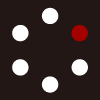
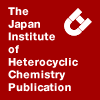
HETEROCYCLES
An International Journal for Reviews and Communications in Heterocyclic ChemistryWeb Edition ISSN: 1881-0942
Published online by The Japan Institute of Heterocyclic Chemistry
e-Journal
Full Text HTML
Received, 25th June, 2013, Accepted, 7th August, 2013, Published online, 19th August, 2013.
DOI: 10.3987/COM-13-S(S)47
■ Selective Preparation of 6- and 7-(Polyhydroxypropyl)pterins from Pentos-2-uloses
Tadashi Hanaya* and Kasumi Ito
Department of Chemistry, Faculty of Science, Okayama University, 3-1-1 Tsushima-naka, Okayama 700-8530, Japan
Abstract
The Gabriel-Isay condensation of three types of pentos-2-uloses with 2,5,6-triaminopyrimidin-4(3H)-one (3) was examined under both acidic and basic conditions. The condensation of 5-deoxy- and 5-O-protected pentofuranos-2-uloses with 3 at pH 8 afforded 6-substituted pterins as major isomers, whereas the same reaction at pH 3 yielded the 7-substituted pterins predominantly.Various pterin derivatives having a polyhydroxyalkyl side-chain at C-6 or C-7 have been isolated from many living organisms. Among them, biopterin (1a)1 is one of the most ubiquitous naturally occurring pterins and was isolated for the first time from human urine.2 Its 5,6,7,8-tetrahydro derivative exhibits enzyme cofactor activity in hydroxylation of aromatic amino acids3 and nitric oxide synthesis.4 In addition to 1a, primapterin (7-biopterin)5 (1b), neopterin (2a), and anapterin (7-neopterin)5 (2b) have also been isolated from the urine of patients with hyperphenylalaninemia.6 Neopterin (2a) has attracted attention as a marker for activation of cellular immunity or an inducer of apoptosis.7
The Gabriel-Isay reaction8 is one of the most effective methods to obtain the 6- and 7-substituted pteridines directly from the condensation of 5,6-diaminopyrimidines and 2-ketoaldehyde. As the condensation of the unsymmetrical diaminopyrimidines with unsymmetrical dicarbonyl compounds generally leads to the formation of regioisomers, various means of controlling a preponderant formation of the 6- or 7-substituted pterins have been attempted.9,10 For example, the pteridine ring formation of 2,5,6-triaminopyrimidin-4(3H)-one (3) with phenyl glyoxal under acidic conditions affords the 6-phenylpterin (4a), whereas the same condensation under basic conditions yields 7-phenylpterin (4b) preferentially (Scheme 1).10
The difference in the major product provided under these two conditions could be explained in terms of the combination of basicity of the relevant two amino groups (5- and 6-) of pyrimidine 3 and the higher electrophilicity of the aldehyde carbon (than that of the keto); under the basic conditions, the most nucleophilic 5-amino group of 3 would attack the aldehyde carbon, while under the acidic conditions an attack of the 6-amino group of 3 (owing to the protonation of the 5-amino group) to the aldehyde would preferentially take place.
With regard to preparation of (polyhydroxyalkyl)pterins (such as 1a,b, 2a,b) and their derivatives, the condensation of 3 and the appropriate sugar precursors (2-ketoaldoses) seems to be feasible. The 2-ketoaldoses, however, exist in an equilibrium between cyclic hemiacetal and acyclic aldehyde forms and therefore it is difficult to predict which carbonyl carbon reacts initially. Although the Gabriel-Isay reaction has been used for preparation of simple 6- and 7-alkylpterins well, the application of it to (polyhydroxyalkyl)pterin syntheses has scarcely been reported so far.11 We now report a systematic investigation of the regioselectivity and synthetic efficiency for the Gabriel-Isay condensation in the preparation of biopterin (1a) and neopterin (2a) derivatives from 2-ketoaldoses.
