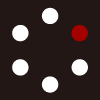
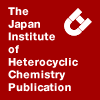
HETEROCYCLES
An International Journal for Reviews and Communications in Heterocyclic ChemistryWeb Edition ISSN: 1881-0942
Published online by The Japan Institute of Heterocyclic Chemistry
e-Journal
Full Text HTML
Received, 31st July, 2013, Accepted, 2nd September, 2013, Published online, 11th September, 2013.
DOI: 10.3987/COM-13-S(S)105
■ Enantioselective Synthesis of Planar-Chiral Phosphines with 1,N-Dioxa[N]paracyclophane Scaffold and Their Application as Chiral Ligands
Kazumasa Kanda, Shoya Oshima, Tsubasa Shizuno, Risa Hamanaka, Miku Fukai, and Takanori Shibata*
Chemistry and Biochemistry, Advanced Research Center of Science and Engineering, Waseda University, 3-4-1 Okubo, Shinjuku-ku, Tokyo 169-8555, Japan
Abstract
A new family of chiral phosphines based on planar-chiral 1,n-dioxa[n]paracyclophane scaffold was created. They were synthesized with excellent enantioselectivity via asymmetric ortho-lithiation using sec-butyllithium and (-)-sparteine. These phosphines were used as chiral ligands in three reactions: Ag-catalyzed allylation of imines, Pd-catalyzed asymmetric Sonogashira coupling of diiodoparacyclophanes, and Pd-catalyzed asymmetric Suzuki-Miyaura coupling of tricarbonyl(η6-ortho-dichlorobenzene)chromium.INTRODUCTION
Asymmetric catalysis is a powerful tool for the preparation of enantiomerically enriched compounds.1 Especially, chiral phosphines have shown great ability as efficient ligands, which induce high enantioselectivity in a number of transition-metal-catalyzed reactions such as asymmetric hydrogenation, conjugate addition, cross coupling and cycloaddition.1,2 Many of these chiral ligands contain some common backbones, such as binaphthyl, ferrocenyl, and spirocyclic systems. If a new chiral backbone is created, new and/or high selectivity, which cannot be achieved by conventional chiral ligands, would be expected. In this regard, planar-chiral paracyclophane structure is an attractive chiral framework.3,4 Actually, several [2.2]paracyclophane-based chiral ligands have been reported.5,6 Especially, 4,12-bis(diarylphosphino)[2.2]paracyclophanes (PHANEPHOS derivatives) are commercially available and realized higher enantioselectivity than other common chiral diphosphine ligands, such as BINAP derivatives in some reactions.7 But the enantioselective preparation of chiral [2.2]paracyclophane derivatives is still difficult. In many cases, individual and ingenious resolution procedures are necessary for racemic compounds.5,6,8 A few protocols of other planar-chiral paracyclophane synthesis was reported,4,9,10 however, their application for the synthesis of phosphine ligands is difficult. To the best of our knowledge, there is no phosphine ligand with planar-chiral paracyclophane scaffold other than [2.2]paracyclophane derivatives. Recently, we reported a new strategy for the planer-chiral paracyclophane synthesis: enantioselective ortho-lithiation of unflippable 1,n-dioxa[n]paracyclophanes (n ≤ 11) using sec-butyllithium and (-)-sparteine at -78 °C and further lithiation at -20 °C gave planar-chiral monolithiated and C2-symmetric dilithiated paracyclophanes, respectively. Subsequent treatment of these chiral lithium salts with various electrophiles gave planar-chiral mono- and disubstituted paracyclophanes with excellent to almost perfect ee (Scheme 1).11
We considered that this protocol can be used for the synthesis of planar-chiral phosphine derivatives. In this paper, a new family of planar-chiral phosphines with a 1,n-dioxa[n]paracyclophane backbone was synthesized by this method and used as chiral ligands in Ag-catalyzed asymmetric allylation, Pd-catalyzed Sonogashira, and Suzuki-Miyaura couplings.
RESULTS AND DISCUSSION
Each of 1,n-Dioxa[n]paracyclophanes 1a (n=10) and 1b (n=11) possesses an unflippable ansa chain,12,13 therefore, their mono-substitution on the benzene ring generates planar chirality (Table 1). The reaction of 1a and 1b with sec-butyllithium and (-)-sparteine at -78 °C in diethyl ether followed by treatment with chlorodiphenylphosphine gave planar-chiral diphenylphosphinoparacyclophanes 2a and 2b with excellent ee (Entries 1 and 2).14 The structure of 2b was determined by X-ray analysis and its ORTEP diagram was shown in Figure 1. Treatment of monolithiated 1b with chlorobis(3,5-bis(trifluoromethyl)phenyl)- phosphine gave corresponding electron-deficient phosphine 2c (Entry 3). Furthermore, planar-chiral phosphine with (1.4)naphthalenophane skeleton was obtained with excellent ee from 1c (Entry 4).
We also synthesized C2 symmetric planar-chiral diphosphines (Table 2). After the first enantioselective lithiation of 1a and 1b, further addition of two equivalent amounts of sec-butyllithium at -20 °C induced the second lithiation. Treatment of the obtained dilithiated compounds with excess amounts of chlorodiphenylphosphine gave the corresponding diphosphines 3a and 3b with almost perfect ee (Entries 1 and 2). The preparation of electron-deficient diphosphine 3c was also possible (Entry 3).
