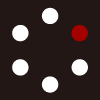
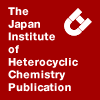
HETEROCYCLES
An International Journal for Reviews and Communications in Heterocyclic ChemistryWeb Edition ISSN: 1881-0942
Published online by The Japan Institute of Heterocyclic Chemistry
e-Journal
Full Text HTML
Received, 12th April, 2013, Accepted, 27th May, 2013, Published online, 30th May, 2013.
DOI: 10.3987/COM-13-S(S)4
■ Copper-Mediated Regioselective Homocoupling of Thiophenes and Indoles via Directed C–H Cleavage
Riko Odani, Mayuko Nishino, Koji Hirano,* Tetsuya Satoh, and Masahiro Miura*
Department of Applied Chemistry, Faculty of Engineering, Osaka University, Suita, Osaka 560-0871, Japan
Abstract
We have developed a copper-mediated regioselective homocoupling reaction of thiophenes and indoles via functional-group-directed C–H cleavage to afford the corresponding bisthiophenes (bithiophenes) and bisindoles directly. The copper-based system can provide a concise and precious-metal-free access to the above heterocyclic cores of fundamental importance in material and pharmaceutical chemistry.Bisthiophenes (bithiophenes) and bisindoles are frequently occurring heterocyclic structures in functional materials and pharmaceuticals. For example, the former is a key motif in many organic field effect transistors (OFETs), organic light-emitting diodes (OLEDs), and photovoltaic cells.1 The latter is also found in many biologically active compounds and alkaloids.2 Among numerous approaches to the above target structures, in addition to the conventional cross-coupling technologies with organic halides and organometallic reagents, metal-mediated direct C–H functionalization methodology has recently received significant attention.3 In particular, dehydrogenative homocoupling reactions of thiophenes4 and indoles5 via two-fold C–H cleavage is of great interest. To date, a combination of precious palladium catalysts and a stoichiometric amount of copper- or silver-based oxidants is generally employed for such transformations. On the other hand, our group6 and others7 have focused on the potential and unique activity of less expensive and abundant copper salts and complexes, and succeeded in some types of copper-promoted C–H functionalization of (hetero)arenes. In this context, copper-mediated or copper-catalyzed regioselective direct homocoupling reactions also have been developed. However, in most cases, the substrate scope is still limited to relatively acidic arenes such as 1,3-azoles and polyfluoroarenes.8 Thus, the application of copper-based protocols to nonacidic thiophenes and indoles remains somewhat challenging and is strongly desired.9 Here we report a copper-mediated regioselective homocoupling of thiophenes and indoles through functional-group-directed dual C–H cleavage, providing bisthiophenes and bisindoles directly.
Very recently, we reported a copper-mediated C–H/C–H biaryl cross-coupling of benzoic acid derivatives and 1,3-azoles6p with the aid of double coordination.10 In the course of this study, we attempted the reaction of 2-thiophenecarboxamide 1a with pentafluorobenzene. However, the desired cross-coupling product was not observed. Instead, a small but significant amount of homocoupling product, bisthiophene 2a, was detected (Scheme 1).
The serendipity prompted us to optimize conditions for the regioselective homocoupling of 1a. After investigation of various reaction parameters, we identified higher reaction temperature (165 ˚C) to be essential for the satisfactory yield of 2a (Table 1, entry 1). Under the same conditions, we next performed the reaction of an array of substituted thiophenecarboxamides 1. The installation of any substituents at the C5 position gave negative impacts on yield; methyl and chloro-substituted bisthiophenes 2b and 2c were obtained in lower yields (entries 2 and 3). On the other hand, 4-substituted 2-thiophenecarboxamide 1d was completely inert probably due to steric factors (entry 4). 3-Thiophenecarboxamide 1e showed good reactivity, and a regioisomeric mixture of 2,2’- and 2,4’-bisthiophenes was obtained in a ratio of 78:22 (entry 5). Some substituted 3-thiophenecarboxamides were also tested. Although moderate, the corresponding phenyl-substituted bisthiophenes 2f, 2g, and 2h were formed directly (entries 6–8). In the case of 5-phenyl-3-thiophenecarboxamide 1h, the C–C bond formation occurred regioselectively at the less congested C2 position (entry 8). Moreover, methoxyphenyl and thienyl groups were tolerated, and functionalized π-extended bisthiophene cores 2i and 2j were readily accessible (entries 9 and 10). The resultant amide moiety can be easily transformed to the ethyl ester, which is useful synthetic handle for further manipulations.6p Unfortunately, attempts to apply other arenecarboxamides involving benzene, furan, and pyrrole remained unsuccessful, and the homocoupling reaction was unique to the thiophene ring.