With regard to the sugar precursors for pteridine-ring formation, we chose three types of pentos-2-ulose, that is, 5-deoxy-pentofuranos-2-ulose, pentofuranos-2-ulose, and pentopyranos-2-ulose derivatives (6, 8, 10) (Scheme 2). 5-Deoxy-3-O-(p-methoxybenzyl)-L-erythro-pentofuranos-2-ulose (6) was prepared from L-rhamnose in nine steps via the thioacetal (5) according to the reported procedures.12
Glycosidation of D-ribose with benzyl alcohol, followed by protection with 1,1,3,3-tetraisopropyldisiloxan-1,3-diyl (TIPDS) group and Swern oxidation, afforded the benzyl pentofuranosid-2-ulose (7),13 which was then hydrogenated with 10%-Pd-C to give 3,5-O-TIPDS-D-erythro-pentofuranos-2-ulose (8). Meanwhile, benzyl glycosidation of D-arabinose, followed by acetalization with acetone and then Swern oxidation, provided the benzyl pentopyranosid-2-ulose (9),14 which was then hydrogenated to give 3,4-O-isopropylidene-D-erythro-pentopyranos-2-ulose (10).
The Gabriel-Isay condensation of pentos-2-uloses (6, 8, 10) with monosulfate of 2,5,6-triaminopyrimidin-4(3H)-one (3) was examined on both a condition to add sodium bicarbonate (pH 8) and a condition not to add (pH 3). The resulting mixture of the 6- and 7-substituted pterins was acetylated with acetic anhydride-pyridine for purification of products and determination of their ratio by 1H NMR spectra (Scheme 3, Table 1).
The pteridine ring formation of the 5-deoxy-pentofuranos-2-ulose (6) with 3 (1.2 mol equiv.) at pH 8 and the following acetylation afforded an inseparable mixture (76:24) of the 6-substituted pterin (biopterin) (11a) and its 7-substituted isomer (primapterin) (11b), whereas the same reaction at pH 3 resulted in a predominant formation of 11b in a ratio of 20:80 (Table 1, Entries 1,2).
The condensation of the 3,5-O-protected pentofuranos-2-ulose (8) with 3 showed a tendency of the regioselectivity similar to that of 6. Namely, the 6-substituted pterin (neopterin) (12a) was obtained as a major isomer under the basic condition, while the 7-substituted pterin (anapterin) (13b) was obtained selectively under the acidic condition accompanying with cleavage of the TIPDS group (Entries 3,4). The condensation of the 3,4-O-protected pentopyranos-2-ulose (10) with 3, however, resulted in a predominant formation of the 7-substituted isomers (14b and 13b) under both basic and acidic conditions (Entries 5,6).
From these results, the combination of the more reactive carbonyl group of the 2-uloses (6, 8, 10) and the more reactive amino group of the pyrimidine (3) under each condition seems to be as follows. Plausible pathways for the formation of the major isomers (12a, 13b, 14b) from the pentos-2-uloses (8 and 10) are illustrated in Scheme 4.
Under the basic condition (pH 8), the most nucleophilic 5-amino group of 3 would attack the 2-keto group of 6 and 8 (existing mainly as cyclic hemiacetals rather than open-chain 2-ketoaldehydes like 8’) and the following pteridine ring formation would preferentially yield the 6-substituted isomers 11a and 12a (Table 1, Entries 1,3). On the other hand, under the acidic condition (pH 3) an attack of the 6-amino group of 3 (owing to the protonation of the 5-amino group) to the 2-keto groups of 6 and 15 (arising from the TIPDS cleavage of 8) would predominantly take place to afford the 7-substituted isomers 11b and 13b (Entries 2,4).
In contrast to the results of Entries 1 and 3, the predominant production of the 7-substituted isomer (14b) from 10 in Entry 5 appears to ascribe to an initial attack of the 5-amino group of 3 to the C-1 of the aldehyde form present in an equilibrated solution of 10. The pentos-2-ulo-2,5-furanose (10’’)15 having a stable cis-fused bicyclic structure is considered as the most probable structure to afford 14b, rather than the acyclic aldehyde (10’). As well as Entry 4, the preferential formation of 13b in Entry 6 seems to result from the condensation of the 6-amino group of 3 with the C-2 of 15 derived from the acid hydrolysis of 10.