Next we considered the use of the obtained mono- and diphosphines as chiral ligands in transition-metal-catalyzed reactions and paid attention to the similarity as P,O-ligands between our developed phosphines and 2-(diphenylphosphino)-2'-methoxy-1,1'-binaphthyl (MOP). We first chose Ag-catalyzed enantioselective allylation of imines with allylsilanes, where MOP was the preferable ligand (Table 3).15 We used N-benzylidene-2-methoxyaniline (4a), which was a good substrate in the literature,15 and we examined asymmetric Ag-catalyzed allylation using monophosphines 2a, 2b and diphosphines 3a, 3b, but both of the yield and ee were low in all cases (Entries 1-4).16 In contrast, the reaction of N-benzylideneaniline (4b) smoothly proceeded and chiral homoallyl amine 5b was obtained in good yield with moderate ee (Entries 5-8). These results suggest that our developed planar-chiral phosphines could be used as chiral ligands and show the different selectivity with conventional ligands.
Next we focused attention on the planar chirality of our ligands. One of the typical chiral phosphine ligands with planar chirality is a ferrocenyl ligand, and various types of chiral ligands are now commercially available. We also used a ferrocenyl phosphine ligand, (RP)-1-[(R)-α-(dimethylamino)-2- (diphenylphosphino)benzyl]-2-diphenylphosphinoferrocene (TANIAPHOS) as the best ligand in the Pd-catalyzed asymmetric double Sonogashira coupling of diiodoparacyclophanes possessing a flippable ansa chain.10c Based on this background, we chose the asymmetric Sonogashira coupling as the second model reaction. Under the optimal reaction conditions, the coupling of diiodoparacyclophane 6a with 4-ethynylanisole (7a) gave dialkynylated product 8aa with 57% ee in the presence of cesium carbonate as a base (Table 4, Entry 1). When organic base such as diisopropylamine was used, the reaction proceeded smoothly at room temperature, but ee was low (Entry 2). Under the same reaction conditions, Sonogashira coupling was examined using paracyclophane-based ligands (Entries 3-6). As a result, monophosphine 2b realized the highest ee of 72% (Entry 4), which was higher than the previous best result (57% ee) (Entry 1). Even when the amount of the catalyst was lowered from 30 mol% to 10 mol%, comparable yield and ee were achieved (Entry 7).
Substrate scope of alkynes and diiodoparacyclophanes was examined, and the enantiomeric excesses under the previous optimal conditions were listed in the rightmost row for comparison (Table 5). A few MeO-substituted arylalkynes 7b-7d were submitted to the reaction with paracyclophane 6a and the corresponding dialkynylated products 8ab-8ad were obtained in excellent yield (Entries 1-3). Among them, 2-ethynylanisole (7c) induced the highest enantioselectivity of 76% (Entry 2). Phenylacetylene (7e) was also a good coupling partner (Entry 4). Propargyl ethers 7f and 7g could be used, but the yield was low to moderate because of the formation of 1,3-diynes as by-products (Entries 5 and 6). In the reaction of other diiodoparacyclophanes 6b-6e with alkyne 7a (Entries 7-10), 1,4-naphthylene-tethered paracyclophane 6c was a preferable substrate and the highest ee of 90% was achieved, which is the highest value among all previous results using TANIAPHOS as a chiral ligand.10c
Finally, we investigated enantioselective desymmetrization of tricarbonyl(η6-ortho-dichlorobenzene)- chromium 9 by Suzuki-Miyaura coupling because planar chiral ligand, N,N-dimethyl-1-[2- (diphenylphosphino)ferrocenyl]ethylamine (PPFA) realized good enantioselectivity in this reaction, but toxic TlOH was used as a base.17 We examined the coupling of the chromium arene complex with phenylboronic acid (10a) using much less toxic Na2CO3 as a base. According to the screening of planar-chiral phosphines (Table 6, Entries 1-4), diphosphine 3b was optimal and high enantioselectivity was achieved.18 The coupling of 9 with 4-methoxyphenylboronic acid (10b) also proceeded in high enantioselectivity (Entry 5).
In conclusion, a new family of [n]paracyclophane-based planar-chiral phosphines was synthesized by enantioselective ortho-lithiation of 1,n-dioxa[n]paracyclophane using sec-butyllithium and (-)-sparteine. Treatment of the chiral monolithiated- and dilithiated 1,n-dioxa[n]paracyclophane with a chlorophosphine derivatives gave planar-chiral monophosphines and C2-symmetric diphosphines, respectively, with excellent ee. These phosphines were used as chiral ligands in Ag-catalyzed allylation of imines and Pd-catalyzed couplings. As a result, good to high enantioselectivities were achieved in the asymmetric Sonogashira coupling of diiodoparacyclophanes possessing a flippable ansa chain (up to 90% ee) and asymmetric desymmetrization of tricarbonyl(η6-ortho-dichlorobenzene)chromium by Suzuki-Miyaura coupling (up to 85% ee).
