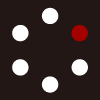
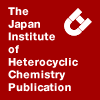
HETEROCYCLES
An International Journal for Reviews and Communications in Heterocyclic ChemistryWeb Edition ISSN: 1881-0942
Published online by The Japan Institute of Heterocyclic Chemistry
e-Journal
Full Text HTML
Received, 7th July, 2013, Accepted, 25th September, 2013, Published online, 7th October, 2013.
DOI: 10.3987/COM-13-S(S)88
■ Synthesis of de novo Chiral γ-Amino-ynamides Using Lithiated Ynamides. Observation of a Unique 5-endo-dig Cyclization with an Inversion of S-Center
Xiao-Na Wang, Richard P. Hsung,* Sierra K. Fox, Ming-Can Lv, and Rui Qi
Department of Chemistry, School of Pharmacy, University of Wisconsin, 777 Highland Aenue, Madison, WI 53705, U.S.A.
Abstract
We describe herein details of our efforts in developing a highly stereoselective synthesis of de novo chiral γ-amino-ynamides through additions of lithiated ynamides to Ellman–Davis chiral N-tert-butanesulfinyl imines. While additions of ynamides could be highly stereoselective even without Lewis acids, the use of BF3-OEt2 completely reversed the stereoselectivity. On the other hand, additions of oxazolidinone-substituted, oxazinanone-substituted and tetrahydropyrimidinone-substituted ynamides behaved quite differently and functioned better with BF3-OEt2. The chirality of the oxazolidinone ring exerts no impact on the selectivity. This work also features a unique 5-endo-dig cyclization of oxazolidinone-substituted γ-amino-ynamides that could be promoted with acid, leading to isothiazoles and 2,3-dihydro-isothiazole S-oxides.INTRODUCTION
The field of ynamide chemistry has experienced rapid expansion in the past decade and has attracted significant attention from the synthetic community.1-4 Consequently, novel and improved protocols for synthetizing ynamides3 and their structural relatives5,6 have been continuously reported in the literature over the past few years.3e Recently, we have been developing N-tethered intramolecular transformations to construct N-heterocycles and encountered the need of utilizing γ-amino-ynamides such as 1 [Scheme 1],7 and we found that this class of ynamides is actually not trivial to make.
The existing copper-catalyzed protocols,1,2,5 albeit attractive, may not be the most suitable approaches, because the required optically enriched propargyl amines 3 are not always readily available, and the deprotection step could pose problem to the stability of the ynamides 2.8 The most direct and practical accesses maybe: (a) An addition of metalated chiral ynamides 4* to achiral imines 5, which would constitute a long-range stereochemical induction; or (b) addition of metalated ynamides 4 to chiral imines such as Ellman-Davis chiral N-tert-butanesulfinyl imines 6.9,10 To the best of our knowledge, the addition of metallated ynamides to imines remains unreported,1,11,12 although their additions to a number of other electrophiles have been developed.13 In particular, we note here that Poisson’s elegant work11 on stereoselective additions of lithiated ynol ethers to Ellman-Davis chiral N-tert-butanesulfinyl imines provided a significant inspiration for our own pursuit of this methodology. We report herein a highly stereoselective addition of lithiated ynamides to Ellman-Davis chiral imines for synthesizing chiral γ−amino-ynamides.
RESULTS AND DISCUSSION
Additions to imines using lithiated ynamides such as 7, which is substituted with an Evans chiral oxazolidinone auxiliary, was feasible but resulted a very low diastereoselectivity in γ-amino-ynamide 9 [Scheme 2]. This effectively ended any speculation of a possible remote 1,5-asymmetric induction. On the other hand, additions to Ellman-Davis chiral imine 11a using achiral ynamides such as 10 were more selective in affording 12, although the yield is low.
Subsequently, we found that N-sulfonyl-substituted ynamides were more suitable substrates for this asymmetric addition. Addition of lithiated ynamide 13a to 11a at -78 oC afforded γ-amino-ynamide 14 with a dr of 7:1 in favor of the (S)-isomer [entry 1, Table 1]. The selectivity improved significantly as the temperature turned higher [entries 2, 3 and 4], although the yield suffered when the reaction was carried at rt [entry 3]. The presence of TMEDA enhanced the yield, albeit at the expense of diastereoselectivity [entries 5 and 6]. The (S) relative stereochemistry at the gamma-carbon was unambiguously confirmed based on the single crystal X-ray structure of γ-amino-ynamide 14-S [Figure 1].
We next examined effects of Lewis acids. Bidentate Lewis acids appeared to be poor promoters overall [entries 7-12] with TiCl4, SnCl4, and Cu(OTf)2 impeding the reaction [entries 8, 9 and 12]. For monodentate Lewis acids, we found a complete reversal of stereoselectivity when using 1.2 to 2.0 equiv of BF3-OEt2 [entries 13 and 14],14 and the extent of reversal depended upon the amount of BF3-OEt2 that was used [entries 15 and 16]. However, such a reversal could not be realized when using other monodentate Lewis acids such as AlCl3 and AlMe2Cl [entries 17 and 18]. These combined outcomes suggest that this phenomenon is unique with BF3-OEt2.
A rationale of this stereoselectivity switch is proposed as shown in Figure 2. In the absence of a Lewis acid, the S-selectivity is likely derived from the Zimmerman-Traxler type chelated transition state. In contrast, with BF3-OEt2 coordinating to the sulfinyl oxygen atom, a synclinal or anti-periplanar approach takes precedent, thereby effectively breaking up the chelation especially when using ≥1.0 equiv. It has been suggested that other Lewis acids such as AlMe2Cl could coordinate to the imino lone pair, and thus, are not effective in deterring the pro-S-TS.9 It is noteworthy that in Poisson’s lithiated ynol-ether additions to Ellman-Davis chiral imines,11 they also documented such reversal of stereochemistry when using BF3-OEt2.
With the optimized protocols [entries 4 and 13, Table 1] in hand, our next goal was to examine the tolerance of the reaction to functionality on both ynamides 13 and Ellman-Davis chiral N-tert-butanesulfinyl imines 11 [Schemes 3 and 4]. Most of chiral γ-amino-ynamides 14-31 could be obtained in high stereoselectivities and good to high yields. Examples of the ynamide precursors included: N-sulfonyl-substituted γ-amino-ynamides [14-19, 23-25, and 29], carbamoyl-substituted γ-amino-ynamide [20], phosphoryl-substituted systems [21 and 22], oxazolidinone-substituted systems [26, 30, and 31], oxazinanone-substituted and tetrahydropyrimidinone-substituted γ-amino-ynamides 27 and 28, respectively.
In the absence of BF3-OEt2, we found an interesting effect on the selectivity for sulfonyl-substituted ynamides. Most notably, the para-nitro substituent actually eroded the diastereoselectivity significantly [17-S in Scheme 3]. This loss of selectivity is likely due to the nitro group competing for the Li-chelation in the pro-S-TS. It is a phenomenon that was also observed in 18-S when the R1 substituent is a phenyl group. N-Sulfonyl-substituted ynamides still afforded γ-amino-ynamides 23-S, 24-S, and 25-S in good
yields with high selectivities when added to imines 11b, 11c, and 11d. Carbamoyl-substituted γ-amino-ynamide 20-S could be given in better yield, and phosphoryl-substituted ynamides also afforded γ-amino-ynamides in better yields but with lower selectivities [21-S and 22-S]. In addition, oxazolidinone-substituted, oxazinanone-substituted and tetrahydropyrimidinone-substituted ynamides behaved rather differently, leading to products with very low yields and opposite selectivities [see 26-S and 28-S], and for reasons unknown to us at this moment, no reaction was observed in an attempt to synthesize γ-amino-ynamide 27-S.
When using 1.2 equiv BF3-OEt2, N-sulfonyl and carbamoyl-substituted γ-amino-ynamides 14-R through 31 could be synthesized with complete reversal of stereochemistry [Scheme 4]. Moreover, all chiral γ-amino-ynamides were isolated virtually in quantitative yields except for the oxazinanone-substituted γ-amino-ynamide 27-R and tetrahydropyrimidinone-substituted γ-amino-ynamide 28-R. The low yields of 27-R and 28-R may be caused by the rapid hydrolysis of the starting materials. The (R) relative stereochemistry at the gamma-carbon was unambiguously assigned based on the single crystal X-ray structure of γ-amino-ynamide 26-R [left side in Figure 3].
We anticipated a potential matched and mismatched scenario when using chiral ynamide [see products 30-R and 31 in Scheme 4]. Instead, the addition of chiral lithiated ynamide to either (SS)-11a or (RS)-11a led to their respective addition products 30-R and 31 in high yields and diastereoselectivities. These reactions suggest that the chirality on the oxazolidinone ring exerts no impact on the selectivity, but it is still noteworthy that 30-R and 31 represent de novo ynamides that are highly rich in chirality. Stereochemistry of 31 was unambiguously assigned based on its X-ray structure [right side in Figure 3]. Very interestingly on the other hand, the relative stereochemistry at the γ−carbon of 30-R was assigned through X-ray structure of its derivative 2,3-dihydro-isothiazole S-oxide 33 [Figure 4] obtained via a serendipitously discovered 5-endo-dig cyclization [Scheme 5].
