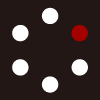
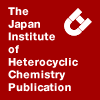
HETEROCYCLES
An International Journal for Reviews and Communications in Heterocyclic ChemistryWeb Edition ISSN: 1881-0942
Published online by The Japan Institute of Heterocyclic Chemistry
e-Journal
Full Text HTML
Received, 17th June, 2013, Accepted, 12th July, 2013, Published online, 16th July, 2013.
DOI: 10.3987/COM-13-S(S)34
■ Synthesis of the Diazepanone-Nucleoside Core Structure of Liposidomycins and Caprazamycins Based on 7-Exo Cyclization of Epoxyamine
Hiroaki Miyaoka,* Jumpei Wada, and Etsuko Kawashima
School of Pharmacy, Tokyo University of Pharmacy and Life Sciences, 1432-1 Horinouchi, Hachioji, Tokyo 192-0392, Japan
Abstract
The diazepanone-nucleoside core structure of liposidomycins and caprazamycins has been synthesized based on 7-exo cyclization of epoxyamine.Liposidomycins are a family of nucleoside antibiotics initially isolated from Streptomyces griseosporeus in 1985 (Figure 1).1 Liposidomycins inhibit formation of the lipid intermediate in peptidoglycan synthesis of Escherichia coli at IC50 = 0.03 µg/mL. In particular, liposidomycin B showed anti-mycobacterial activity against Mycobacterium phlei IFO 3158 (MIC = 1.6 µg/mL).1a Caprazamycins are a family of nucleoside antibiotics initially isolated from Streptomyces sp. MK 730-62F2 in 2003.2 Caprazamycin B showed anti-mycobacterial activity against drug-susceptible and multi drug-resistant Mycobacterium tuberculosis strains. The MICs of caprazamycin B were 3.13 µg/mL for M. tuberculosis H37Rv and Kurono strains, 6.25-12.5 µg/mL for drug-susceptible M. tuberculosis, and 6.25-12.5 µg/mL for multi drug-resistant M. tuberculosis.2a Many synthetic studies of liposidomycins and caprazamycins including syntheses of their analogues have been reported in relation to their complicated structural features and potential biological activities.3,4 Matsuda and Ichikawa’s group achieved the first total synthesis of caprazol, which represents the core structure of caprazamycins.4a,b Caprazol was obtained by hydrolysis of caprazamycin B in studies concerning the structural determination of caprazamycins.2b Furthermore, Matsuda and Ichikawa’s group synthesized various caprazamycin analogues and examined their structure-activity relationship.4c-f In this paper, the authors wish to report the synthesis of the diazepanone-nucleoside core of liposidomycins and caprazamycins based on 7-exo cyclization of epoxyamine.
The authors planned the synthesis of 5’-epi-diazepanone-nucleoside core A of liposidomycins and caprazamycins from epoxy nucleoside C, which is easily prepared. Our retrosynthetic approach for diazepanone-nucleoside A features 7-exo cyclization of the epoxyamine outlined in Scheme 1. Diazepanone-nucleoside A is synthesized by 7-exo cyclization of epoxyamine B. Epoxyamine B may likely be formed through amide formation by reaction of nucleoside segment C and amine segment D. The similar synthetic study of diazepanone-nucleoside by 7-exo cyclization has been reported by Sarabia and co-workers.3n,v
Amine 7 corresponding to amine segment D in the synthetic plan was prepared from known olefin 15 (Scheme 2). Epoxidation of olefin 1 with dimethyldioxirane (DMDO)6 afforded a diastereomeric mixture of β-epoxide 2a and α-epoxide 2b (2a : 2b = 3.4 : 1) in 93% yield. The relative configuration of β-epoxide 2a was confirmed by chemical conversion to a known compound. β-Epoxide 2a was treated with hydrogen in the presence of Pd(OH)2/C and di-tert-butyl dicarbonate (Boc2O) to give known epoxide 2c.7 Opening of the epoxide in 2a with methylamine afforded the secondary alcohol.3d The secondary alcohol was protected with the TBDPS group to give TBDPS ether 3 in 45% yield (2 steps). The amino group in 3 was protected with fluorenylmethyloxycarbonyl chloride (FmocCl) and 10% NaHCO3 aqueous to afford Fmoc carbamate 4 in quantitative yield. The acetonide in 4 was deprotected with bismuth tribromide8 to give alcohol 5 (62% yield) and acetonide 4 (30% recovered). The primary hydroxy group in 5 was protected with the TBS group to give TBS ether 6 in 98% yield. Deprotection of the Cbz group in 6 was accomplished by catalytic hydrogenation to give amine 79 in 94% yield.