EXPERIMENTAL
General. All reactions were examined under an argon atmosphere in oven-dried glassware with a magnetic stirring bar. A hexane-cyclohexane solution of sec-butyllithium (1.0 M) was purchased from Kanto Chemical Co., Inc. Dehydrated diethyl ether and tetrahydrofuran (THF) were purchased from Wako Pure Chemical Industries Ltd. (Wako), and purified by passing thorough a purification system (Glass Contour) before use.19 (-)-Sparteine was purchased from Tokyo Chemical Industry Co., Ltd. (TCI). Other reagents were purchased from Wako, Kanto, TCI, or Aldrich and were used without further purification. Flash column chromatography was performed with silica gel (Kanto Chemical Co., Inc. 60 N 40-50 μm). Preparative thin-layer chromatography (PTLC) was performed with silica gel-precoated glass plates (Merck 60 GF254) prepared in our laboratory. Gel permeation chromatography (GPC) was performed on JAI LC-908. IR spectra were recorded with Horiba FT730 spectrophotometer. NMR spectra were measured with JEOL AL-400 (400 MHz), JEOL ECS400 (400 MHz), JEOL ECX500 (500 MHz), or JEOL Lambda 500 (500 MHz) using TMS as an internal standard and CDCl3 was used as a solvent. High-resolution mass spectra (HRMS) were measured on a JEOL JMS-SX102A with FAB (Fast Atomic Bombardment) method or JMS-T100CS with ESI (Electro Spray Ionization) method. Optical rotations were measured with Jasco DIP-1000 polarimeter. Physical properties of phosphines 2a and 3b, and Sonogashira-coupling products 8aa-8ag were omitted, because they were already listed in the precedent report.10c,11
Typical Experimental Procedure of Enantioselective Monolithiation for the Synthesis of Monophosphine 2 (Table 1). A cyclohexane-hexane solution of sec-butyllithium (1.0 M, 1.2 or 2.0 mL, 1.2 or 2.0 mmol) was added dropwise to an ether solution (5 mL) of a 1,n-dioxa[n]paracyclophane 1 (1.0 mmol) and (-)-sparteine (230 μL, 1.0 mmol) at -78 °C and the reaction mixture was stirred for 3 h at -78 °C. To the mixture was added dropwise chlorodiarylphosphine (2 mmol) at -78 °C, and the reaction mixture stirred for 2 h at room temperature. It was filtered through a short plug of silica gel with CH2Cl2 and the filtrate was evaporated under reduced pressure. The crude products were purified by flash column chromatography (hexane/CH2Cl2= 3/1) to give the corresponding paracyclophane 2.
13-(Diphenylphosphino)-1,11-dioxa[11]paracyclophane (2b). White solid. Mp 110 °C; IR (CH2Cl2) 2925, 2854, 1473, 1456, 1194, 742, 696 cm-1; 1H NMR (500 MHz) δ 0.67-0.79 (m, 1H), 0.79-1.08 (m, 8H), 1.10-1.23 (m, 1H), 1.35-1.47 (m, 1H), 1.50-1.67 (m, 2H), 1.81-1.93 (m, 1H), 3.87-3.96 (m, 1H), 3.99-4.07 (m, 1H), 4.20-4.334 (m, 2H), 6.32-6.37 (m, 1H), 7.00-7.07 (m, 2H), 7.26-7.40 (m, 10H); 13C NMR (125 MHz) δ 25.8, 26.0, 26.0, 26.2, 26.5, 27.9, 29.8, 71.7, 72.0 (d, J= 2.1 Hz), 119.8 (d, J= 2.1 Hz), 122.1, 125.2, 128.3 (d, J= 6.2 Hz), 128.4, 128.5 (d, J= 6.2 Hz), 128.9, 130.9 (d, J= 13.4 Hz), 133.1 (d, J= 19.7 Hz), 134.5 (d, J= 20.7 Hz), 136.4 (d, J= 10.3 Hz), 137.1 (d, J= 11.3 Hz), 154.5, 156.6 (d, J= 16.6 Hz); 31P NMR (200 MHz) δ -17.9; HRMS (FAB+) for M+ found m/z 418.2057, calcd for C27H31O2P: 418.2056. [α]26D 5.8 (c 1.21 CHCl3, 98% ee). Ee was determined by HPLC analysis using a chiral column (Daicel Chiralpak IA: 4 x 250mm, 254nm UV detector, rt, eluent: 20% CH2Cl2 in hexane, flow rate: 0.5 mL/min, retention time: 9.1 min for major isomer and 9.8 min for minor isomer). The crystal data of 2b: C27H31O2P, M=418.51, monoclinic, space group P21 (no. 4), a = 8.7764(3) Å, b = 12.7465(4) Å, c = 10.3739(3) Å, β = 98.110(2) °, V = 1148.91(6) Å3, T=173 K, Z=2, µ(Cu Kα) 12.086 cm-1; number of reflections measured: total 12,015 and unique 4026, R1=0.0490, wR2=0.1295, Flack parameter (Friedel pairs=1823) 0.01(3). CCDC 953143.
13-(Bis(3,5-bis(trifluoromethyl)phenyl)phosphino)-1,11-dioxa[11]paracyclophane (2c). White solid. Mp 85 °C; IR (CH2Cl2) 2924, 1279, 1185, 1136, 899, 802, 682 cm-1; 1H NMR (400 MHz) δ 0.65-0.72 (m, 1H), 0.84-0.97 (m, 5H), 0.98-1.06 (m, 1H), 1.07-1.13 (m, 1H), 1.19-1.34 (m, 1H), 1.39-1.43 (m, 1H), 1.58-1.67 (m, 3H), 1.72-1.84 (m, 1H), 3.95-3.99 (m, 1H), 4.09-4.13 (m, 1H), 4.22-4.31 (m, 2H), 6.28-6.30 (m, 1H), 7.11-7.18 (m, 2H), 7.68-7.74 (m, 4H), 7.91 (d, J= 15.9 Hz, 2H); 13C NMR (125 MHz) δ 25.7, 25.8, 26.1, 26.6, 27.8, 29.5, 72.1 (d, J= 14.4 Hz), 119.9, 121.7 (d, J= 8.5 Hz), 123.4, 124.1-124.7 (m), 126.1 (d, J= 14.2 Hz), 131.9-132.5 (m), 133.1 (d, J= 26.3 Hz), 133.6 (d, J= 25.2 Hz), 138.5 (d, J= 22.4 Hz), 139.8 (d, J= 22.4 Hz), 154.9, 156.6 (d, J= 20.3 Hz); 31P NMR (200 MHz) δ -12.2; 19F NMR (470 MHz) δ -62.6, -62.9; HRMS (FAB+) for [M+H]+ found m/z 691.1627, calcd for C31H28O2F12P: 691.1630. [α]37D -28.5 (c 1.09 CHCl3, 98% ee). Ee was determined by HPLC analysis using a chiral column (Daicel Chiralpak IA: 4 x 250 mm, 254 nm UV detector, rt, eluent: 20% CH2Cl2 in hexane, flow rate: 0.5 mL/min, retention time: 6.0 min for major isomer and 8.3 min for minor isomer).