This acid promoted 5-endo-dig cyclization deserves more comments. It occurred concomitant with the loss of the t-Bu group led to isothiazoles and 2,3-dihydro-isothiazole S-oxides respectively. The single crystal X-ray structure of 2,3-dihydro-isothiazole S-oxide 33 [Figure 4] not only provided the stereochemical assignment of 30-R but also revealed that an inversion at the S-center had taken place in 33. This cyclization appears to be unique with the oxazolidinone-substituted γ-amino-ynamides, as not all γ−amino-ynamides underwent such cyclization, and it is also consistently accompanied with a competing pathway. Aromatized products 32 and 35 are likely derived from 33 [or 34] and 36, respectively, through an acid promoted dehydrative process. Although we have been unable to expand the scope of or optimize this unique cyclization, to the best of our knowledge, it represents a novel transformation involving propargylic N-sulfinyl amines.
CONCLUSION
We have described herein details in developing an expeditious and convenient method for synthesizing chiral γ-amino-ynamides via a highly stereoselective addition of lithiated ynamides to Ellman–Davis Chiral N-tert-Butanesulfinyl Imines. Our work demonstrates that additions of ynamides can be highly stereoselective even without Lewis acids, although the use of BF3-OEt2 completely reversed the stereoselectivity. Additions of oxazolidinone-substituted, oxazinanone-substituted and tetrahydropyrimidinone-substituted ynamides appear to require BF3-OEt2, and the chirality of the oxazolidinone ring does not play a role in the asymmetric induction. This work also features a rare acid promoted 5-endo-dig cyclization of the oxazolidinone-substituted γ-amino-ynamides concomitant with the loss of the t-Bu group and an inversion at the S-center to afford isothiazoles and 2,3-dihydro-isothiazole S-oxides.
EXPERIMENTAL
General Procedure for Additions of Lithiated Ynamides to Chiral Imines.
To a flamed-dried vial were added ynamide 13a (135.2 mg, 0.47 mmol) and THF (1.58 mL, ynamide concn = 0.30 M). To this solution was added LHMDS (0.71 mL, 1.0 M in THF) at -78 oC. After the mixture was stirred at -78 oC for 1.0 h, a solution of imine (Ss)-11a15 (109.1 mg, 0.52 mmol) in THF (1 mL) was added over 1 min. The reaction mixture was then allowed to warm to -40 oC slowly and monitored using TLC analysis. When it was 9.0 h post addition of the imine, TLC analysis showed complete consumption of the starting material. The mixture was re-cooled to -78 oC, and H2O (2 mL) was added to quench the reaction. The quenched mixture was extracted with equal volume of EtOAc, dried over Na2SO4, filtered, and concentrated under reduced pressure. The crude residue was purified using silica gel flash column chromatography [Gradient eluent: 8:1~1:1 Hexane/EtOAc + 3% Et3N] to afford 14-S (161.6 mg, 0.33 mmol) in 69% yield.
14-S: Rf = 0.18 [3:1 Hexane/EtOAc]; pale yellow solid; mp 129–130 °C; 1H NMR (400 MHz, CDCl3) δ 1.16 (s, 9H), 2.41 (s, 3H), 3.51 (d, 1H, J = 5.6 Hz), 4.44 (d, 1H, J = 14.0 Hz), 4.51 (d, 1H, J = 14.0 Hz), 5.23 (d, 1H, J = 5.6 Hz), 7.24-7.29 (m, 12H), 7.72 (d, 2H, J = 8.4 Hz); 13C NMR (100 MHz, CDCl3) δ 21.6, 22.4, 50.6, 55.2, 56.2, 70.2, 79.9, 127.6, 127.7, 128.1, 128.2, 128.4, 128.5, 129.0, 129.7, 134.2, 134.4, 139.0, 144.5; IR (film) cm-1 2249m, 1597m, 1494m, 1455m, 1363s, 1168s; mass spectrum (ESI): m/z (% relative intensity) 495 (M+H)+ (100); HRMS (ESI): m/z calcd for C27H31N2O3S2 [M+H]+: 495.1771; found 495.1758.
Ynamide 15-S (228.9 mg, 0.45 mmol) was prepared from the corresponding ynamide (142.8 mg, 0.47 mmol) and imine (Ss)-11a (109.1 mg, 0.52 mmol) in 95% yield.
15-S: Rf = 0.28 [1:1 Hexane/EtOAc]; yellow oil; 1H NMR (400 MHz, CDCl3) δ 1.15 (s, 9H), 3.53 (d, 1H, J = 5.6 Hz), 3.83 (s, 3H), 4.43 (d, 1H, J = 13.6 Hz), 4.51 (d, 1H, J = 14.0 Hz), 5.23 (d, 1H, J = 5.6 Hz), 6.89 (d, 2H, J = 8.8 Hz), 7.28 (d, 10H, J = 3.6 Hz), 7.75 (d, 2H, J = 8.8 Hz); 13C NMR (100 MHz, CDCl3) δ 22.6, 50.8, 55.3, 55.7, 56.3, 70.3, 80.3, 114.4, 114.9, 127.7, 128.2, 128.4, 128.55, 128.60, 129.1, 130.1, 134.4, 139.2, 163.7; IR (film) cm-1 2248m, 1595m, 1497m, 1363m, 1262m, 1162s; mass spectrum (APCI): m/e (% relative intensity) 511 (100) (M+H)+; HRMS (ESI): m/z calcd for C27H31N2O4S2 [M+H]+: 511.1720; found 511.1718.
Ynamide 16-S (147.0 mg, 0.29 mmol) was prepared from the corresponding ynamide (135.0 mg, 0.47 mmol) and imine (Ss)-11a (109.1 mg, 0.52 mmol) in 62% yield.
16-S: Rf = 0.19 [4:1 Petroleum Ether/EtOAc]; yellow solid; mp 81-82 oC; 1H NMR (600 MHz CDCl3) δ 1.17 (s, 9H), 3.52 (d, 1H, J= 6.0 Hz), 4.46 (d, 1H, J = 12.0 Hz), 4.56 (d, 1H, J = 12.0 Hz), 5.24 (d, 1H, J = 6.0 Hz), 7.09 (t, 2H, J = 8.4 Hz), 7.26-7.29 (m, 10 H), 7.83 (dd, 2H, J = 5.4, 9.0 Hz); 13C NMR (150 MHz, CDCl3) δ 22.5, 51.0, 55.5, 56.3, 70.5, 79.9, 116.4 (d, 2JF-C = 22.7 Hz), 127.6, 128.2, 128.5, 128.58, 128.63, 129.1, 130.68 (d, 3JF-C = 9.6 Hz), 133.53 (d, 4JF-C = 2.7 Hz), 134.1, 139.0, 165.7 (d, 1JF-C = 254.7 Hz); IR (KBr) cm-1 2246m, 1590m, 1491m, 1360m, 1239m, 1176s; mass spectrum (ESI): m/e (% relative intensity) 499 (100) (M+H)+.
A separable 4:1 mixture of ynamides 17-S (157.3 mg, 0.30 mmol) and 17-R (39.3 mg, 0.07 mmol) were prepared from the corresponding ynamide (149.9 mg, 0.47 mmol) and imine (Ss)-11a (109.1 mg, 0.52 mmol) in 79% yield.
17-S: Rf = 0.62 [1:1 Hexane/EtOAc]; pale yellow oil; 1H NMR (400 MHz, CDCl3) δ 1.20 (s, 9H), 3.52 (d, 1H, J = 6.8 Hz), 4.52 (d, 1H, J = 13.6 Hz), 4.61 (d, 1H, J = 14.0 Hz), 5.25 (d, 1H, J = 6.8 Hz), 7.26-7.42 (m, 12H), 7.99 (d, 2H, J = 8.8 Hz), 8.23 (d, 2H, J = 8.4 Hz). 13C NMR (125 MHz, CDCl3) δ 22.6, 51.3, 56.0, 56.6, 71.0, 79.4, 124.4, 127.5, 128.76, 128.81, 128.84, 129.1, 129.2, 129.3, 133.7, 138.9, 142.8, 150.5; IR (film) cm-1 2251w, 1613m, 1531s, 1371m, 1348m, 1313m, 1172s; mass spectrum (ESI): m/e (% relative intensity) 526 (100) (M+H)+; HRMS (ESI): m/z calcd for C26H28N3O5S2 [M+H]+: 526.1465; found 526.1459.
A separable 6:1 mixture of ynamides 18-S (150.2 mg, 0.31 mmol) and 18-R (25.0 mg, 0.05 mmol) were prepared from the corresponding ynamide (118.2 mg, 0.44 mmol) and imine (Ss)-11a (100.3 mg, 0.48 mmol) in 84% yield.