The present work thus demonstrates the regioselectivity for the Gabriel-Isay condensation of some pentos-2-uloses with 2,5,6-triaminopyrimidin-4(3H)-one (3). The condensation of 5-deoxy- and 5-O-protected pentofuranos-2-uloses with 3 under basic conditions was found to be effective for preparation of 6-substituted pterins such as biopterin (1a) and neopterin (2a), while the same reaction under acidic conditions was found to be convenient for 7-substituted pterins. The same condensation of the 3,4-O-protected pentopyranos-2-ulose, however, resulted in a predominant formation of 7-substituted pterins regardless of the pH values. Extension of this work including applications of these findings in synthesizing other 6- and 7-(polyhydroxyalkyl)pterins, as well as improvement of regioselectivity and yield for the condensation, is expected.
EXPERIMENTAL
All reactions were monitored by TLC (Merck Silica gel 60 F254 or Merck Cellulose F) with an appropriate solvent system. Column chromatography was performed with Daiso Silica Gel IR-60/210w. Components were detected by exposing the plates to UV light and/or spraying them with 20% H2SO4-EtOH (with subsequent heating). The 1H NMR spectra were measured in CDCl3 with Varian NMR System 600 (600 MHz) or 400-MR (400 MHz) at 23 °C. Chemical shifts are reported as δ values relative to CHCl3 (7.26 ppm) as an internal standard.
Di-N2:2’-O-acetyl-1’-O-(4-methoxybenzyl)biopterin (11a) and its 7-biopterin isomer (11b).
A. Condensation at pH 8. Monosulfate of 3 (90.0 mg, 0.376 mmol) and NaHCO3 (130 mg, 1.54 mmol) were dissolved in water (3 mL), and then a solution of compound 612 (79.0 mg, 0.313 mmol) dissolved in MeOH (3 mL) was added. The mixture was refluxed for 3 h and then evaporated in vacuo. The residue was dissolved in DMF and sodium sulfate was filtered off. The filtrate was concentrated in vacuo. The residue was dissolved in dry pyridine (3 mL) and acetic anhydride (0.50 mL, 5.3 mmol) was added at 0 °C. The mixture was stirred at rt for 16 h and then concentrated in vacuo. The residue was purified by column chromatography with 1:19 MeOH-CHCl3 to give an inseparable mixture (76:24) of the biopterin derivative (11a) and its 7-substituted isomer (11b) (116 mg, 84% yield from 6) as a pale yellow foam: Rf = 0.33 (1:19 MeOH-CHCl3): 1H-NMR (600 MHz) for 11a δ 1.24 (3H, d, J2’,3’ = 6.5 Hz, H3-3’), 1.96 (3H, s, AcO-2’), 2.42 (3H, s, AcN), 3.79 (3H, s, MeO), 4.51 (2H, s, CH2O-1’), 4.76 (1H, d, J1’,2’ = 5.0 Hz, H-1’), 5.37 (1H, qd, H-2’), 6.83, 7.20 (2H each, 2d, Jo,m = 8.5 Hz, C6H4), 9.09 (1H, s, H-7), 10.93 [1H, br s, H-N(3)], 12.60 (1H, br s, AcNH); 1H-NMR (600 MHz) for 11b δ 1.22 (3H, d, J2’, 3’ = 6.7 Hz, H3-3’), 1.98 (3H, s, AcO-2’), 2.42 (3H, s, AcN), 3.795 (3H, s, MeO), 4.52, 4.56 (1H each, 2d, 2J = 11.7 Hz, CH2O-1’), 4.68 (1H, d, J1’,2’ = 4.1 Hz, H-1’), 5.37 (1H, qd, H-2’), 6.85, 7.22 (2H each, 2d, Jo,m = 8.5 Hz, C6H4), 8.89 (1H, s, H-6), 10.37 [1H, br s, H-N(3)], 12.60 (1H, br s, AcNH). Anal. Calcd for C21H23N5O6: C, 57.14; H, 5.25. Found: C, 57.29; H, 5.14.
B. Condensation at pH 3. Monosulfate of 3 (86.0 mg, 0.360 mmol) was dissolved in water (3 mL), and then a solution of compound 6 (76.0 mg, 0.301 mmol) dissolved in MeOH (3 mL) was added. The mixture was refluxed for 3 h and then evaporated in vacuo. The residue was acetylated in the same manner described above to give an inseparable mixture (20:80) of the biopterin derivative (11a) and its 7-substituted isomer (11b) (101 mg, 76% yield from 6).