13-(Diphenylphosphinic)-1,11-dioxa[11](1,4)naphthalenophane (oxide of 2d). To a mixture of 1c and 2d in CH2Cl2-acetone (1/1, 0.5 mL), H2O2 (0.5 mL) was added and stirred for 1 h at room temperature. The solvent was removed under reduced pressure. The residue was extracted with CH2Cl2 and the combined organic layer was dried over Na2SO4. The solvent was removed under the reduced pressure and the crude products were purified by PTLC (AcOEt) to give the oxide of 2d. White solid. Mp 57 °C; IR (CH2Cl2) 3060, 1586, 1499, 1230, 1025, 728, 699 cm-1; 1H NMR (400 MHz) δ 0.26-0.29 (m, 3H), 0.60-0.63 (m, 3H), 0.77-0.82 (m, 3H), 0.88-0.90 (m, 1H), 1.19-1.24 (m, 1H), 1.34-1.39 (m, 1H), 1.70-1.76 (m, 1H), 1.80-1.86 (m, 1H), 4.17-4.28 (m, 2H), 4.31-4.40 (m, 2H), 6.98-7.02 (m, 1H), 7.45-7.47 (m, 4H), 7.51-7.53 (m, 3H), 7.58-7.60 (m, 1H), 7.71-7.78 (m, 4H), 8.12-8.17 (m, 1H), 8.24-8.28 (m, 1H); 13C NMR (125 MHz) δ 24.9, 25.9, 26.2, 26.3, 27.6, 29.4, 30.6, 72.4, 75.9, 115.6 (d, J= 11.7 Hz), 119.8, 120.9, 122.4, 124.3, 126.4, 128.0, 128.3 (d, J= 15.6 Hz), 128.5 (d, J= 14.4. Hz), 130.5 (d, J= 12.7 Hz), 131.1, 131.5, 131.6, 131.7, 132.0 (d, J= 12.0 Hz), 133.0, 152.0, 154.6; 31P NMR (200 MHz) δ 33.8; HRMS (FAB+) for [M+H]+ found m/z 485.2242, calcd for C31H34O3P: 485.2240. [α]34D -106.8 (c 1.58 CHCl3, 98% ee). Ee was determined by HPLC analysis using a chiral column (Daicel Chiralpak IC: 4 x 250 mm, 254 nm UV detector, rt, eluent: 50% 2-propanol in hexane, flow rate: 1.0 mL/min, retention time: 5.7 min for major isomer and 7.0 min for minor isomer).
Typical Experimental Procedure of Enantioselective Dilithiation for the Synthesis of Diphosphine 3 (Table 2). A cyclohexane-hexane solution of sec-butyllithium (1.0 M, 2.0 mL, 2.0 mmol) was added dropwise to an ether solution (5 mL) of a 1,n-dioxa[n]paracyclophane 1 (1 mmol) and (-)-sparteine (275 μL, 1.2 mmol) at -78 °C and the reaction mixture was stirred for 3 h at -78 °C. Then A cyclohexane-hexane solution of sec-butyllithium (1.0 M, 2.0 mL, 2.0 mmol) was added dropwise and the resulting mixture was stirred for 12 h at -20 °C. To the mixture was added dropwise chlorodiphenylphosphine (6 mmol) at -78 °C, and the reaction mixture was stirred for 2 h at room temperature. It was filtered through a short plug of silica gel with CH2Cl2 and the filtrate was evaporated under reduced pressure. The crude products were purified by flash column chromatography (hexane/CH2Cl2 = 5/1) to give the corresponding paracyclophane 3.
12,15-Bis(diphenylphosphino)-1,10-dioxa[10]paracyclophane (3a). White solid. Mp 218 °C; IR (CH2Cl2) 2926, 2854, 1465, 1433, 1384, 1348, 1331, 1265, 1244, 743, 696, 416 cm-1; 1H NMR (400 MHz) δ 0.49-0.55 (m, 2H), 0.80-0.86 (m, 2H), 1.03-1.04 (m, 4H), 1.50-1.51 (m, 2H), 1.52-1.53 (m, 2H), 3.73-3.77 (m, 2H), 4.08-4.13 (m, 2H), 6.28-6.30 (m, 2H), 7.25-7.36 (m, 20H); 13C NMR (125 MHz) δ 24.0, 26.6, 27.5 (d, J= 3.9 Hz), 72.3, 128.0 (d, J=7.2 Hz), 128.5 (d, J= 7.5 Hz), 129.1, 133.13, 134.6 (d, J= 19.9 Hz), 136.9, 155.1; 31P NMR (200 MHz) δ -12.2; HRMS (FAB+) for [M+H]+ found m/z 589.2446, calcd for C38H39O2P2: 589.2420. [α]37D -78.6 (c 0.1 CHCl3, 97% ee). Ee was determined by HPLC analysis of the oxidized product using a chiral column (Daicel Chiralpak IA: 4 x 250 mm, 254 nm UV detector, rt, eluent: 70% 2-propanol in hexane, flow rate: 1.0 mL/min, retention time: 7.5 min for major isomer and 12.9 min for minor isomer).