18-S: Rf = 0.46 [1:1 Hexane/EtOAc]; pale yellow oil; 1H NMR (400 MHz, CDCl3) δ 1.20 (s, 9H), 2.40 (s, 3H), 3.63 (d, 1H, J = 5.6 Hz), 5.35 (d, 1H, J = 5.6 Hz), 7.18 (d, 2H, J = 8.4 Hz), 7.26-7.41 (m, 8H), 7.48-7.52 (m, 4H). 13C NMR (125 MHz, CDCl3) δ 21.9, 22.7, 51.1, 56.5, 69.7, 80.6, 126.3, 128.0, 128.4, 128.45, 128.51, 128.8, 129.3, 129.7, 133.0, 138.7, 139.3, 145.1; IR (film) cm-1 2254m, 1596m, 1492m, 1455m, 1373m, 1175s; mass spectrum (ESI): m/e (% relative intensity) 481 (100) (M+H)+; HRMS (ESI): m/z calcd for C26H29N2O3S2 [M+H]+: 481.1615; found 481.1622.
Ynamide 19-S (125.5 mg, 0.31 mmol) were prepared from the corresponding ynamide (99.2 mg, 0.47 mmol) and imine (Ss)-11a (109.1 mg, 0.52 mmol) in 63% yield.
19-S: Rf = 0.22 [1:1 Hexane/EtOAc]; pale yellow oil; 1H NMR (400 MHz, CDCl3) δ 1.18 (s, 9H), 2.41 (s, 3H), 3.06 (s, 3H), 3.62 (d, 1H, J = 6.0 Hz), 5.28 (d, 1H, J = 6.0 Hz), 7.25-7.39 (m, 5H), 7.46-7.48 (m, 2H), 7.69-7.72 (m, 2H); 13C NMR (100 MHz, CDCl3) δ 21.7, 22.6, 39.0, 51.2, 56.4, 68.1, 81.6, 127.8, 128.0, 128.4, 128.8, 129.9, 133.2, 139.3, 144.9; IR (film) cm-1 2251m, 1597m, 1454m, 1364s, 1171s; mass spectrum (ESI): m/e (% relative intensity) 419 (100) (M+H)+; HRMS (ESI): m/z calcd for C21H27N2O3S2 [M+H]+: 419.1458; found 419.1460.
Ynamide 20-S (72.9 mg, 0.18 mmol) were prepared from the corresponding ynamide (39.8 mg, 0.21 mmol) and imine (Ss)-11a (48.4 mg, 0.23 mmol) in 87% yield.
20-S: Rf = 0.25 [1:1 Hexane/EtOAc]; pale yellow solid; mp 85-86 oC; 1H NMR (400 MHz, CDCl3) δ 1.19 (s, 9H), 3.57 (d, 1H, J = 6.0 Hz), 3.82 (s, 3H), 4.63 (s, 2H), 5.31 (d, 1H, J = 5.6 Hz), 7.26-7.39 (m, 10H); 13C NMR (125 MHz, CDCl3) δ 22.6, 51.0, 53.7, 54.2, 56.3, 70.1, 80.3, 127.8, 128.15, 128.23, 128.59, 128.62, 128.84, 135.9, 139.4, 155.7; IR (film) cm-1 2260m, 1726s, 1444m, 1383m, 1285m, 1220m; mass spectrum (ESI): m/e (% relative intensity) 399 (100) (M+H)+; HRMS (ESI): m/z calcd for C22H27N2O3S [M+H]+: 399.1737; found 399.1726.
A 13:1 mixture of ynamides 21-S/R (203.6 mg, 0.42 mmol) were prepared from the corresponding ynamide (132.3 mg, 0.47 mmol) and imine (Ss)-11a (109.1 mg, 0.52 mmol) in 88% yield.
21-S: Rf = 0.28 [1:1 CH2Cl2/EtOAc]; pale yellow oil; 1H NMR (400 MHz, CDCl3) δ 0.93 (s, 3H), 1.10 (s, 3H), 1.16 (s, 9H), 3.51 (d, 1H, J = 6.0 Hz), 4.05-4.16 (m, 4H), 4.42-4.51 (m, 2H), 5.21 (dd, 1H, J = 2.8, 5.2 Hz), 7.25-7.41 (m, 10H). 13C NMR (125 MHz, CDCl3) δ 20.9, 21.5, 22.6, 32.2 (d, J = 6.6 Hz), 51.1 (d, J = 1.4 Hz), 54.7 (d, J = 5.8 Hz), 56.2, 65.4 (d, J = 5.8 Hz), 78.5 (dd, J = 7.3, 36.4 Hz), 82.4 (d, J = 4.8 Hz), 127.6, 128.1, 128.5, 128.6, 129.0, 136.2 (d, J = 1.9 Hz), 139.5; 31P NMR (202 MHz, CDCl3) δ -1.99; IR (film) cm-1 2970w, 2247m, 1455m, 1281m, 1052s; mass spectrum (ESI): m/e (% relative intensity) 489 (100) (M+H)+; HRMS (ESI): m/z calcd for C25H34N2O4PS [M+H]+: 489.1972; found 489.1985.
A 6:1 mixture of ynamides 22-S and 22-R (183.1 mg, 0.42 mmol) were prepared from the corresponding ynamide (108.6 mg, 0.47 mmol) and imine (Ss)-11a (109.1 mg, 0.52 mmol) in 88% yield.
22-S: Rf = 0.32 [1:1 CH2Cl2/EtOAc]; pale yellow oil; 1H NMR (400 MHz, CDCl3) δ 0.94 (s, 3H), 1.10 (s, 3H), 1.20 (s, 9H), 3.64 (d, 1H, J = 5.6 Hz), 3.92 (dd, 2H, J = 6.8, 8.0 Hz), 4.04-4.15 (m, 4H), 5.25-5.36 (m, 2H), 5.87-5.97 (m, 1H), 7.28-7.36 (m, 3H), 7.47 (d, 2H, J = 7.2 Hz), 13C NMR (100 MHz, CDCl3) δ 20.9, 21.5, 22.6, 32.2 (d, J = 6.9 Hz), 51.1 (d, J = 1.6 Hz), 53.5 (d, J = 5.3 Hz), 56.2, 64.6 (d, J = 5.4 Hz), 78.4 (dd, J = 6.9, 25.3 Hz), 82.5 (d, J = 4.6 Hz), 119.2, 127.6, 128.2, 128.6, 132.4 (d, J = 2.3 Hz), 139.6; 31P NMR (162 MHz, CDCl3) δ -2.10; IR (film) cm-1 2974w, 2248m, 1455m, 1279m, 1052s; mass spectrum (APCI): m/e (% relative intensity) 439 (100) (M+H)+; HRMS (ESI): m/z calcd for C21H31N2O4PS [M+H]+: 439.1815; found 439.1801.
Ynamide 23-S (129.9 mg, 0.25 mmol) was prepared from the corresponding ynamide 13a (111.7 mg, 0.39 mmol) and imine (Ss)-11b16 (103.1 mg, 0.43 mmol) in 63% yield.
23-S: Rf = 0.28 [1:1 Hexane/EtOAc]; pale yellow oil; 1H NMR (400 MHz, CDCl3) δ 1.14 (s, 9H), 2.41 (s, 3H), 3.48 (d, 1H, J = 5.2 Hz), 3.78 (s, 3H), 4.44 (d, 1H, J = 14.0 Hz), 4.50 (d, 1H, J = 13.6 Hz), 5.17 (d, 1H, J = 5.2 Hz), 6.80 (d, 2H, J = 8.8 Hz), 7.18-7.28 (m, 9H), 7.72 (d, 2H, J = 8.0 Hz); 13C NMR (100 MHz, CDCl3) δ 21.7, 22.6, 50.2, 55.36, 55.40, 56.2, 70.6, 79.8, 113.9, 127.9, 128.4, 128.6, 129.05, 129.14, 129.8, 131.3, 134.4, 134.6, 144.7, 159.5; IR (film) cm-1 2248w, 1611w, 1456m, 1364m, 1250m, 1169s; mass spectrum (ESI): m/e (% relative intensity) 525 (100) (M+H)+; HRMS (ESI): m/z calcd for C28H33N2O4S2 [M+H]+: 525.1877; found 525.1881.
Ynamide 24-S (183.3 mg, 0.37 mmol) was prepared from the corresponding ynamide 13a (135.2 mg, 0.47 mmol) and imine (Ss)-11c16 (112.3 mg, 0.52 mmol) in 77% yield.
24-S: Rf = 0.51 [1:1 Hexane/EtOAc]; pale yellow solid; mp 119–120 °C; 1H NMR (400 MHz, CDCl3) δ 0.81-0.90 (m, 2H), 0.99-1.20 (m, 3H), 1.17 (s, 9H), 1.43-1.46 (m, 1H), 1.56-1.67 (m, 5H), 2.43 (s, 3H), 3.09 (d, 1H, J = 6.4 Hz), 3.88 (t, 1H, J = 6.0 Hz), 4.42 (d, 1H, J = 13.6 Hz), 4.50 (d, 1H, J = 14.0 Hz), 7.27-7.33 (m, 7H), 7.79 (d, 2H, J = 8.4 Hz); 13C NMR (100 MHz, CDCl3) δ 21.6, 22.6, 25.8, 25.9, 26.2, 27.8, 29.4, 43.3, 53.0, 55.4, 56.1, 70.1, 78.6, 127.9, 128.3, 128.5, 129.0, 129.8, 134.5, 134.6, 144.6; IR (film) cm-1 2925brm, 2852w, 2250w, 1361m, 1168s, 1070m; mass spectrum (ESI): m/e (% relative intensity) 501 (100) (M+H)+; HRMS (ESI): m/z calcd for C27H37N2O3S2 [M+H]+: 501.2241; found 501.2235.