Nucleoside segment C in the synthetic plan was prepared from known α,β-unsaturated ester 8.10 α,β-Unsaturated ester 8 was reduced with DIBAH in CH2Cl2 at –78 °C to afford the aldehyde (Scheme 3). The aldehyde was reduced with NaBH4 in MeOH to give the allylic alcohol. Direct reduction of α,β-unsaturated ester 8 to allylic alcohol with two and more equivalents of DIBAH resulted in an extremely low yield. The uracil base in the allylic alcohol was protected with MPMCl and DBU to give allylic alcohol 9 in 36% yield (3 steps). Epoxidation of allylic alcohol 9 was performed according to Sharpless’ procedure to afford epoxyalcohol 10 in 87% yield. In this epoxidation, the diastereomer of 10 was not obtained.
Nucleoside segment 10 and amine segment 7 were connected by formation of an amide bond. Oxidation of epoxyalcohol 10 with 2,2,6,6-tetramethylpiperidine 1-oxyl (TEMPO), NaClO and KBr gave the carboxylic acid.11 Treatment of a mixture of the carboxylic acid and amine 7 with 1-ethyl-3-(3-dimethylaminopropyl)carbodiimide hydrochloride (EDC) and 1-hydroxybenzotriazole (HOBt)12 in THF afforded amide 11 in 75% yield (based on 7). The Fmoc group of amide 11 was removed by treatment with Et3N in THF to give epoxyamine 12 in 90% yield. Epoxyamine 12 is a precursor of the diazepanone-nucleoside. A solution of epoxyamine 12 in THF was refluxed to afford diazepanone 13 in 66% yield.
The structure of diazepanone 13 was confirmed by HMBC and NOESY spectra (Figure 2). The HMBC spectrum indicated H-5’ to be correlated with C-4’’’ and H-4’’’ to be correlated with C-7’, while Me-6’-N was correlated with C-7’. On the basis of these correlations, the presence of a diazepanone ring in 13 was clarified. The absolute configuration at C-6’ in 13 was determined by the following NOESY correlations: H-6’/H-3’’’, H-6’/H-4’’’ and Me-6’-N/H-4’’’. The structure of 13 was thus determined to be that as shown.
In conclusion, the authors achieved the synthesis of diazepanone-nucleoside core 13 of liposidomycins and caprazamycins based on 7-exo cyclization of an epoxyamine. The authors plan to invert the stereochemistry of hydroxy group at C-5’ of 13. The synthesis of caprazamycins from diazepanone-nucleoside 13 is now being undertaken.
EXPERIMENTAL
Optical rotations were measured using a Jasco P-1030 polarimeter. Melting points (mp) were measured using a Yanako melting point apparatus MP-S3 and are uncorrected. IR spectra were recorded using a Jasco FT-IR/620 spectrometer. 1H and 13C NMR spectra were recorded on a Bruker DRX-400 (400 MHz) or Bruker Biospin AV-600 (600 MHz) spectrometer. Chemical shifts are given on the δ (ppm) scale using tetramethylsilane (TMS) as the internal standard (s, singlet; d, doublet; t, triplet; q, quartet; m, multiplet; br, broad). High resolution-electrospray ionization-mass spectra (HR-ESI-MS) were obtained using a Micromass LCT spectrometer. Elemental analysis data were obtained using an Elementar Vario EL.