Di-N2:2’-O-acetyl-1’,3’-O-(1,1,3,3-tetraisopropyldisilosan-1,3-diyl)neopterin (12a) and its 7-neopterin isomer (12b).
Compound 713 (75.0 mg, 0.156 mmol) was dissolved in EtOAc (2 mL) and hydrogenated in the presence of 10% Pd-C (34.0 mg, 0.032 mmol) at rt under an atmospheric pressure of H2. After 20 h, the catalyst was filtered off and the filtrate was evaporated in vacuo to give 3,5-O-TIPDS-D-erythro- pentofuranos-2-ulose (8) (61.0 mg, quant.) in a colorless syrup: Rf = 0.50–0.38 (1:2 AcOEt-hexane).
Compound 8 was dissolved in MeOH (3 mL) and then added to a solution of 3 (monosulfate, 45.0 mg, 0.188 mmol) and NaHCO3 (53.0 mg, 0.629 mmol) dissolved in water (3 mL). The mixture was refluxed for 3 h and then evaporated in vacuo. The residue was dissolved in dry pyridine (2 mL) and acetic anhydride (0.40 mL, 4.2 mmol) was added at 0 °C. The mixture was stirred at rt for 16 h and then concentrated in vacuo. The residue was purified by column chromatography with 1:19 MeOH-CHCl3 to give an inseparable mixture (70:30) of the neopterin derivative (12a) and its 7-substituted isomer (12b) (57.1 mg, 63% yield from 8) as a pale yellow syrup: Rf = 0.68 (1:9 MeOH-CHCl3): 1H-NMR (600 MHz) for 12a δ 0.94–1.12 [28H, m, (Me2CH)2Si], 2.14 (3H, s, AcO-2’), 2.35 (3H, s, AcN), 3.70 (1H, dd, J3’a,3’b = 12.3, J2’,3’b = 8.2 Hz, Hb-3’), 4.10 (1H, dd, J2’,3’a = 1.5 Hz, Ha-3’), 4.52 (1H, ddd, H-2’), 5.77 (1H, d, J1’,2’ = 6.5 Hz, H-1’), 8.90 (1H, s, H-7), 10.95 [1H, br s, H-N(3)], 12.45 (1H, br s, AcNH); 1H-NMR (600 MHz) for 12b δ 0.94–1.12 [28H, m, (Me2CH)2Si], 1.98 (3H, s, AcO-2’), 2.35 (3H, s, AcN), 3.74 (1H, dd, J3’a,3’b = 12.3, J2’,3’b = 7.9 Hz, Hb-3’), 4.16 (1H, dd, J2’,3’a = 1.5 Hz, Ha-3’), 4.42 (1H, ddd, H-2’), 5.69 (1H, d, J1’,2’ = 6.5 Hz, H-1’), 8.75 (1H, s, H-6), 10.85 [1H, br s, H-N(3)], 12.45 (1H, br s, AcNH). Anal. Calcd for C25H41N5O7Si2: C, 51.79; H, 7.13. Found: C, 51.62; H, 7.21.
Tetra-N2:1’,2’,3’-O-acetylneopterin (13a) and its 7-neopterin isomer (13b).