13,16-Bis(3,5-bis(trifluoromethyl)phenyl)phosphino)-1,11-dioxa[11]paracyclophane (3c). White solid. Mp 210 °C; IR (CH2Cl2) 2923, 1470, 1278, 1177, 1096, 898, 765 cm-1; 1H NMR (400 MHz) δ 0.71-0.75 (m, 2H), 0.91-1.12 (m, 6H), 1.38-1.43 (m, 2H), 1.53-1.62 (m, 2H), 1.67-1.72 (m, 2H), 3.81-3.87 (m, 2H), 4.11-4.17 (m, 2H), 6.31-6.33 (m, 2H), 7.64-7.65 (m, 4H), 7.72-7.74 (m, 4H), 7.93 (d, J= 5.5 Hz, 4H); 13C NMR (125 MHz) δ 25.5 (d, J= 4.8 Hz), 26.5, 27.9, 28.9, 72.2, 121.6 (d, J= 2.4 Hz), 122.8, 123.6-123.9 (m), 124.3, 127.0, 130.0 (d, J= 16.8 Hz), 133.0-133.5 (m), 137.2 (d, J= 21.5 Hz), 139.0 (d, J= 23.5 Hz), 156.4 (d, J= 20.3 Hz); 31P NMR (200 MHz) δ -11.4; 19F NMR (470 MHz) δ -63.1, -62.9; HRMS (FAB+) for [M+H]+ found m/z 1147.1578, calcd for C47H33F24O2P2: 1147.1567. [α]36D -82.5 (c 0.465 CHCl3, 98% ee). Ee was determined by HPLC analysis of the oxidized product using a chiral column (Doubly-arrayed Daicel Chiralcel IC and IC-3: 4 x 250 mm, 254 nm UV detector, rt, eluent: 50% CH2Cl2 in hexane, flow rate: 1.0 mL/min, retention time: 6.1 min for major isomer and 6.8 min for minor isomer).
Typical Experimental Procedure for Enantioselective Allylation of Imines 4b Using Phosphine Ligands (Table 3, Entries 5-8). A MeOH solution (1.0 mL) of AgF (6.3 mg, 0.05 mmol) and planar-chiral phosphine (0.05 mmol) was stirred for 10 min and then MeOH was excluded under vacuum for 1 h. After cooling of the resulting white solid to -78 °C, MeOH (20 µL, 0.5 mmol) and THF (1.5 mL) were added and the mixture was stirred for 10 min. To the resulting clear solution, allyltrimethoxysilane (1.0 mmol) and imine 4b (91.0 mg, 0.50 mmol) were added successively at -78 °C and the mixture was stirred at -78 °C for 12 h. The reaction mixture was passed through a short silica gel column using Et2O as an eluent. The solvent was excluded under the reduced pressure, and the crude products were purified by thin-layer chromatography to give allylated product 5b.20
Typical Experimental Procedure for Enantioselective Sonogashira Coupling (Table 5). A THF solution (0.2 mL) of PdCl2(MeCN)2 (3.9 mg, 0.015 mmol) and 2b (10.2 mg, 0.015 mmol) was stirred for 10 min. To the solution were added diiodoparacyclophane 6 (0.05 mmol) in THF (0.8 mL), i-Pr2NH (0.2 mL), alkyne 7 (0.2 mmol) and CuI (2.9 mg, 0.015 mmol) in order. The resulting mixture was stirred at room temperature. After the reaction was complete, H2O was added to the reaction mixture, and organic materials were extracted with AcOEt. The organic layer was washed with brine and dried with Na2SO4. The solvent was removed under reduced pressure, and the crude products were purified by thin-layer chromatography to give dialkynylated product 8.
22,25-Bis{2-(4-methoxyphenyl)ethynyl}-1,20-dioxa[9.9]paracyclophane (8ba). Yellow oil; IR (CH2Cl2) 2929, 2854, 2227, 1516, 1248, 1031, 831; 1H NMR δ 1.12-1.55 (m, 18H), 1.63-1.77 (m, 4H), 1.84-2.01 (m, 2H), 2.36-2.50 (m, 4H), 3.83 (s, 6H), 3.92-4.02 (m, 2H), 4.06-4.19 (m, 2H), 6.88 (d, J= 9.0 Hz, 4H), 6.95 (s, 4H), 6.99 (s, 2H), 7.47 (d, J= 9.0 Hz, 4H); 13C NMR δ 24.7, 27.1, 27.8, 28.0, 28.3, 30.7, 35.0, 55.3, 66.4, 84.8, 94.7, 113.9, 114.1, 115.7, 116.9, 128.3, 133.0, 139.7, 153.3, 159.5; HRMS (FAB+) for M+ found m/z 668.3848, calcd for C46H52O4: 668. 3860. [α]12D -23.9 (c 1.62 CHCl3, 55% ee). Ee was determined by HPLC analysis using a chiral column (Daicel Chiralpak IA: 4 x 250 mm, 254 nm UV detector, rt, eluent: 30% CH2Cl2 in hexane, flow rate: 1.0 mL/min, retention time: 7.5 min for major isomer and 13.1 min for minor isomer).