Although the detailed mechanism is not yet clear at the present, the reaction is believed to proceed through 1) N,N-double chelation-assisted C–H cupration of the first thiophene to provide a Cu(II)-containing metalacycle, 2) oxidation of Cu(II) into Cu(III) through disproportionation by additional Cu(OAc)2, 3) C–H metalation of the second thiophene at the Cu(III) center, and 4) productive reductive elimination of two thiophene ligands from the Cu(III) intermediate, as exemplified by the reaction of 1a shown in Scheme 2. To get some information about the proposed above mechanism, we carried out the reaction of 1e in the presence of AcOH-d4 at lower temperature (135 ˚C) (Scheme 3). While neither 2e nor 2e’ were detected, the recovered 1e showed significant amount of H/D exchange at both C2 and C4 positions. The result indicates that the C–H cleavage of, at least, the first thiophene, reversibly occurs even at non-productive lower temperature. Thus, the rate-limiting step may be involved in elementary steps after the first C–H cleavage. In view of the rapid reductive elimination from some Cu(III) complexes in the literature,11 the disproportionation or second C–H cleavage is believed to be rate-limiting. Further efforts on clarification of the detailed mechanism are ongoing.
Finally, we turned our attention to indoles. To our delight, N-(2-pyrimidyl)indole and N-(2-pyridyl)indole6n underwent the homocoupling under similar copper-based conditions to provide the corresponding 2,2’-bisindoles regioselectively (Scheme 4, 3a and 3b). The reaction was compatible with methoxy and chloro groups, and functionalized 2,2’-bisindoles were readily obtained (3c and 3d).
In summary, we have developed a precious-metal-free copper-mediated direct homocoupling of thiophenes and indoles via directed C–H cleavage.12 The copper-based reaction system can provide a facile access to bisthiophenes and bisindoles of fundamental importance in material science and pharmaceutical chemistry. Moreover, the reaction is also regarded as a limited successful example of the copper-mediated direct biaryl formation with nonacidic (hetero)arenes.
EXPERIMENTAL
Copper-mediated homocoupling of thiophenecarboxamides: the reaction of 1a is representative (Table 1, entry 1). Cu(OAc)2 (91 mg, 0.50 mmol) and N-(quinolin-8-yl)thiophene-2-carboxamide (1a, 64 mg, 0.25 mmol) were placed in a 20 mL two-necked reaction flask equipped with a reflux condenser. The flask was filled with nitrogen by using the standard Schrenk technique. o-Xylene (1.5 mL) was added dropwise via a syringe, and the solution was stirred at 165 °C for 3 h. The resulting mixture was then quenched with water. The mixture was extracted with ethyl acetate, and the combined organic layer was dried over sodium sulfate. Concentration in vacuo followed by silica gel column purification with hexane/ethyl acetate (1:1, v/v) gave N2, N2'-di(quinolin-8-yl)-[3,3'-bithiophene]-2,2'-dicarboxamide (2a, 43 mg, 0.085 mmol) in 67% yield.
ACKNOWLEDGEMENTS
This work was partly supported by Grants-in-Aid from MEXT and JSPS, Japan. M.M. acknowledges Kansai Research Foundation for technology promotion.
References