A. From 8. By employing the same procedures as those for preparation for 11a,b (by condensation at pH 3), compound 8 gave an inseparable mixture (7:93) of the neopterin derivative (13a) and its 7-substituted isomer (13b) (59% yield) as a pale yellow syrup: Rf = 0.53 (1:9 MeOH-CHCl3); 1H-NMR (600 MHz) for 13a δ 1.98, 2.05, 2.16 (3H each, 3s, AcO-1’,2’,3’), 2.38 (3H, s, AcN), 4.30 (1H, dd, J3’a,3’b = 12.3, J2’,3’b = 5.6 Hz, Hb-3’), 4.42 (1H, dd, J2’,3’a = 3.2 Hz, Ha-3’), 5.63 (1H, ddd, H-2’), 6.14 (1H, d, J1’,2’ = 6.5 Hz, H-1’), 8.91 (1H, s, H-7), 10.90 [1H, br s, H-N(3)], 12.50 (1H, br s, AcNH); 1H-NMR (600 MHz) for 13b δ 2.00, 2.01, 2.20 (3H each, 3s, AcO-1’,2’,3’), 2.37 (3H, s, AcN), 4.26 (1H, dd, J3’a,3’b = 12.3, J2’,3’b = 6.3 Hz, Hb-3’), 4.38 (1H, dd, J2’,3’a = 3.7 Hz, Ha-3’), 5.63 (1H, ddd, H-2’), 6.11 (1H, d, J1’,2’ = 5.3 Hz, H-1’), 8.72 (1H, s, H-6), 10.90 [1H, br s, H-N(3)], 12.50 (1H, br s, AcNH). Anal. Calcd for C17H19N5O8: C, 48.46; H, 4.54. Found: C, 48.33; H, 4.61.
B. From 10. By the same procedures described above, compound 10 gave an inseparable mixture (17:83) of 13a and 13b in 53% yield.
Di-N2:3’-O-acetyl-1’,2’-O-isopropylideneneopterin (14a) and its 7-neopterin isomer (14b).
Compound 913 (124 mg, 0.446 mol) was dissolved in EtOH (2.5 mL) and hydrogenated in the presence of 20% Pd(OH)2-C (63.0 mg, 0.089 mmol) at rt under an atmospheric pressure of H2. After 20 h, the catalyst was filtered off and the filtrate was evaporated in vacuo to give 3,4-O-isopropylidene-D-erythro-pentopyranos-2-ulose (10) (84.0 mg, quant.) in a colorless syrup: Rf = 0.62–0.50 (AcOEt).
Compound 10 was treated with the same procedures as those described for 11a,b (by condensation at pH 8) to give an inseparable mixture (19:81) of the neopterin derivative (14a) and its 7-substituted isomer (14b) (110 mg, 65% yield from 10) as a pale yellow syrup: Rf = 0.58 (1:9 MeOH-CHCl3); 1H-NMR (400 MHz) for 14a δ 1.52, 1.71 (3H each, 2s, CMe2), 1.89 (3H, s, AcO-3’), 2.39 (3H, s, AcN), 3.67 (1H, dd, J3’a,3’b = 11.9, J2’,3’b = 5.9 Hz, Hb-3’), 3.98 (1H, dd, J2’,3’a = 3.9 Hz, Ha-3’), 4.83 (1H, ddd, H-2’), 5.62 (1H, d, J1’,2’ = 7.4 Hz, H-1’), 9.11 (1H, s, H-7), 10.90 [1H, br s, H-N(3)], 12.50 (1H, br s, AcNH); 1H-NMR (400 MHz) for 14b δ 1.52, 1.70 (3H each, 2s, CMe2), 1.86 (3H, s, AcO-3’), 2.36 (3H, s, AcN), 3.69 (1H, dd, J3’a,3’b = 12.1, J2’,3’b = 5.7 Hz, Hb-3’), 4.10 (1H, dd, J2’,3’a = 3.3 Hz, Ha-3’), 4.82 (1H, ddd, H-2’), 5.46 (1H, d, J1’,2’ = 7.6 Hz, H-1’), 8.92 (1H, s, H-6), 10.90 [1H, br s, H-N(3)], 12.50 (1H, br s, AcNH). Anal. Calcd for C16H19N5O6: C, 50.93; H, 5.08. Found: C, 50.84; H, 5.10.
ACKNOWLEDGEMENTS
We are grateful to the SC-NMR Laboratory of Okayama University for the NMR measurements.
References
1. (a) W. Pfleiderer, Angew. Chem., Int. Ed. Engl., 1964, 3, 114; CrossRef (b) H. Rembold and W. L. Gyure, Angew. Chem., Int. Ed. Engl., 1972, 11, 1061; CrossRef (c) T. Hama and T. Obika, Nature, 1960, 187, 326; CrossRef (d) H. S. Forrest and C. Van Baalen, Ann. Rev. Microbiol., 1970, 24, 91; CrossRef (e) I. Ziegler and R. Harmsen, Advan. Insect. Physiol., 1969, 6, 139.