24,27-Bis{2-(4-methoxyphenyl)ethynyl}-1,22-dioxa[7](1,4)naphthalene[7]paracyclophane (8ca). Yellow oil; 1H NMR δ 1.17-1.32 (m, 4H) 1.33-1.49 (m, 2H), 1.59-1.79 (m, 6H), 1.81-1.96 (m, 2H), 1.97-2.13 (m, 2H), 2.74-2.92 (m, 2H), 3.02-3.18 (m, 2H), 3.83 (s, 6H), 3.96-4.22 (m, 4H), 6.28 (s, 2H), 6.89 (d, J= 8.8 Hz, 4H), 6.98 (s, 2H), 7.40 (dd, J= 3.3 Hz, 6.6 Hz, 2H), 7.50 (d, J= 8.8 Hz, 4H), 7.98 (dd, J= 3.3 Hz, 6.6 Hz, 2H); 13C NMR δ 23.1, 25.6, 27.7, 28.5, 30.6, 55.4, 67.7, 84.9, 95.0, 114.1, 114.6, 115.8, 117.4, 124.7, 124.8, 126.4, 132.2, 133.1, 135.5, 153.0, 159.7; HRMS (FAB+) for [M+Na]+ found m/z 685.3284, calcd for C46H46O4Na: 685.3288. [α]28D 16.5 (c 1.38 CHCl3, 54% ee). Ee was determined by HPLC analysis using a chiral column (Daicel Chiralpak IA: 4 x 250 mm, 254 nm UV detector, rt, eluent: 30% CH2Cl2 in hexane, flow rate: 1.0 mL/min, retention time: 5.5 min for major isomer and 8.4 min for minor isomer).
20,23-Bis{2-(4-methoxyphenyl)ethynyl}-1,18-dioxa[18]paracyclophane (8da). Pale yellow solid. Mp 134 °C; IR (CH2Cl2) 2925, 2852, 2208, 1604, 1515, 1463, 1248, 1172, 1032, 831 cm-1; 1H NMR δ 0.99-1.14 (m, 8H) 1.14-1.28 (m, 8H), 1.28-1.47 (m, 6H), 1.60-1.77 (m, 4H), 1.80-1.96 (m, 2H), 3.83 (s, 6H), 4.05-4.16 (m, 2H), 4.16-4.27 (m, 2H), 6.87 (d, J= 8.7 Hz, 4H), 7.00 (s, 2H), 7.47 (d, J= 8.7 Hz, 4H); 13C NMR δ 24.1, 26.9, 27.7, 28.0, 28.2, 28.7, 29.4, 55.2, 68.6, 84.8, 94.6, 113.9, 114.3, 115.8, 117.6, 133.0, 153.0, 159.6; HRMS (FAB+) for M+ found m/z 592.3550. calcd for C40H48O4: 592.3547. [α]26D -34.0 (c 1.55 CHCl3, 54% ee). Ee was determined by HPLC analysis using a chiral column (Daicel Chiralpak IA: 4 x 250 mm, 254 nm UV detector, rt, eluent: 30% CH2Cl2 in hexane, flow rate: 1.0 mL/min, retention time: 7.8 min for major isomer and 11.9 min for minor isomer).
22,25-Bis{2-(4-methoxyphenyl)ethynyl}-1,4,7,14,17,20-hexaoxa-[7.7]paracyclophane (8ea). Yellow solid. Mp 95 °C; IR (CH2Cl2) 2927, 2862, 2210, 1604, 1515, 1463, 1248, 1209, 1062, 1029, 833 cm-1; 1H NMR δ 3.72-3.80 (m, 2H), 3.83 (s, 6H), 3.83-3.92 (m, 2H), 3.92-4.10 (m, 8H), 4.18-4.25 (m, 4H), 6.58 (s, 4H), 6.88 (d, J= 9.1 Hz, 4H), 6.89 (s, 2H), 7.43 (d, J= 9.1 Hz, 4H); 13C NMR δ 55.3, 69.1, 69.5, 70.0, 70.0, 85.0, 94.5, 113.8, 114.0, 115.6, 116.4, 117.4, 132.9, 152.2, 152.9, 159.6; HRMS (FAB+) for M+ found m/z 620.2394, calcd for C38H36O8: 620.2405. [α]13D 10.5 (c 1.41 CHCl3, 58% ee). Ee was determined by HPLC analysis using a chiral column (Daicel Chiralpak IA: 4 x 250 mm, 254 nm UV detector, rt, eluent: 50% CH2Cl2 in hexane, flow rate: 1.0 mL/min, retention time: 5.1 min for major isomer and 6.1 min for minor isomer).
Typical Procedure for Enantioselective Suzuki Coupling (Table 6). A MeOH solution (0.5 mL) of PdCl2(MeCN)2 (3.9 mg, 0.015 mmol) and phosphine ligand (0.015 mmol) was stirred for 30 min. To the solution were added chromium complex 9 (14.2 mg, 0.05 mmol) and arylboronic acid 10 (0.1 mmol) in MeOH (0.5 mL) and Na2CO3 (10.6 mg, 0.1 mmol) in water (0.1 mL) in order. The resulting mixture was stirred at room temperature. After the reaction was complete, it was filtered through a short plug of silica gel with Et2O as an eluent and the filtrate was evaporated under reduced pressure. The crude products were purified by thin-layer chromatography (hexane/EtOAc = 5/1) to give mono-arylated product 11.
(1S,2R)-Tricarbonyl(2-phenylchlorobenzene)chromium (11a). The physical properties of this compound were consistent with those in the literature.11b
Tricarbonyl(2-(4-methoxyphenyl)chlorobenzene)chromium (11b). Yellow oil; IR (CH2Cl2) 1970, 1893, 1338, 1249, 1179 cm-1; 1H NMR δ 3.85 (s, 3H), 5.13 (dd, J= 6.0, 6.0 Hz, 1H), 5.45-5.54 (m, 2H), 5.62 (d, J= 6.0 Hz, 1H), 6.94 (d, J= 8.2 Hz, 2H), 7.45 (d, J= 8.2 Hz, 2H); 13C NMR δ 55.3, 87.8, 90.6, 93.2, 96.1, 110.3, 113.4, 113.6, 126.9, 131.7, 160.1, 231.7; HRMS (FAB+) for M+ found m/z 353.9744, calcd for C16H11O4ClCr: 353.9745; [α]27D 19.6 (c 1.44 CHCl3, 79% ee). Ee was determined by HPLC analysis using a chiral column (Doubly-arrayed Daicel Chiralpak AD-H: 4 x 250 mm, 254 nm UV detector, rt, eluent: 2% 2-propanol in hexane, flow rate: 1.0 mL/min, retention time: 9 min for minor isomer and 10 min for major isomer).