Ynamide 25-S (162.2 mg, 0.32 mmol) was prepared from the corresponding ynamide 13a (135.2 mg, 0.47 mmol) and imine (Ss)-11d17 (113.3 mg, 0.52 mmol) in 68% yield.
25-S: Rf = 0.52 [1:1 Hexane/EtOAc]; white solid; mp 69–70 °C; 1H NMR (400 MHz, CDCl3) δ 0.88 (t, 3H, J = 7.0 Hz), 1.16 (s, 9H), 1.18-1.29 (m, 8H), 1.48-1.64 (m, 2H), 2.43 (s, 3H), 3.13 (d, 1H, J = 5.6 Hz), 4.02-4.07 (m, 1H), 4.43 (d, 1H, J = 13.6 Hz), 4.50 (d, 1H, J = 13.6 Hz), 7.27-7.32 (m, 7H), 7.77 (d, 2H, J = 8.4 Hz); 13C NMR (100 MHz, CDCl3) δ 14.2, 21.7, 22.6, 22.7, 25.5, 28.9, 31.8, 37.0, 47.7, 55.5, 56.0, 71.2, 78.2, 127.9, 128.4, 128.6, 129.1, 129.8, 134.6, 134.7, 144.7; IR (film) cm-1 2926brm, 2856m, 2252w, 1456m, 1364m, 1169s; mass spectrum (ESI): m/e (% relative intensity) 503 (100) (M+H)+; HRMS (ESI): m/z calcd for C27H39N2O3S2 [M+H]+: 503.2397; found 503.2396.
A 1:3 mixture of ynamides 26-S and 26-R (37.2 mg, 0.12 mmol) were prepared from the corresponding ynamide (52.7 mg, 0.47 mmol) and imine (Ss)-11a (109.1 mg, 0.52 mmol) in 25% yield.
26-S/R: Rf = 0.22 [EtOAc]; pale yellow oil; 1H NMR (400 MHz, CDCl3) [for 26-S/R] δ 1.19 (s, 9H), 1.21 (s, 27H), 3.69 (d, 3H, J = 4.0 Hz), 3.79 (d, 1H, J = 6.8 Hz), 3.90-3.94 (m, 8H), 4.39-4.43 (m, 8H), 5.36 (d, 1H, J = 6.8 Hz), 5.43 (d, 1H, J = 4.0 Hz), 7.30-7.39 (m, 12H), 7.52-7.56 (m, 8H); 13C NMR (100 MHz, CDCl3) [for 26-S/R] δ 22.6, 46.7, 46.8, 51.2, 51.3, 56.1, 56.5, 63.17, 63.24, 69.5, 70.2, 76.8, 127.7, 127.8, 128.4, 128.6, 128.7, 128.9, 138.7, 139.0, 156.0; IR (film) cm-1 [for 26-S/R] 2264m, 1765s, 1477m, 1417m, 1200m. mass spectrum (APCI): m/e (% relative intensity) 321 (100) (M+H)+; HRMS (ESI): m/z calcd for C16H20N2O3S [M+H]+: 321.1267; found 321.1266.
A 1:2 mixture of ynamides 28-S and 28-R (39.2 mg, 0.11 mmol) were prepared from the corresponding ynamide (52.5 mg, 0.38 mmol) and imine (Ss)-11a (87.5 mg, 0.42 mmol) in 30% yield.
28-S/R: Rf = 0.54 [acetone]; pale yellow oil; 1H NMR (500 MHz, CDCl3) [for 28-S/R] δ 1.20 (s, 9H), 1.21 (s, 18H), 2.00-2.05 (m, 6H), 2.98 (s, 9H), 3.25-3.30 (m, 6H), 3.57 (d, 3H, J = 4.5 Hz), 3.68 (t, 6H, J = 5.7 Hz), 5.36 (d, 1H, J = 6.5 Hz), 5.43 (d, 2H, J = 4.0 Hz), 7.27-7.38 (m, 9H), 7.39-7.60 (m, 6H); 13C NMR (125 MHz, CDCl3) [for 28-S/R] δ 14.9, 21.80, 21.83, 22.7, 34.5, 36.2, 47.97, 47.90, 50.0, 50.2, 51.7, 51.8, 56.0, 56.3, 66.5, 67.3, 83.1, 127.91, 127.92, 128.1, 128.2, 128.6, 128.8, 139.6, 139.9, 153.9; IR (film) cm-1 [for 28-S/R] 2958brm, 2254m, 1668s, 1498m, 1441m, 1407m, 1319m; mass spectrum (ESI): m/e (% relative intensity) 348 (100) (M+H)+; HRMS (ESI): m/z calcd for C18H26N3O2S [M+H]+: 348.1741; found 348.1746.
General Procedure for Additions with BF3-OEt2.
To a flamed-dried vial were charged with ynamide 13a (135.2 mg, 0.47 mmol) and THF (1.58 mL, ynamide concn = 0.3 M). To this solution at -78 oC was added LHMDS (0.71 mL, 1.0 M in THF). After the mixture was stirred at -78 oC for 1.0 h, a solution of imine (Ss)-11a (109.1 mg, 0.52 mmol) in THF (1 mL) pre-treated with BF3-Et2O (0.070 mL, 0.59 mmol) was added over 1 min. The resulting mixture was warmed to rt slowly, stirred overnight (~15 h), and monitored using TLC analysis. After complete consumption of the starting material, re-cooling of the mixture to -78 oC, H2O (2 mL) was added to quench the reaction. The quenched mixture was extracted with equal volume of EtOAc, dried over Na2SO4, filtered, and concentrated under reduced pressure. The crude residue was purified using silica gel flash column chromatography [Gradient eluent: 8:1~1:1 Hex:EtOAc + 3% Et3N] to afford 14-R (234.4 mg, 0.47 mmol) in ≥95% yield.
14-R: Rf = 0.30 [1:1 Hexane/EtOAc]; pale yellow oil; 1H NMR (400 MHz, CDCl3) δ 1.13 (s, 9H), 2.43 (s, 3H), 3.32 (d, 1H, J = 4.0 Hz), 4.45 (d, 1H, J = 13.6 Hz), 4.55 (d, 1H, J = 14.0 Hz), 5.30 (d, 1H, J = 4.0 Hz), 7.24-7.31 (m, 12H), 7.70 (d, 2H, J = 8.4 Hz); 13C NMR (100 MHz, CDCl3) δ 21.8, 22.6, 51.5, 55.5, 56.0, 69.8, 80.2, 127.8, 128.0, 128.5, 128.6, 128.7, 128.9, 129.1, 129.9, 134.5, 134.7, 139.3, 144.8; IR (film) cm-1 2250m, 1598m, 1455m, 1365s, 1169s; mass spectrum (APCI): m/e (% relative intensity) 495 (100) (M+H)+; HRMS (ESI): m/z calcd for C27H31N2O3S2 [M+H]+: 495.1771; found 495.1751.
Ynamide 15-R (225.0 mg, 0.44 mmol) were prepared from the corresponding ynamide (142.8 mg, 0.47 mmol) and imine (Ss)-11a (109.1 mg, 0.52 mmol) in 93% yield.
15-R: Rf = 0.18 [1:1 Hexane/EtOAc]; pale yellow solid; mp 104–105 °C; 1H NMR (500 MHz, CDCl3) δ 1.12 (s, 9H), 3.40 (d, 1H, J = 3.5 Hz), 3.83 (s, 3H), 4.44 (d, 1H, J = 14.0 Hz), 4.54 (d, 1H, J = 14.0 Hz), 5.29 (d, 1H, J = 3.5 Hz), 6.90 (d, 2H, J = 9.0 Hz), 7.28 (d, 10H, J = 8.0 Hz), 7.74 (d, 2H, J = 9.0 Hz); 13C NMR (125 MHz, CDCl3) δ 22.5, 51.3, 55.3, 55.7, 55.8, 69.7, 80.3, 114.3, 127.6, 128.3, 128.4, 128.5, 128.7, 128.9, 129.0, 130.0, 134.4, 139.2, 163.7; IR (film) cm-1 2251m, 1595m, 1497m, 1364m, 1262m, 1163s; mass spectrum (ESI): m/e (% relative intensity) 511 (100) (M+H)+; HRMS (ESI): m/z calcd for C27H31N2O4S2 [M+H]+: 511.1720; found 511.1702.
Ynamide 16-R (197.0 mg, 0.40 mmol) were prepared from the corresponding ynamide (135.0 mg, 0.47 mmol) and imine (Ss)-11a (109.1 mg, 0.52 mmol) in 84% yield.