1. (a) H. E. Katz, Z. Bao, and S. L. Gilat, Acc. Chem. Res., 2001, 34, 359; CrossRef (b) K. Takimiya, Y. Kunugi, and T. Otsubo, Chem. Lett., 2007, 36, 578; CrossRef (c) A. R. Murphy and J. M. J. Fréchet, Chem. Rev., 2007, 107, 1066; CrossRef (d) B. S. Ong, Y. Wu, Y. Li, P. Liu, and H. Pan, Chem. Eur. J., 2008, 14, 4766; CrossRef (e) S. Allard, M. Forster, B. Souharce, H. Thiem, and U. Schert, Angew. Chem. Int. Ed., 2008, 120, 4138; CrossRef (f) J. Chen and A. Y. Cao, Acc. Chem. Res., 2009, 42, 1709. CrossRef
2. (a) G. W. Gribble and S. J. Berthel, 'Studies in Natural Products Chemistry,' Vol. 12, ed. by Attaur-Rahman, Elsevier, New York, 1993, pp. 365-409; (b) L. E. Overman, J. F. Larrow, B. A. Stearns, and J. M. Vance, Angew. Chem. Int. Ed., 2000, 39, 213; CrossRef (c) N. Wahlström, J. Slätt, B. Stensland, A. Ertan, J. Bergman, and T. Janosik, J. Org. Chem., 2007, 72, 5886; CrossRef (d) A. Pezzella, L. Panzella, A. Natangelo, M. Arzillo, A. Napolitano, and M. d’Ischisa, J. Org. Chem., 2007, 72, 9225; CrossRef (e) M. Movassaghi and M. A. Schmidt, Angew. Chem. Int. Ed., 2007, 46, 3725. CrossRef
3. Recent reviews: (a) D. Alberico, M. E. Scott, and M. Lautens, Chem. Rev., 2007, 107, 174; CrossRef (b) T. Satoh and M. Miura, Chem. Lett., 2007, 36, 200; CrossRef (c) F. Kakiuchi and T. Kochi, Synthesis, 2008, 3013; CrossRef (d) X. Chen, K. M. Engle, D.-H. Wang, and J.-Q. Yu, Angew. Chem. Int. Ed., 2009, 48, 5094; CrossRef (e) L. Ackermann, R. Vicente, and A. R. Kapdi, Angew. Chem. Int. Ed., 2009, 48, 9792; CrossRef (f) T. W. Lyons and M. S. Sanford, Chem. Rev., 2010, 110, 1147; CrossRef (g) J. Yamaguchi, A. D. Yamaguchi, and K. Itami, Angew. Chem. Int. Ed., 2012, 51, 8960. CrossRef
4. (a) K. Masui, H. Ikegami, and A. Mori, J. Am. Chem. Soc., 2004, 126, 5075; CrossRef (b) M. Takahashi, K. Masui, H. Sekiguchi, N. Kobayashi, A. Mori, M. Funahashi, and N. Tamokai, J. Am. Chem. Soc., 2006, 128, 10930. CrossRef
5. (a) Z. Liang, J. Zhao, and Y. Zhang, J. Org. Chem., 2010, 75, 170; CrossRef (b) Y. Li, W.-H. Wang, S.-D. Yang, B.-J. Li, C. Feng, and Z.-J. Shi, Chem. Commun., 2010, 46, 4553; CrossRef (c) A. García-Rubia, B. Urones, R. G. Arrayás, and J. C. Carretero, Chem. Eur. J., 2010, 16, 9676. CrossRef
6. (a) T. Yoshizumi, H. Tsurugi, T. Satoh, and M. Miura, Tetrahedron Lett., 2008, 49, 1598; CrossRef (b) T. Yoshizumi, T. Satoh, K. Hirano, D. Matsuo, A. Orita, J. Otera, and M. Miura, Tetrahedron Lett., 2009, 50, 3273; CrossRef (c) T. Kawano, T. Yoshizumi, K. Hirano, T. Satoh, and M. Miura, Org. Lett., 2009, 11, 3072; CrossRef (d) M. Kitahara, K. Hirano, H. Tsurugi, T. Satoh, and M. Miura, Chem. Eur. J., 2010, 16, 1772; CrossRef (e) T. Kawano, N. Matsuyama, K. Hirano, T. Satoh, and M. Miura, J. Org. Chem., 2010, 75, 1764; CrossRef (f) N. Matsuyama, M. Kitahara, K. Hirano, T. Satoh, and M. Miura, Org. Lett., 2010, 12, 2358; CrossRef (g) T. Kawano, K. Hirano, T. Satoh, and M. Miura, J. Am. Chem. Soc., 2010, 132, 6900; CrossRef (h) T. Yao, K. Hirano, T. Satoh, and M. Miura, Angew. Chem. Int. Ed., 2011, 50, 2990; CrossRef (i) M. Kitahara, N. Umeda, K. Hirano, T. Satoh, and M. Miura, J. Am. Chem. Soc., 2011, 133, 2160; CrossRef (j) N. Matsuda, K. Hirano, T. Satoh, and M. Miura, Org. Lett., 2010, 13, 2860; CrossRef (k) H. Hachiya, K. Hirano, T. Satoh, and M. Miura, Org. Lett., 2011, 13, 3076; CrossRef (l) Y. Oda, N. Matsuyama, K. Hirano, T. Satoh, and M. Miura, Synthesis, 2012, 44, 1515; CrossRef (m) A. Nakatani, K. Hirano, T. Satoh, and M. Miura, Org. Lett., 2012, 14, 2586; CrossRef (n) M. Nishino, K. Hirano, T. Satoh, and M. Miura, Angew. Chem. Int. Ed., 2012, 51, 6993; CrossRef (o) K. Hirano and M. Miura, Chem. Commun., 2012, 48, 10704; CrossRef (p) M. Nishino, K. Hirano, T. Satoh, and M. Miura, Angew. Chem. Int. Ed., 2013, 52, 4457. CrossRef