ACKNOWLEDGEMENTS
K. Kanda is grateful to the Japan Society for the Promotion of Science for the fellowship support. This work was supported by Grant-in-Aid for Scientific Research from the Ministry of Education, Culture, Sports, Science and Technology, Japan (No. 23655091). We also thank Asahi Glass Foundation, the Global COE program ‘Center for Practice Chemical Wisdom’ by MEXT, and ACT-C. We also express our thanks to Dr. Takashi Otani (Waseda Univ.) for general support for this project.
References
1. For reviews, see: (a) Comprehensive Asymmetric Catalysis, ed. by E. N. Jacobsen, A. Pfaltz, and H. Yamamoto, Springer, Berlin, Germany, 1999, vol. 2; (b) New Frontiers in Asymmetric Catalysis, ed. by K. Mikami and M. Lautens, Wiley-Interscience, 2007; CrossRef (c) V. Caprio and J. M. J. Williams, Catalysis in Asymmetric Synthesis, Wiley-Blackwell, Hoboken, USA, 2009; (d) Catalytic Asymmetric Synthesis, ed. by I. Ojima, Wiley-VCH, New York, USA, 2010.
2. For reviews, see: Phosphorus Ligands in Asymmetric Catalysis, ed. by A. Börner, Wiley-VCH, New York, USA, 2008.
3. For reviews, see: (a) F. Vögtle, Cyclophane Chemistry, Wiley, Chichester, UK, 1993; (b) Modern Cyclophane Chemistry; ed. by R. Gleither and H. Hopf, Wiley-VCH, New York, USA, 2004.
4. Several application of planar-chiral paracyclophane, see: (a) S. Oi and S. Miyano, Chem. Lett., 1992, 987; CrossRef (b) T. Hattori, N. Harada, S. Oi, H. Abe, and S. Miyano, Tetrahedron: Asymmetry, 1995, 6, 1043; CrossRef (c) T. Hattori, N. Koike, Y. Okaishi, and S. Miyano, Tetrahedron Lett., 1996, 37, 2057; CrossRef (d) R. Fiesel, J. Huber, and U. Scherf, Angew. Chem., Int. Ed. Engl., 1996, 35, 2113; CrossRef (e) R. Fiesel, J. Huber, U. Apel, V. Enkelmann, R. Hentschke, and U. Scherf, Macromol. Chem. Phys., 1997, 198, 2623; CrossRef (f) R. Maeda, T. Wada, T. Mori, S. Kono, N. Kanomata, and Y. Inoue, J. Am. Chem. Soc., 2011, 133, 10379; CrossRef (g) Y. Morisaki, R. Hifumi, L. Lin, K. Inoshita, and Y. Chujo, Polym. Chem., 2012, 3, 2727. CrossRef
5. For a review of [2.2]paracyclophane derivatives for asymmetric syntheses, see: S. E. Gibson and J. D. Knight, Org. Biomol. Chem., 2003, 1, 1256; CrossRef (b) J. Paradies, Synthesis, 2011, 3749. CrossRef
6. For example of planar-chiral [2.2]paracyclophane-based monophosphine derivatives, see: (a) X.-W. Wu, K. Yuan, W. Sun, M.-J. Zhang, and X.-L. Hou, Tetrahedron: Asymmetry, 2003, 14, 107; CrossRef (b) T. Focken, G. Raabe, and C. Bolm, Tetrahedron: Asymmetry, 2004, 15, 1693; CrossRef (c) D. K. Whelligan and C. Bolm, J. Org. Chem., 2006, 71, 4609; CrossRef (d) T.-Z. Zhang, L.-X. Dai, and X.-L. Hou, Tetrahedron: Asymmetry, 2007, 18, 251; CrossRef (e) B. Jiang, Y. Lei, and X.-L. Zhao, J. Org. Chem., 2008, 73, 7833. CrossRef
7. For examples of PHANEPHOS derivatives as chiral ligands, see: (a) P. J. Pye, K. Rossen, R. A. Reamer, N. N. Tsou, R. P. Volante, and P. J. Reider, J. Am. Chem. Soc., 1997, 119, 6207; CrossRef (b) K. Rossen, P. J. Pye, A. Maliakal, and R. P. Volante, J. Org. Chem., 1997, 62, 6462; CrossRef (c) P. J. Pye, K. Rossen, R. A. Reamer, R. P. Volante, and P. J. Reider, Tetrahedron Lett., 1998, 39, 4441; CrossRef (d) P. W. Dyer, P. J. Dyson, S. L. James, C. M. Martin, and P. Suman, Organometallics, 1998, 17, 4344; CrossRef (e) M. J. Burk, W. Hems, D. Herzberg, C. Malan, and A. Zanotti-Gerosa, Org. Lett., 2000, 2, 4173; CrossRef (f) H. Heinrich, R. Giernoth, J. Bargon, and J. M. Brown, Chem. Commun., 2001, 1296; CrossRef (g) L. T. Boulton, I. C. Lennon, and R. McCague, Org. Biomol. Chem., 2003, 1, 1094; CrossRef (h) M. Rubina, M. Rubin, and V. Gevorgyan, J. Am. Chem. Soc., 2003, 125, 7198; CrossRef (i) B. Dominguez, A. Zanotti-Gerosa, and W. Hems, Org. Lett., 2004, 6, 1927; CrossRef (j) H.