16-R: Rf = 0.16 [7:3 Petroleum Ether/ EtOAc]; mp 84-85 oC; 1H NMR (600 MHz CDCl3) 1.14 (s, 9H), 3.37 (d, 1H, J= 3.6 Hz), 4.49 (d, 1H, J = 13.8 Hz), 4.58 (d, 1H, J = 13.8 Hz), 5.31 (d, 1H, J = 3.6 Hz), 7.09 (t, J = 8.4 Hz), 7.27-7.34 (m, 10H, ), 7.77-7.79 (m, 2H); 13C NMR (150 MHz, CDCl3) 22.5, 51.2, 55.6, 55.9, 69.9, 79.9, 116.3 (d, 2JF-C = 22.5 Hz), 127.6, 128.47, 128.53, 128.6, 128.8, 128.9, 130.6 (d, 3JF-C = 9.5 Hz), 133.6 (d, 4JF-C = 3.0 Hz), 134.1, 139.0, 165.6 (d, 1JF-C = 254.9 Hz); IR (KBr) cm-1 2247w, 1590m, 1493m, 1366s, 1241m, 1178s; mass spectrum (ESI): m/e (% relative intensity) 521 (100) (M + Na)+.
Ynamide 17-R (62.3 mg, 0.12 mmol) were prepared from the corresponding ynamide (39.7 mg, 0.13 mmol) and imine (Ss)-11a (28.9 mg, 0.14 mmol) in 91% yield.
17-R: Rf = 0.31 [1:1 Hexane/EtOAc]; pale yellow oil; 1H NMR (400 MHz, CDCl3) δ 1.16 (s, 9H), 3.45 (s, 1H), 4.55 (d, 1H, J = 14.0 Hz), 4.63 (d, 1H, J = 14.0 Hz), 5.33 (d, 1H, J = 3.6 Hz), 7.26-7.34 (m, 12H), 7.88 (d, 2H, J = 8.4 Hz), 8.19 (d, 2H, J = 8.4 Hz). 13C NMR (100 MHz, CDCl3) δ 22.6, 51.0, 56.1, 56.2, 70.4, 79.6, 124.3, 127.7, 128.8, 128.85, 128.88, 129.0, 129.08, 129.12, 133.8, 139.0, 143.0, 150.5; IR (film) cm-1 2251m, 1531s, 1366m, 1349s, 1313m, 1174s; mass spectrum (ESI): m/e (% relative intensity) 526 (100) (M+H)+; HRMS (ESI): m/z calcd for C26H28N3O5S2 [M+H]+: 526.1465; found 526.1443.
Ynamide 18-R (114.3 mg, 0.24 mmol) were prepared from the corresponding ynamide (65.8 mg, 0.24 mmol) and imine (Ss)-11a (55.9 mg, 0.27 mmol) in ≥95% yield.
18-R: Rf = 0.24 [1:1 Hexane/EtOAc]; pale yellow solid; mp 84–85 °C; 1H NMR (500 MHz, CDCl3) δ 1.18 (s, 9H), 2.40 (s, 3H), 3.48 (d, 1H, J = 4.0 Hz), 5.42 (d, 1H, J = 3.5 Hz), 7.19-7.40 (m, 11H), 7.50-7.51 (m, 4H); 13C NMR (125 MHz, CDCl3) δ 21.8, 22.7, 51.5, 56.1, 68.9, 80.6, 126.2, 127.9, 128.35, 128.40, 128.7, 129.0, 129.2, 129.6, 133.0, 138.7, 139.4, 145.0; IR (film) cm-1 2251m, 1595m, 1491m, 1455m, 1371s; mass spectrum (ESI): m/e (% relative intensity) 481 (100) (M+H)+; HRMS (ESI): m/z calcd for C26H29N2O3S2 [M+H]+: 481.1615; found 481.1631.
Ynamide 20-R (112.1 mg, 0.28 mmol) were prepared from the corresponding ynamide (56.6 mg, 0.30 mmol) and imine (Ss)-11a (68.9 mg, 0.33 mmol) in 94% yield.
20-R: Rf = 0.17 [1:1 Hexane/EtOAc]; pale yellow oil; 1H NMR (400 MHz, CDCl3) δ 1.16 (s, 9H), 3.47 (d, 1H, J = 4.0 Hz), 3.78 (s, 3H), 4.59 (d, 1H, J = 14.4 Hz), 4.65 (d, 1H, J = 14.8 Hz), 5.37 (d, 1H, J = 4.0 Hz), 7.28-7.33 (m, 8H), 7.35-7.40 (m, 2H). 13C NMR (100 MHz, CDCl3) δ 22.5, 51.4, 53.7, 54.1, 55.9, 69.3, 80.4, 127.7, 128.1, 128.4, 128.6, 128.7, 135.9, 139.5, 155.8; IR (film) cm-1 2259m, 1724s, 1444m, 1365m, 1286m, 1221m; mass spectrum (ESI): m/e (% relative intensity) 399 (100) (M+H)+; HRMS (ESI): m/z calcd for C22H27N2O3S [M+H]+: 399.1737; found 399.1724.
Ynamide 21-R (208.4 mg, 0.43 mmol) were prepared from the corresponding ynamide (132.3 mg, 0.47 mmol) and imine 11a (109.1 mg, 0.52 mmol) in 90% yield.
21-R: Rf = 0.28 [1:1 CH2Cl2/EtOAc]; white solid; mp 143–144 °C; 1H NMR (400 MHz, CDCl3) δ 0.92 (s, 3H), 1.09 (s, 3H), 1.16 (s, 9H), 3.41 (d, 1H, J = 3.2 Hz), 4.05-4.12 (m, 4H), 4.42-4.50 (m, 2H), 5.29 (t, 1H, J = 3.0 Hz), 7.28-7.40 (m, 10H); 13C NMR (100 MHz, CDCl3) δ 21.0, 21.4, 22.6, 32.1 (d, J = 6.9 Hz), 51.1, 54.8 (d, J = 6.9 Hz), 55.8, 64.6 (d, J = 5.4 Hz), 78.4 (dd, J = 6.9, 19.1 Hz), 82.8 (d, J = 5.3 Hz), 127.5, 128.1, 128.4, 128.5, 128.78, 128.84, 136.2 (d, J = 2.3 Hz), 139.6; 31P NMR (162 MHz, CDCl3) δ -1.81; IR (film) cm-1 2962brw, 2249m, 1456m, 1281m, 1060s; mass spectrum (ESI): m/e (% relative intensity) 489 (100) (M+H)+; HRMS (ESI): m/z calcd for C25H34N2O4PS [M+H]+: 489.1972; found 489.1970.
Ynamide 23-R (258.0 mg, 0.49 mmol) were prepared from the corresponding ynamide (135.2 mg, 0.47 mmol) and imine (Ss)-11b (124.8 mg, 0.52 mmol) in ≥95% yield.
23-R: Rf = 0.15 [1:1 Hexane/EtOAc]; pale yellow oil; 1H NMR (400 MHz, CDCl3) δ 1.09 (s, 9H), 2.38 (s, 3H), 3.34 (d, 1H, J = 3.6 Hz), 3.73 (s, 3H), 4.41 (d, 1H, J = 14.0 Hz), 4.51 (d, 1H, J = 14.0 Hz), 5.22 (d, 1H, J = 3.6 Hz), 6.77 (d, 2H, J = 8.4 Hz), 7.17-7.25 (m, 9H), 7.69 (d, 2H, J = 8.0 Hz); 13C NMR (100 MHz, CDCl3) δ 21.5, 22.37, 22.45, 50.7, 55.2, 55.3, 55.7, 69.8, 79.7, 113.9, 127.7, 128.2, 128.5, 128.77, 128.84, 129.7, 131.2, 134.3, 134.4, 144.6, 159.5; IR (film) cm-1 2249w, 1611w, 1511m, 1364m, 1248m, 1169s; mass spectrum (ESI): m/e (% relative intensity) 525 (100) (M+H)+; HRMS (ESI): m/z calcd for C28H33N2O4S2 [M+H]+: 525.1877; found 525.1902.
Ynamide 24-R (213.6 mg, 0.43 mmol) were prepared from the corresponding ynamide (135.2 mg, 0.47 mmol) and imine (Ss)-11c (112.3 mg, 0.52 mmol) in 90% yield.
24-R: Rf = 0.20 [1:1 Hexane/EtOAc]; white solid; mp 92–93 °C; 1H NMR (400 MHz, CDCl3) δ 0.84-1.07 (m, 5H), 1.12 (s, 9H), 1.44-1.49 (m, 1H), 1.55-1.68 (m, 5H), 2.43 (s, 3H), 3.15 (d, 1H, J = 5.2 Hz), 3.99 (t, 1H, J = 5.0 Hz), 4.42 (d, 1H, J = 14.0 Hz), 4.54 (d, 1H, J = 14.0 Hz), 7.28-7.32 (m, 7H), 7.76 (d, 2H, J = 8.0 Hz); 13C NMR (100 MHz, CDCl3) δ 21.6, 22.5, 25.7, 25.9, 26.1, 27.5, 29.6, 43.8, 53.4, 55.4, 55.8, 69.2, 78.8, 127.8, 128.3, 128.5, 128.9, 129.7, 134.5, 134.6, 144.6; IR (film) cm-1 2925m, 2852w, 2251w, 1452m, 1363m, 1168s; mass spectrum (ESI): m/e (% relative intensity) 501 (100) (M+H)+; HRMS (ESI): m/z calcd for C27H37N2O3S2 [M+H]+: 501.2241; found 501.2234.
Ynamide 25-R (186.7 mg, 0.37 mmol) were prepared from the corresponding ynamide (114.1 mg, 0.40 mmol) and imine (Ss)-11b (95.6 mg, 0.44 mmol) in 93% yield.