2. E. L. Patterson, H. P. Broquist, A. M. Albrecht, M. H. von Saltza, and E. L. R. Stokstad, J. Am. Chem. Soc., 1955, 77, 3167. CrossRef
3. (a) S. Kaufman and E. E. Kaufman, ‘Folates and Pterins,’ ed. by R. Blakley and S. J. Benkovic, J. Wiley & Sons, New York, 1985, Vol. 2, pp. 251–352; (b) S. Kaufman and D. B. Fisher, ‘Molecular Mechanisms of Oxygen Activation,’ ed. by O. Hayaishi, Academic Press, New York, 1974, pp. 285–369; (c) P. F. Fitzpatrick, Annu. Rev. Biochem., 1999, 68, 355. CrossRef
4. (a) N. S. Kwon, C. F. Nathan, and D. J. Stuehr, J. Biol. Chem., 1989, 264, 20496; (b) M. A. Marletta, Cell, 1994, 78, 927; CrossRef (c) B. R. Crane, A. S. Arvai, D. K. Ghosh, C. Q. Wu, E. D. Getzoff, D. J. Stuehr, and J. A. Tainer, Science, 1998, 279, 2121. CrossRef
5. The trivial name recommended by the International Society of Pteridinology: J. Ferre, K. B. Jocobson, and W. Pfleiderer, Pteridines, 1990, 2, 129. CrossRef
6. (a) H.-C. Curtius, T. Kuster, A. Matasovic, N. Blau, and J.-L. Dhondt, Biochem. Biophys. Res. Commun., 1988, 153, 715; CrossRef (b) H.-C. Curtius, T. Kuster, A. Matasovic, N. Blau, and J.-L. Dhondt, J. Biol. Chem., 1990, 265, 3923.
7. (a) H. Wachter, E. R. Werner, G. Reibnegger, D. Fuchs, and A. Hausen, Pteridines, 1989, 1, 3; (b) A. Hausen, G. Reibnegger, B. Speck, and H. Wachter, Transplantation, 1984, 38, 497; CrossRef (c) G. Hoffman, S. Kenn, B. Wirleitner, C. Deetjen, S. Frede, M. Smolny, J. Rieder, D. Fuchs, G. Baier-Bitterlich, and W. Schobersberger, Immunobiology, 1998, 199, 63. CrossRef
8. (a) S. Gabriel and J. Colman, Ber. Dtsch. Chem. Ges., 1901, 34, 1234; CrossRef (b) O. Isay, Ber. Dtsch. Chem. Ges., 1906, 39, 250; CrossRef (c) A. Albert, Quart. Rev., Chem. Soc., 1952, 6, 197. CrossRef
9. (a) H. S. Forrest and J. Walker, J. Chem. Soc., 1949, 79; CrossRef (b) W. Pfleiderer, H. Zondler, and R. Mengel, Liebigs Ann. Chem., 1970, 741, 64; CrossRef (c) P. Waring and W. L. F. Armarego, Aust. J. Chem., 1985, 38, 629; CrossRef (d) S. Goswami and A. K. Adak, Tetrahedron Lett., 2002, 43, 8371. CrossRef
10. C. B. Storm, R. Shiman, and S. Kaufman, J. Org. Chem., 1971, 36, 3925. CrossRef
11. (a) J. Weinstock, U.S. Patent, 3,505,329, 1970 (Chem. Abstr., 1970, 72, 132787h); (b) T. Hanaya and H. Yamamoto, Heterocycles, 2012, 85, 2375. CrossRef
12. T. Hanaya, H. Baba, H. Toyota, and H. Yamamoto, Tetrahedron, 2009, 65, 7989. CrossRef
13. H. Kakinuma, H. Yuasa, and H. Hashimoto, Carbohydr. Res., 1998, 312, 103. CrossRef
14. (a) J. C. Wu, H. Bazin, and J. Chattopadhyaya, Tetrahedron, 1987, 43, 2355; CrossRef (b) T. Hanaya, D. Takayama, and H. Yamamoto, Heterocycles, 2006, 70, 355. CrossRef
15. T. Vuorinen and A. S. Serianni, Carbohydr. Res., 1990, 207, 185. CrossRef