-Y. Jang, F. W. Hughes, H. Gong, J. Zhang, J. S. Brodbelt, and M. J. Krische, J. Am. Chem. Soc., 2005, 127, 6174; CrossRef (k) T. G. Driver, J. R. Harris, and K. A. Woerpel, J. Am. Chem. Soc., 2007, 129, 3836; CrossRef (l) M. N. Cheemala, M. Gayral, J. M. Brown, K. Rossen, and P. Knochel, Synthesis, 2007, 3877; CrossRef (m) A. C. Laungania and B. Breit, Chem. Commun., 2008, 844; CrossRef (n) G. A. Grasa, A. Zanotti-Gerosa, S. Ghosh, C. A. Teleha, W. A. Kinney, and B. E. Maryanoff, Tetrahedron, Lett., 2008, 49, 5328; CrossRef (o) C. A. Mullen, A. N. Campbell, and M. R. Gagné, Angew. Chem. Int. Ed., 2008, 47, 6011; CrossRef (p) T. M. Konrad, J. A. Fuentes, A. M. Z. Slawin, and M. L. Clarke, Angew. Chem. Int. Ed., 2010, 49, 9197; CrossRef (q) A. M. R. Smith, H. S. Rzepa, A. J. P. White, D. Billen, and K. K. Hii, J. Org. Chem., 2010, 75, 3085; CrossRef (r) B.-M. Fan, X.-J. Li, F.-Z. Peng, H.-B. Zhang, A. S. C. Chan, and Z.-H. Shao, Org. Lett., 2010, 12, 304; CrossRef (s) R. J. Felix, D. Weber, O. Gutierrez, D. J. Tantillo, and M. R. Gagné, Nature Chem., 2012, 4, 405; CrossRef (t) N. A. Cochrane, H. Nguyen, and M. R. Gagné, J. Am. Chem. Soc., 2013, 135, 628. CrossRef
8. Review of optical resolution of [2.2]paracyclophanes, see: (a) G. J. Rowlands, Org. Biomol. Chem., 2008, 6, 1527; CrossRef Recently, practical resolution of pseudo-ortho disubstituted [2.2]paracyclophanes, see: (b) Y. Morisaki, R. Hifumu, L. Lin, K. Inoshita, and Y. Chujo, Chem. Lett., 2012, 41, 990. CrossRef
9. (a) T. Ueda, N. Kanomata, and H. Machida, Org. Lett., 2005, 7, 2365; CrossRef (b) K. Mori, K. Ohmori, and K. Suzuki, Angew. Chem. Int. Ed., 2009, 48, 5638. CrossRef
10. Example of preparation of planar-chiral paracyclophanes by enantioselective reaction, see (a) G. Islas-Gonzalez, M. Bois-Choussy, and J. Zhu, Org. Biomol. Chem., 2003, 1, 30; CrossRef (b) K. Tanaka, T. Hori, T. Osaka, K. Noguchi, and M. Hirano, Org. Lett., 2007, 9, 4881; CrossRef (c) K. Kanda, T. Koike, K. Endo, and T. Shibata, Chem. Commun., 2009, 1870; (d) T. Hori, Y. Shibata, and K. Tanaka, Tetrahedron: Asymmetry, 2010, 21, 1303; CrossRef (e) T. Araki, D. Hojo, K. Noguchi, and K. Tanaka, Synlett, 2011, 539; CrossRef (f) T. Araki, K. Noguchi, and K. Tanaka, Angew. Chem. Int. Ed., 2013, 52, 5617. CrossRef
11. (a) K. Kanda, K. Endo, and T. Shibata, Org. Lett., 2010, 12, 1980; CrossRef (b) K. Kanda, R. Hamanaka, K. Endo, and T. Shibata, Tetrahedron, 2012, 68, 1407. CrossRef
12. For a review, see: K. Schlögl, Top. Curr. Chem., 1984, 125, 27. CrossRef
13. (a) A. Lüttringhaus and H. Gralheer, Liebigs Ann., 1942, 550, 67; CrossRef (b) D. H. Hochmuth and W. A. König, Liebigs Ann., 1996, 947; (c) D. H. Hochmuth and W. A. König, Tetrahedron: Asymmetry, 1999, 10, 1089; CrossRef (d) K. P. Scharwächter, D. H. Hochmuth, H. Dittmann, and W. A. König, Chirality, 2001, 13, 679. CrossRef
14. No racemization of 2a in solid state was observed at room temperature for about 8 months.
15. M. Naodovic, M. Wadamoto, and H. Yamamoto, Eur. J. Org. Chem., 2009, 5129. CrossRef
16. Ligand screening was examined by the use of phosphines obtained in Tables 1 and 2.
17. (a) M. Uemura, H. Nishimura, and T. Hayashi, Tetrahedron Lett., 1993, 34, 107; CrossRef (b) M. Uemura, H. Nishimura, and T. Hayashi, J. Organomet. Chem., 1994, 473, 129. CrossRef
18. When the reaction was quenched for 1 h under the reaction condition of Entry 3, the yield decreased (53%), but the ee was almost unchanged (85% ee). These results mean that the enhancement of ee by kinetic resolution of second arylation was not significant.
19. A. B. Pangborn, M. A. Giardello, R. H. Grubbs, R. K. Rosen, and F. J. Timmers, Organometallics, 1996, 15, 1518. CrossRef
20. H. Nakamura, K. Nakamura, and Y. Yamamoto, J. Am. Chem. Soc., 1998, 120, 4242. CrossRef