25-R: Rf = 0.25 [1:1 Hexane/EtOAc]; white solid; mp 69–70 °C; 1H NMR (400 MHz, CDCl3) δ 0.88 (t, 3H, J = 7.0 Hz), 1.13 (s, 9H), 1.21-1.29 (m, 8H), 1.50-1.67 (m, 2H), 2.43 (s, 3H), 3.12 (d, 1H, J = 5.2 Hz), 4.09-4.14 (m, 1H), 4.42 (d, 1H, J = 13.6 Hz), 4.52 (d, 1H, J = 14.0 Hz), 7.24-7.31 (m, 7H), 7.75 (d, 2H, J = 8.4 Hz); 13C NMR (100 MHz, CDCl3) δ 14.1, 21.7, 22.5, 22.6, 25.6, 28.8, 31.7, 37.5, 48.4, 55.5, 55.7, 70.6, 78.1, 127.8, 128.3, 128.5, 128.9, 129.7, 134.5, 134.7, 144.6; IR (film) cm-1 2925m, 2857m, 2250w, 1597w, 1456m, 1364m, 1168s; mass spectrum (ESI): m/e (% relative intensity) 503 (100) (M+H)+; HRMS (ESI): m/z calcd for C27H39N2O3S2 [M+H]+: 503.2397; found 503.2393.
Ynamide 26-R (145.2 mg, 0.45 mmol) were prepared from the corresponding ynamide (52.7 mg, 0.47 mmol) and imine (Ss)-11a (109.1 mg, 0.52 mmol) in ≥95% yield.
26-R: Rf = 0.22 [EtOAc]; pale yellow solid; mp 131–132 °C; 1H NMR (400 MHz, CDCl3) δ 1.21 (s, 9H), 3.69 (d, 1H, J = 4.0 Hz), 3.90-3.94 (m, 2H), 4.41 (t, 2H, J = 8.0 Hz), 5.43 (d, 1H, J = 4.0 Hz), 7.30-7.39 (m, 3H), 7.54 (d, 2H, J = 7.2 Hz); 13C NMR (100 MHz, CDCl3) δ 22.6, 46.8, 51.2, 56.1, 63.2, 69.5, 76.8, 127.8, 128.6, 128.9, 139.0, 156.0; IR (film) cm-1 2979brw, 2924w, 2264m, 1764s, 1477m, 1418m, 1201m; mass spectrum (ESI): m/e (% relative intensity) 321 (100) (M+H)+; HRMS (ESI): m/z calcd for C16H21N2O3S [M+H]+: 321.1273; found 321.1279.
Ynamide 27-R (24.3 mg, 0.07 mmol) were prepared from the corresponding ynamide (59.3 mg, 0.47 mmol) and imine (Ss)-11a (109.1 mg, 0.52 mmol) in 15% yield.
27-R: Rf = 0.43 [1:1 acetone/EtOAc]; pale yellow oil; 1H NMR (400 MHz, CDCl3) δ 1.21 (s, 9H), 2.08-2.13 (m, 2H), 3.64 (d, 1H, J = 4.0 Hz), 3.77 (t, 2H, J = 6.2 Hz), 4.33 (t, 2H, J = 5.4 Hz), 5.43 (d, 1H, J = 4.4 Hz), 7.28-7.39 (m, 3H), 7.57 (d, 2H, J = 7.2 Hz); 13C NMR (100 MHz, CDCl3) δ 21.6, 22.7, 50.0, 51.3, 56.2, 67.7, 68.1, 81.2, 128.0, 128.6, 128.9, 139.2, 151.8; IR (film) cm-1 2955brw, 2265w, 1721s, 1476m, 1423m, 1278m; mass spectrum (ESI): m/e (% relative intensity) 335 (100) (M+H)+; HRMS (ESI): m/z calcd for C17H23N2O3S [M+H]+: 335.1424; found 335.1417.
Ynamide 28-R (42.1 mg, 0.12 mmol) were prepared from the corresponding ynamide (52.5 mg, 0.38 mmol) and imine (Ss)-11a (87.5 mg, 0.42 mmol) in 32% yield.
28-R: Rf = 0.54 [acetone]; pale yellow oil; 1H NMR (400 MHz, CDCl3) δ 2.17 (s, 9H), 1.99 (pent, 2H, J = 5.8 Hz), 2.94 (s, 3H), 3.25 (t, 2H, J = 5.8 Hz), 3.63-3.66 (m, 3H), 5.40 (d, 1H, J = 4.8 Hz), 7.23-7.34 (m, 3H), 7.55 (d, 2H, J = 7.2 Hz); 13C NMR (100 MHz, CDCl3) δ 21.8, 22.7, 36.2, 47.9, 50.2, 51.7, 56.0, 66.6, 83.2, 127.9, 128.2, 128.8, 139.9, 154.0; IR (film) cm-1 2955m, 2257w, 1662s, 1498s, 1441m, 1408m, 1319m, 1207m; mass spectrum (ESI): m/e (% relative intensity) 348 (100) (M+H)+; HRMS (ESI): m/z calcd for C18H26N3O2S [M+H]+: 348.1741; found 348.1728.
Ynamide 29 (233.2 mg, 0.47 mmol) were prepared from the corresponding ynamide (135.2 mg, 0.47 mmol) and imine (Rs)-11a (109.1 mg, 0.52 mmol) in ≥95% yield.
29: Rf = 0.30 [1:1 Hexane/EtOAc]; colorless oil; 1H NMR (500 MHz, CDCl3) δ 1.13 (s, 9H), 2.41 (s, 3H), 3.35 (d, 1H, J = 4.0 Hz), 4.45 (d, 1H, J = 13.5 Hz), 4.54 (d, 1H, J = 14.0 Hz), 5.29 (d, 1H, J = 4.0 Hz), 7.24-7.29 (m, 12H), 7.70 (d, 2H, J = 8.0 Hz); 13C NMR (125 MHz, CDCl3) δ 21.7, 22.5, 51.4, 55.4, 55.9, 69.7, 80.1, 127.7, 127.8, 128.4, 128.5, 128.6, 128.8, 129.0, 129.8, 134.4, 134.6, 139.1, 144.7; IR (film) cm-1 2250m, 1598w, 1494w, 1455m, 1363s, 1167s; mass spectrum (ESI): m/e (% relative intensity) 495 (100) (M+H)+; HRMS (ESI): m/z calcd for C27H31N2O3S2 [M+H]+: 495.1771; found 495.1756.
Ynamide 30-R (188.0 mg, 0.47 mmol) were prepared from the corresponding ynamide (88.7 mg, 0.47 mmol) and imine (Ss)-11a (109.1 mg, 0.52 mmol) in ≥95% yield.
30-R: Rf = 0.49 [1:1 CH2Cl2/EtOAc]; pale yellow oil; 1H NMR (400 MHz, CDCl3) δ 1.10 (s, 9H), 3.40 (d, 1H, J = 4.4 Hz), 4.24 (dd, 1H, J = 7.2, 8.8 Hz), 4.72 (t, 1H, J = 8.8 Hz), 5.06 (t, 1H, J = 7.8 Hz), 5.27 (d, 1H, J = 4.0 Hz), 7.25-7.30 (m, 5H), 7.35-7.37 (m, 2H), 7.43-7.45 (m, 3H); 13C NMR (100 MHz, CDCl3) δ 22.4, 51.3, 55.9, 62.0, 70.8, 71.1, 75.6, 127.1, 127.6, 128.5, 128.8, 129.4, 129.6, 136.0, 139.0, 155.5; IR (film) cm-1 2267m, 1773s, 1475m, 1457m, 1409m, 1181m; mass spectrum (ESI): m/e (% relative intensity) 397 (100) (M+H)+; HRMS (ESI): m/z calcd for C22H25N2O3S [M+H]+: 397.1581; found 397.1580.
Ynamide 31 (183.9 mg, 0.46 mmol) were prepared from the corresponding ynamide (88.7 mg, 0.47 mmol) and imine (Ss)-11a (109.1 mg, 0.52 mmol) in ≥95% yield.
31: Rf = 0.26 [1:1 CH2Cl2/EtOAc]; white solid; mp 150–151 °C; 1H NMR (400 MHz, CDCl3) δ 1.09 (s, 9H), 3.48 (d, 1H, J = 4.4 Hz), 4.27 (dd, 1H, J = 7.2, 9.2 Hz), 4.74 (t, 1H, J = 9.0 Hz), 5.10 (dd, 1H, J = 7.2, 8.8 Hz), 5.29 (d, 1H, J = 4.4 Hz), 7.23-7.24 (m, 5H), 7.37-7.39 (m, 2H), 7.44-7.46 (m, 3H); 13C NMR (100 MHz, CDCl3) δ 22.4, 51.4, 55.9, 62.0, 70.8, 71.3, 75.7, 127.3, 127.7, 128.4, 128.7, 129.4, 129.6, 136.1, 138.6, 155.5; IR (film) cm-1 2267m, 1773s, 1457m, 1406m, 1182m; mass spectrum (APCI): m/e (% relative intensity) 397 (42) (M+H)+; HRMS (ESI): m/z calcd for C22H25N2O3S [M+H]+: 397.1581; found 397.1598.