(R)-Benzyl 2,2-dimethyl-4-((R)-oxiran-2-yl)oxazolidine-3-carboxylate (2a) and (R)-benzyl 2,2-dimethyl-4-((S)-oxiran-2-yl)oxazolidine-3-carboxylate (2b). To a cold (0 °C) solution of olefin 15 (442 mg, 1.96 mmol) in CH2Cl2 (8.45 mL) was added DMDO (78.6 mL, 3.38 mmol, 0.043 M in acetone). After stirring at room temperature for 12 h, the reaction mixture was concentrated under reduced pressure. The residue was purified by silica gel column chromatography (hexane : AcOEt = 5 : 1) to give β-epoxide 2a (339 mg, 72% yield) and α-epoxide 2b (98.2 mg, 21% yield) as pale yellow oil, respectively. 2a: [α]D25 –11.1 (c = 1.00, CHCl3); IR (neat) 2985, 1708, 1089, 1059 cm-1; 1H NMR (400 MHz, CDCl3) δ 7.40-7.30 (5H, m), 5.22-5.10 (1.4H, m), 5.04 (0.6H, d, J = 12.0 Hz), 4.08 (1H, dd, J = 9.3, 0.9 Hz), 4.01 (1H, dd, J = 9.3, 5.7 Hz), 3.63 (0.4H, m), 3.48 (0.6H, m), 3.04 (0.4H, m), 2.97 (0.6H, m), 2.89 (1H, m), 2.68 (0.4H, m), 2.48 (0.6H, m), 1.68 (1.8H, s), 1.60 (1.2H, s), 1.55 (1.8H, s), 1.47 (1.2H, s); 13C NMR (100 MHz, CDCl3) δ 153.1, 152.3, 136.0, 128.6, 128.3, 128.0, 94.7, 94.2, 67.3, 67.0, 66.3, 65.7, 59.9, 59.0, 52.1, 51.8, 48.2, 27.4, 26.5, 24.4, 23.1; ESI-MS m/z 278 (M++H, 100); HR-ESI-MS m/z 278.1407 (Calcd for C15H20NO4: M++H, 278.1392); Anal. Calcd for C15H19NO4: C, 64.97; H, 6.91; N, 5.05. Found: C, 64.95; H, 7.09; N, 5.22. 2b: [α]D25 +46.2 (c = 1.00, CHCl3); IR (neat) 2984, 1706, 1410, 1332, 1257, 1091 cm-1; 1H NMR (400 MHz, CDCl3) δ 7.40-7.20 (5H, m), 5.20-5.05 (2H, m), 4.33 (0.4H, m), 4.23 (0.6H, m), 3.85 (1H, m), 3.76 (1H, m), 3.24 (0.4H, m), 3.15 (0.6H, m), 2.67 (1H, m), 2.60 (1H, m), 1.60-1.40 (6H, m); 13C NMR (100 MHz, CDCl3) δ 153.1, 152.1, 136.2, 136.0, 128.5, 128.1, 127.9, 94.5, 94.0, 67.4, 66.8, 63.1, 56.9, 56.2, 50.8, 44.0, 27.0, 26.2, 24.4, 23.0; ESI-MS m/z 278 (M++H, 100); HR-ESI-MS m/z 278.1365 (Calcd for C15H20NO4: M++H, 278.1392); Anal. Calcd for C15H19NO4: C, 64.97; H, 6.91; N, 5.05. Found: C, 65.03; H, 7.06; N, 4.98.
(R)-tert-Butyl 2,2-dimethyl-4-((R)-oxiran-2-yl)oxazolidine-3-carboxylate (2c). To a solution of carbamate 2a (43.8 mg, 0.158 mmol) in MeOH (1.9 mL) were added Boc2O (73.5 µL, 0.320 mmol) and 20% Pd(OH)2/C (21.9 mg). After stirring at room temperature for 12 h under H2 atmosphere, the reaction mixture was filtered through a celite pad. The filtrate was concentrated under reduced pressure and the residue was purified by silica gel column chromatography (hexane : AcOEt = 4 : 1) to give known carbamate 2c7 (3.0 mg, 7% yield) and recovered carbamate 2a (20.0 mg, 46% recovered).