7. Reviews: (a) O. Daugulis, H.-Q. Do, and D. Shabashov, Acc. Chem. Res., 2009, 42, 1074; (b) A. A. Kulkarni and O. Daugulis, Synthesis, 2009, 4087; CrossRef Selected work: (c) X. Chen, X.-S. Hao, C. E. Goodhue, and J.-Q. Yu, J. Am. Chem. Soc., 2006, 128, 6790; CrossRef (d) H.-Q. Do and O. Daugulis, J. Am. Chem. Soc., 2007, 129, 12404; CrossRef (e) L. Ackermann, H. K. Potukuchi, D. Landsberg, and R. Vicente, Org. Lett., 2008, 10, 3081; CrossRef (f) I. Ban, T. Sudo, T. Taniguchi, and K. Itami, Org. Lett., 2008, 10, 3607; CrossRef (g) G. Brasche and S. L. Buchwald, Angew. Chem. Int. Ed., 2008, 47, 1932; CrossRef (h) S. Ueda and H. Nagasawa, Angew. Chem. Int. Ed., 2008, 47, 6411; CrossRef (i) R. J. Phipps, N. P. Grimster, and M. J. Gaunt, J. Am. Chem. Soc., 2008, 130, 8172; CrossRef (j) S. Yotphan, R. G. Bergman, and J. A. Ellman, Org. Lett., 2009, 11, 1511; CrossRef (k) R. J. Phipps and M. J. Gaunt, Science, 2009, 323, 1593; CrossRef (l) D. Zhao, W. Wang, F. Yang, J. Lan, L. Yang, G. Gao, and J. You, Angew. Chem. Int. Ed., 2009, 48, 3296; CrossRef (m) F. Besseliévre and S. Piguel, Angew. Chem. Int. Ed., 2009, 48, 9553; CrossRef (n) D. Monguchi, T. Fujiwara, H. Furukawa, and A. Mori, Org. Lett., 2009, 11, 1607; CrossRef (o) J. J. Mousseau, J. A. Bull, and A. B. Charette, Angew. Chem. Int. Ed., 2010, 49, 1115. CrossRef
8. (a) H.-Q. Do and O. Daugulis, J. Am. Chem. Soc., 2009, 131, 17052; CrossRef (b) D. Monguchi, A. Yamamura, T. Fujikawa, T. Somete, and A. Mori, Tetrahedron Lett., 2010, 51, 850; CrossRef (c) M. Zhu, K.-i. Fujita, and R. Yamaguchi, Chem. Commun., 2011, 47, 12876. CrossRef
9. A copper/iodine-promoted regioselective homocoupling of 2-phenylpyridine was reported, see ref. 7c. Although CuCl2-mediated homocoupling of indoles was reported, highly basic BuLi or t-BuLi was required for the deprotonation of the C2-position of indoles; J. Bergman and N. Eklund, Tetrahedron, 1980, 36, 1439. CrossRef
10. Recent examples of the double coordination strategy in C–H activation: (a) J. A. Johnson and D. Sames, J. Am. Chem. Soc., 2000, 122, 6321; CrossRef (b) V. G. Zaitsev, D. Shabashov, and O. Daugulis, J. Am. Chem. Soc., 2005, 127, 13154; CrossRef (c) R. Giri, N. Maugel, B. M. Foxman, and J.-Q. Yu, Organometallics, 2008, 27, 1667; CrossRef (d) S. Inoue, H. Shiota, Y. Fukumoto, and N. Chatani, J. Am. Chem. Soc., 2009, 131, 6898; CrossRef (e) W. R. Gutekunst and P. S. Baran, J. Am. Chem. Soc., 2011, 133, 19076; CrossRef (f) S.-Y. Zhang, G. He, Y. Zhao, K. Wright, W. A. Nack, and G. Chen, J. Am. Chem. Soc., 2012, 134, 7313; CrossRef (g) L. D. Tran, I. Popov, and O. Daugulis, J. Am. Chem. Soc., 2012, 134, 18237; CrossRef (h) Y. Aihara and N. Chatani, J. Am. Chem. Soc., 2013, 135, 5308. CrossRef
11. (a) L. M. Huffman and S. S. Stahl, J. Am. Chem. Soc., 2008, 130, 9196; CrossRef (b) A. E. King, T. C. Brunold, and S. S. Stahl, J. Am. Chem. Soc., 2009, 131, 5044; CrossRef (c) A. E. King, L. M. Huffman, A. Casitas, M. Costas, X. Ribas, and S. S. Stahl, J. Am. Chem. Soc., 2010, 132, 12068. CrossRef
12. Although we tested N-(naphthalen-1-yl)thiophene-2-carboxamide and N-phenylindole, no corresponding homocoupling products were detected by 1H NMR and MS analysis.