General Procedure for Acid Promoted 5-endo-dig Cyclization.
To a vial were added ynamide 30-R (113.2 mg, 0.29 mmol), THF (0.50 mL), H2O (0.050 mL), and HOAc (0.050 mL). The vial was sealed and heated to 85 oC. When the reaction was judged to be complete by TLC after 14.5 h, the mixture was cooled to rt and concentrated under reduced pressure. The crude residue was purified using silica gel flash column chromatography [Two isocratic eluent: (a) 4:1 Hexane/EtOAc and then (b) 1:1 Acetone/EtOAc] to afford 32 (23.0 mg, 0.07 mmol) in 25% yield and 33 (29.7 mg, 0.09 mmol) in 31% yield.
32: Rf = 0.23 [3:1 Hexane/EtOAc]; white solid; mp 207-208 °C. 1H NMR (400 MHz, CDCl3) δ 4.38 (dd, 1H, J = 5.6, 8.8 Hz), 4.95 (t, 1H, J = 9.0 Hz), 5.36 (dd, 1H, J = 5.6, 9.2 Hz), 6.59 (s, 1H), 7.33-7.46 (m, 8H), 7.67-7.69 (m, 2H); 13C NMR (100 MHz, CDCl3) δ 61.4, 71.8, 106.2, 126.4, 126.7, 128.8, 129.3, 129.9, 130.0, 134.8, 136.7, 155.3, 160.8, 164.7; IR (film) cm-1 1745s, 1539m, 1389m, 1208m; mass spectrum (ESI): m/e (% relative intensity) 323 (100) (M+H)+; HRMS (ESI): m/z calcd for C18H15N2O2S [M+H]+: 323.0849; found 323.0854.
33: Rf = 0.11 [1:1 CH2Cl2/EtOAc]; white solid; mp 198-199 °C. 1H NMR (500 MHz, DMSO) δ 3.30 (dd, 1H, J = 3.5, 8.5 Hz), 3.95 (t, 1H, J = 9.0 Hz), 4.41 (dd, 1H, J = 3.5, 8.5 Hz), 4.51 (s, 1H), 4.63 (s, 1H), 5.95-5.97 (m, 2H), 6.25-6.45 (m, 8H); 13C NMR (100 MHz, DMSO) δ 60.8, 67.0, 71.2, 117.5, 126.4, 127.3, 128.2, 128.6, 128.8, 129.3, 138.1, 138.6, 144.8, 154.0; IR (film) cm-1 2959w, 2927w, 1768m, 1714s, 1645m, 1402m, 1187m; mass spectrum (ESI): m/e (% relative intensity) 341 (100) (M+H)+; HRMS (ESI): m/z calcd for C18H17N2O3S [M+H]+: 341.0955; found 341.0954.
34: Rf = 0.14 [1:1 Hexane/EtOAc]; white solid; mp 159-160 °C. 1H NMR (500 MHz, CDCl3) δ 4.24 (dd, 1H, J = 6.5, 8.5 Hz), 4.64 (s, 1H), 4.83 (t, 1H, J = 8.7 Hz), 5.35 (dd, 1H, J = 6.5, 9.0 Hz), 5.69 (s, 1H), 5.91 (d, 1H, J = 1.0 Hz), 7.10-7.12 (m, 2H), 7.24-7.46 (m, 8H); 13C NMR (125 MHz, CDCl3) δ 60.1, 69.3, 71.0, 118.6, 126.6, 127.3, 129.1, 129.2, 129.6, 129.8, 136.4, 137.8, 143.6, 154.9; IR (film) cm-1 2926w, 1745s, 1462m, 1373m, 1241s; mass spectrum (ESI): m/e (% relative intensity) 341 (100) (M+H)+; HRMS (ESI): m/z calcd for C18H17N2O3S [M+H]+: 341.0955; found 341.0946.
35: Rf = 0.70 [1:1 CH2Cl2/EtOAc]; white solid; 180 °C (decomposed). 1H NMR (500 MHz, DMSO) δ 3.37 (t, 2H, J = 8.0 Hz), 3.83 (t, 2H, J = 8.0 Hz), 6.61-6.68 (m, 4H), 7.20 (d, 2H, J = 7.0 Hz); 13C NMR (125 MHz, DMSO) δ 45.4, 64.2, 105.8, 126.5, 128.8, 129.3, 134.3, 155.3, 161.5, 164.0; IR (film) cm-1 2918brm, 1740s, 1542w, 1373m, 1240s; mass spectrum (ESI): m/e (% relative intensity) 247 (100) (M+H)+; HRMS (ESI): m/z calcd for C12H11N2O2S [M+H]+: 247.0536; found 247.0529.
36: Rf = 0.13 [1:1 CH2Cl2/EtOAc]; white solid; mp 190-191 °C. 1H NMR (400 MHz, DMSO) δ 2.99 (dd, 1H, J = 7.6, 16.8 Hz), 3.18 (dd, 1H, J = 8.8, 17.6 Hz), 3.67 (t, 2H, J = 8.0 Hz), 4.93 (t, 1H, J = 1.6 Hz), 5.22 (dd, 1H, J = 1.2, 2.0 Hz), 6.43-6.56 (m, 5H); 13C NMR (100 MHz, DMSO) δ 45.3, 63.0, 67.1(d, J = 1.5 Hz), 115.7 (d, J = 7.6 Hz), 127.3, 128.1, 128.6, 139.0, 145.0, 154.0; IR (film) cm-1 2984w, 2916w, 1739s, 1373m, 1239s, 1046m; mass spectrum (ESI): m/e (% relative intensity) 265 (100) (M+H)+; HRMS (ESI): m/z calcd for C12H13N2O3S [M+H]+: 265.0642; found 265.0653.
ACKNOWLEDGEMENTS
We thank the NIH [GM066055] for funding and Dr. Victor Young of The University of Minnesota for X-ray structural data.
References
1. For current leading reviews on ynamides, see (a) K. A. DeKorver, H. Li, A. G. Lohse, R. Hayashi, Z. Lu, Y. Zhang, and R. P. Hsung, Chem. Rev., 2010, 110, 5064; CrossRef (b) G. Evano, A. Coste, and K. Jouvin, Angew. Chem. Int. Ed., 2010, 49, 2840. CrossRef
2. For reviews partially accounting chemistry of ynamides, see: (a) L. Ackermann and H. K. Potukuchi, Org. Biomol. Chem., 2010, 8, 4503; CrossRef (b) G. Domínguez and J. Perez-Castells, Chem. Soc. Rev., 2011, 40, 3430; CrossRef (c) N. Weding and M. Hapke, Chem. Soc. Rev., 2011, 40, 4525; CrossRef (d) C. Madelaine, V. Valerio, and N. Maulide, Chem. Asian J., 2011, 6, 2224. CrossRef
3. For reviews on syntheses of ynamides, see: (a) J. A. Mulder, K. C. M. Kurtz, and R. P. Hsung, Synlett, 2003, 1379; CrossRef (b) J. R. Dehli, J. Legros, and C. Bolm, Chem. Commun., 2005, 43, 973; CrossRef (c) M. R. Tracey, R. P. Hsung, J. A. Antoline, K. C. M. Kurtz, L. Shen, B. W. Slafer, and Y. Zhang, in Science of Synthesis, Houben-Weyl Methods of Molecular Transformations, ed. by Steve M. Weinreb, Georg Thieme Verlag KG: Stuttgart, Germany, Chapter 21.4, 2005; (d) G. Evano, N. Blanchard, and M. Toumi, Chem. Rev., 2008, 108, 3054; CrossRef (e) G. Evano, K. Jouvinb, and A. Coste, Synthesis, 2013, 45, 17. CrossRef
4. Given the volume, for ynamide chemistry published since September of 2012, see: (a) R. Karmakar, P. Mamidipalli, S. Y. Yun, and D. Lee, Org. Lett., 2013, 15, 1938; CrossRef (b) J. Brioche, C. Meyer, and J. Cossy, Org. Lett., 2013, 15, 1626; CrossRef (c) S. Y. Yun, K.-P. Wang, N.-K. Lee, P. Mamidipalli, and D. Lee, J. Am. Chem. Soc., 2013, 135, 4668; CrossRef (d) S. J. Heffernan, J. M. Beddoes, M. F. Mahon, A. J. Hennessy, and D. R. Carbery, Chem. Commun., 2013, 49, 2314; CrossRef (e) Y. Kong, K. Jiang, J. Cao, L. Fu, L. Yu, G. Lai, Y. Cui, Z. Hu, and G. Wang, Org. Lett., 2013, 15, 422; CrossRef (f) I. Yavari, M. Nematpour, and E. Sodagar, Synlett, 2013, 24, 161; CrossRef (g) I. Yavari and M. Nematpour, Synlett, 2013, 24, 165; CrossRef (h) S. Bhunia, C.-J. Chang, and R.-S. Liu, Org. Lett., 2012, 14, 5522; CrossRef (i) Y. Minko, M. Pasco, L. Lercher, M. Botoshansky, and I. Marek, Nature, 2012, 490, 522; CrossRef (j) T. R. Hoye, B. Baire, D. Niu, P. H. Willoughby, and B. P. Woods, Nature, 2012, 490, 208; CrossRef (k) J. Cao, Y. Kong, Y. Deng, G. Lai, Y. Cui, Z. Hu, and G. Wang, Org. Biomol. Chem., 2012, 10, 9556; CrossRef (l) R. L. Greenaway, C. D. Campbell, H. A. Chapman, and E. A. Anderson, Adv. Synth. Catal., 2012, 354, 3187; CrossRef (m) Z. Jiang, P. Lu, and Y. Wang, Org. Lett., 2012, 14, 6266; CrossRef (n) W. Gati, M. M. Rammah, M. B. Rammah, and G. Evano, Beilstein J. Org. Chem., 2012, 8, 2214; CrossRef (o) D. L. Smith, S. R. Chidipudi, W. R. Goundry, and H. W. Lam, Org. Lett., 2012, 14, 4934; CrossRef (p) S. J. Heffernan and D. R. Carbery, Tetrahedron Lett., 2012, 53, 5180. CrossRef
