. CrossRef
6. (a) N. A. Stephenson, J. Zhu, S. H. Gellman, and S. S. Stahl, J. Am. Chem. Soc., 2009, 131, 10003; CrossRef (b) J. M. Hoerter, K. M. Otte, S. H. Gellman, Q. Cui, and S. S. Stahl, J. Am. Chem. Soc., 2008, 130, 6474; CrossRef (c) D. A. Kissounko, J. M. Hoerter, L. A. Guzei, Q. Cui, S. H. Gellman, and S. S. Stahl, J. Am. Chem. Soc., 2007, 129, 1776; CrossRef (d) J. M. Hoerter, K. M. Otte, S. H. Gellman, and S. S. Stahl, J. Am. Chem. Soc., 2006, 128, 5177; CrossRef (e) D. A. Kissounko, L. A. Guzei, S. H. Gellman, and S. S. Stahl, Organometallics, 2005, 24, 5208; CrossRef (f) S. E. Eldred, D. A. Stone, S. H. Gellman, and S. S. Stahl, J. Am. Chem. Soc., 2003, 125, 3422. CrossRef
7. M. Zhang, S. Imm, S. Bähn, L. Neubert, H. Neumann, and M. Beller, Angew. Chem. Int. Ed., 2012, 51, 3905. CrossRef
8. M. Tamura, T. Tonomura, K. Shimizu, and A. Satsuma, Green Chem., 2012, 14, 717. CrossRef
9. B. N. Atkinson, A. R. Chhatwal, H. V. Lomax, J. W. Walton, and J. M. J. Williams, Chem. Commun., 2012, 48, 11626. CrossRef
10. C. L. Allen, B. N. Atkinson, and J. M. J. Williams, Angew. Chem. Int. Ed., 2012, 51, 1383. CrossRef
11. Y. Shimizu, H. Morimoto, M. Zhang, and T. Ohshima, Angew. Chem. Int. Ed., 2012, 51, 8564. CrossRef
12. H. K. Hall Jr., J. Am. Chem. Soc., 1957, 79, 5441. CrossRef
13. R. Vanjari, B. K. Allam, and K. N. Singh, RSC Adv., 2013, 3, 1691.
14. S. N. Rao, D. C. Mohan, and S. Adimurthy, Org. Lett., 2012, 15, 1496. CrossRef
15. R. Vanjari, B. K. Allam, and K. N. Singh, Tetrahedron Lett., 2013, 54, 2553. CrossRef
16. T. B. Nguyen, J. Sorres, M. Q. Tran, L. Ermolenko, and A. Al-Mourabit, Org. Lett., 2012, 14, 3202. CrossRef
17. Imidazole was proved to promote N-formylation of amino acid esters and primary amines using DMF as formyl source: M. Suchý, A. A. H. Elmehriki, and R. H. E. Hudson, Org. Lett., 2011, 13, 3952. CrossRef
18. 1,2,4-Triazole anion has been used as an active acyl transfer catalyst for the aminolysis and transesterification of esters: X. Yang and V. B. Birman, Org. Lett., 2009, 11, 1499. CrossRef
19. For reviews, see: a) A. R. Katritzky, X. Lan, J. Z. Yang, and O. V. Denisko, Chem. Rev., 1998, 98, 409; CrossRef (b) A. R. Katritzky, M. Kuanar, S. Slavov, C. D. Hall, M. Karelson, I. Kahn, and D. A. Dobchev, Chem. Rev., 2010, 110, 5714; CrossRef (c) A. R. Katritzky and S. Rachwal, Chem. Rev., 2011, 111, 7063. CrossRef
20. (a) H. A. Staab, H. Bauer, and K. M. Schneider, Azolides in Organic Synthesis and Biochemistry; Wiley-VCH Verlag GmbH & Co. KGaA, 2002; (b) S. E. Denmark and G. L. Beutner, Angew. Chem. Int. Ed., 2008, 47, 1560. CrossRef
21. For hydrogen bond complexation of benzotriazole with amines or phenols, see a) C. Zonta, M. Crisma, O. De Lucchi, R. Motterle, and S. Serafini, Org. Lett., 2006, 8, 1577; CrossRef (b) C. Zonta, O. De Lucchi, R. Motterle, and S. Serafini, J. Phys. Org. Chem., 2011, 24, 122. CrossRef
22. J. Morales-Sanfrutos, A. Megia-Fernandez, F. Hernandez-Mateo, F. Santoyo-Gonzalez, M. D. Giron-Gonzalez, and R. Salto-Gonzalez, Org. Biomol. Chem., 2011, 851. CrossRef
23. T. Matsui, T. Kondo, Y. Nishita, S. Itadani, S. Nakatani, N. Omawari, M. Sakai, S. Nakazawa, A. Ogata, H. Mori, K. Terai, W. Kamoshima, H. Ohno, T. Obata, H. Nakai, and M. Toda, Bioorg. Med. Chem., 2002, 10, 3757. CrossRef
24. C. Spiteri and J. E. Moses, Synlett, 2012, 23, 1546. CrossRef
25. S. C. Ghosh, J. S. Y. Ngiam, C. L. L. Chai, A. M. Seayad, T. T. Dang, and A. Chen, Adv. Synth. Catal., 2012, 354, 1407. CrossRef
26. R. Cadoni, A. Porcheddu, G. Giacomelli, and L. De Luca, Org. Lett., 2012, 14, 5014. CrossRef
27. M. N. Holerca and V. Percec, Eur. J. Org. Chem., 2000, 2257. CrossRef
28. T. Ikawa, T. E. Barder, M. R. Biscoe, and S. L. Buchwald, J. Am. Chem. Soc., 2007, 129, 13001. CrossRef
29. C. M. Boehner, D. M. Marsden, H. F. Sore, D. R. Spring, and D. Norton, Tetrahedron Lett., 2010, 51, 5930. CrossRef
30. M. R. Sambrook, P. D. Beer, J. A. Wisner, R. L. Paul, A. R. Cowley, F. Szemes, and M. G. B. Drew, J. Am. Chem. Soc., 2005, 127, 2292. CrossRef
31. L. Rubio-Perez, P. Sharma, F. J. Perez-Flores, L. Velasco, A. Cabrera, and J. L. Arias, Tetrahedron, 2012, 68, 2342. CrossRef
32. M. Kunishima, C. Kawachi, K. Hioki, K. Terao, and S. Tani, Tetrahedron, 2001, 57, 1551. CrossRef
33. K. Saito and T. Sato, Bull. Chem. Soc. Jpn., 1979, 52, 3601. CrossRef
34. D. Kong, L. He, and J. Wang, Synlett, 2010, 1276. CrossRef
35. T. Mizuno, M. Mihara, T. Nakai, T. Iwai, and T. Ito, Synthesis, 2007, 3135. CrossRef
36. J. C. Anderson and R. B. Moreno, Org. Biomol. Chem., 2012, 10, 1334. CrossRef
37. J. Paz, C. Perez-Balado, B. Iglesias, and L. Munoz, J. Org. Chem., 2010, 75, 3037. CrossRef