5. For accounts on syntheses of ynamides published since 2012, see: (a) X. Jin, K. Yamaguchi, and N. Mizuno, Chem. Lett., 2012, 41, 866; CrossRef (b) X. Jin, K. Yamaguchi, and N. Mizuno, Chem. Commun., 2012, 48, 4974; CrossRef (c) K. Jouvin, J. Heimburger, and G. Evano, Chem. Sci., 2012, 3, 756; CrossRef (d) K. Jouvin, A. Coste, A. Bayle, F. Legrand, G. Karthikeyan, K. Tadiparthi, and G. Evano, Organometallics, 2012, 31, 7933; CrossRef (e) X. Tong, G. Ni, X. Deng, and C. Xia, Synlett, 2012, 23, 2497; CrossRef (f) M.-G. Wang, J. Wu, and Z.-C. Shang, Synlett, 2012, 23, 589; CrossRef (g) A. Laouiti, K. Jouvin, F. Bourdreux, M. M. Rammah, M. B. Rammah, and G. Evano, Synthesis, 2012, 44, 1491. CrossRef
6. For syntheses of novel structural analogs of ynamides, see: For syntheses of novel structural analogs of ynamides, see: (a) Yne-Sulfoxyimines: L. Wang, H. Huang, D. L. Priebbenow, F.-F. Pan, and C. Bolm, Angew. Chem. Int. Ed., 2013, 52, 3478; CrossRef (b) Yne-hydrazides: R. E. Beveridge and R. A. Batey, Org. Lett., 2012, 14, 540; CrossRef (c) Yne-imines: A. Laouiti, M. M. Rammah, M. B. Rammah, J. Marrot, F. Couty, and G. Evano, Org. Lett., 2012, 14, 6; CrossRef (d) Ynimides: J. A. Souto, P. Becker, Á. Iglesias, and K. Muñiz, J. Am. Chem. Soc., 2012, 134, 15505; CrossRef (e) Ynimides: T. Sueda, A. Oshima, and N. Teno, Org. Lett., 2011, 13, 3996; CrossRef (f) Ynimides: T. Sueda, A. Kawada, Y. Urashi, and N. Teno, Org. Lett., 2013, 15, 1560; CrossRef (g) diamino-acetylenes: A. R. Petrov, C. G. Daniliuc, P. G. Jones, and M. Tamm, Chem.-Eur. J., 2010, 16, 11804; CrossRef (h) Amidinyl-ynamides: J. Li and L. Neuville, Org. Lett., 2013, 15, 1752. CrossRef
7. K. A. DeKorver, R. P. Hsung, W.-Z. Song, M. C. Walton, and X.-N. Wang, Org. Lett., 2012, 14, 3214. CrossRef
8. For an example of coupling using unprotected secondary propargylic amines, see: A. S. K. Hashmi, A. M. Schuster, M. Zimmer, and F. Rominger, Chem. Eur. J., 2011, 17, 5511. CrossRef
9. For reviews, see: (a) M. T. Robak, M. A. Herbage, and J. A. Ellman, Chem. Rev., 2010, 110, 3600; CrossRef (b) F. Ferreira, C. Botuha, F. Chemla, and A. Perez-Luna, Chem. Soc. Rev., 2009, 38, 1162; CrossRef (c) G.-Q. Lin, M.-H. Xu, Y.-W. Zhong, and X.-W. Sun, Acc. Chem. Res., 2008, 41, 831; CrossRef (d) D. Morton and R. A. Stockman, Tetrahedron, 2006, 62, 8869; CrossRef (e) P. Zhou, B.-C. Chen, and F. A. Davis, Tetrahedron, 2004, 60, 8003; CrossRef (f) J. A. Ellman, T. D. Owens, and T. P. Tang, Acc. Chem. Res., 2002, 35, 984; CrossRef A. E. Sorochinsky and V. A. Soloshonok, J. Fluorine Chem., 2010, 131, 127. CrossRef
10. For leading examples using metallated acetylides, see: (a) B. Wang and P.-P. Zhang, Tetrahedron Lett., 2012, 53, 119; CrossRef (b) L. Ye, W. He, and L. Zhang, Angew. Chem. Int. Ed., 2011, 50, 3236; CrossRef (c) B.-L. Chen, B. Wang, and G.-Q. Lin, J. Org. Chem., 2009, 75, 941; CrossRef (d) D. M. Hodgson, J. Kloesges, and B. Evans, Synthesis, 2009, 1923; CrossRef (e) S. S. Harried, M. D. Croghan, M. R. Kaller, P. Lopez, W. Zhong, R. Hungate, and P. J. Reider, J. Org. Chem., 2009, 74, 5975; CrossRef (f) A. Voituriez, A. Pérez-Luna, F. Ferreira, C. Botuha, and F. Chemla, Org. Lett., 2009, 11, 931; CrossRef (g) X.-Y. Chen, X.-L. Qiu, and F.-L. Qing, Tetrahedron, 2008, 64, 2301; CrossRef (h) S. Turcaud, F. Berhal, and J. Royer, J. Org. Chem., 2007, 72, 7893; CrossRef (i) A. W. Patterson and J. A. Ellman, J. Org. Chem., 2006, 71, 7110; CrossRef (j) F. A. Davis, M. B. Nolt, Y. Wu, K. R. Prasad, D. Li, B. Yang, K. Bowen, S. H. Lee, and J. H. Eardley, J. Org. Chem., 2005, 70, 2184; CrossRef (k) J. C. Barrow, P. L. Ngo, J. M. Pellicore, H. G. Selnick, and P. G. Nantermet, Tetrahedron Lett., 2001, 42, 2051; CrossRef (l) A. W. Shaw and S. J. deSolms, Tetrahedron Lett., 2001, 42, 7173; CrossRef (m) T. P. Tang, S. K. Volkman, and J. A. Ellman, J. Org. Chem., 2001, 66, 8772. CrossRef
11. For an elegant account on additions of lithiated ynol-ethers to Ellman-Davis chiral imines, see: (a) C. Verrier, S. Carret, and J.-F. Poisson, Org. Lett., 2012, 14, 5122. CrossRef
12. For additions of lithiated ynol-ethers to imines, see: (a) X. Y. Mak, R. P. Ciccolini, J. M. Robinson, J. W. Tester, and R. L. Danheiser, J. Org. Chem., 2009, 74, 9381; CrossRef (b) A. S. K. Hashmi, M. Rudolph, J. Huck, W. Frey, J. W. Bats, and M. Hamzic, Angew. Chem. Int. Ed., 2009, 48, 5848. CrossRef
13. (a) M. O. Frederick, J. A. Mulder, M. R. Tracey, R. P. Hsung, J. Huang, K. C. M. Kurtz, L. Shen, and C. J. Douglas, J. Am. Chem. Soc., 2003, 125, 2368; CrossRef (b) D. Rodriguez, M. F. Martinez-Esperon, L. Castedo, and C. Saá, Synlett, 2007, 1963; CrossRef (c) See Reference 6a.
14. For leading examples of related reversal of stereoselectivity, see: (a) J. Louvel, F. Chemla, E. Demont, F. Ferreira, A. Pérez-Luna, and A. Voituriez, Adv. Synth. Catal., 2011, 353, 2137; CrossRef (b) A. Viso, R. Fernández de la Pradilla, A. Flores, A. García, M. Tortosa, and M. L. López-Rodríguez, J. Org. Chem., 2006, 71, 1442; CrossRef (c) W. Jiang, C. Chen, D. Marinkovic, J. A. Tran, C. W. Chen, L. M. Arellano, N. S. White, and F. C. Tucci, J. Org. Chem., 2005, 70, 8924; CrossRef (d) S. D. Kuduk, R. M. DiPardo, R. K. Chang, C. Ng, and M. G. Bock, Tetrahedron Lett., 2004, 45, 6641; CrossRef (e) Also see reference 12.
15. D. A. Pflum, D. Krishnamurthy, Z. Han, S. A. Wald, and C. H. Senanayake, Tetrahedron Lett., 2002, 43, 923. CrossRef
16. Y. Bolshan and R. A. Batey, Org. Lett., 2005, 7, 1481. CrossRef
17. S.-T. Ruan, J.-M. Luo, Y. Du, and P.-Q. Huang, Org. Lett., 2011, 13, 4938. CrossRef