(R)-Benzyl 4-[(S)-1-(tert-butyldiphenylsilyloxy)-2-(methylamino)ethyl]-2,2-dimethyloxazolidine-3-carboxylate (3). A solution of epoxide 2a (3.81 g, 13.7 mmol) in methylamine (41.9 mL, 411 mmol, 9.8 M in MeOH) was stirred at room temperature for 12 h. The reaction mixture was concentrated under reduced pressure to give the crude aminoalcohol. The crude aminoalcohol was then used in the next reaction without purification.
To a solution of the above crude aminoalcohol in CH2Cl2 (6.85 mL) were added imidazole (4.66 g, 68.5 mmol) and TBDPSCl (17.9 mL, 68.5 mmol). After stirring at room temperature for 24 h, the reaction mixture was diluted with AcOEt and Et2O, and then washed with saturated aqueous NaHCO3, H2O, and saturated aqueous NaCl. The organic layer was dried over MgSO4, filtered, and concentrated under reduced pressure. The residue was purified by silica gel column chromatography (AcOEt) to give methylamine 3 (3.40 g, 45% yield, 2 steps) as a pale yellow oil. [α]D23 +41.4 (c = 1.00, CHCl3); IR (neat) 3339, 2933, 2875, 1703, 1406, 1110 cm-1; 1H NMR (400 MHz, CDCl3) δ 7.75-7.6 (4H, m), 7.45-7.1 (11H, m), 5.12 (1.5H, m), 4.99 (0.5H, m), 4.25 (3H, m), 4.00 (1H, m), 2.57 (1H, m), 2.4-2.2 (4H, m), 1.7-1.4 (7H, m), 1.09 (9H, s); 13C NMR (100 MHz, CDCl3) δ 135.8, 135.7, 134.5, 133.5, 129.6, 128.4, 128.1, 127.6, 94.8, 71.8, 71.6, 66.9, 64.0, 55.0, 35.9, 32.2, 27.1, 19.4; ESI-MS m/z 547 (M++H, 100); HR-ESI-MS m/z 547.2996 (Calcd for C32H43N2O4Si: M++H, 547.2992).
(R)-Benzyl 4-[(S)-1-(9H-fluoren-9-yl)-4,9,9-trimethyl-3-oxo-8,8-diphenyl-2,7-dioxa-4-aza-8-siladecan-6-yl]-2,2-dimethyloxazolidine-3-carboxylate (4). To a solution of methylamine 3 (3.20 g, 5.86 mmol) in 1,4-dioxane (11.7 mL) were added 10% aqueous NaHCO3 (11.7 mL) and FmocCl (1.82 g, 7.02 mmol). After stirring at room temperature for 30 min, the reaction mixture was diluted with AcOEt and Et2O, and then washed with saturated aqueous NH4Cl, H2O, and saturated aqueous NaCl. The organic layer was dried over MgSO4, filtered, and concentrated under reduced pressure. The residue was purified by silica gel column chromatography (hexane : AcOEt = 4 : 1) to give carbamate 4 (4.50 g, quantitative yield) as white crystals. mp 60-63 °C; [α]D25 +8.94 (c = 1.00, CHCl3); IR (KBr) 2933, 1701, 1405, 1092 cm-1; 1H NMR (400 MHz, CDCl3) δ 7.8-7.2 (21H, m), 7.04 (2H, m), 5.2-4.6 (3H, m), 4.5-4.0 (5H, m), 4.0-3.3 (2H, m), 2.5-2.0 (2H, m), 1.8-1.2 (8H, m), 1.09 (9H, s); 13C NMR (100 MHz, CDCl3) δ 156.2, 152.9, 144.0, 143.8, 143.6, 141.3, 135.9, 129.8, 128.8, 128.5, 128.2, 128.0, 127.6, 124.9, 124.8, 119.9, 95.4, 67.8, 67.4, 67.1, 66.9, 63.2, 58.6, 50.8, 47.3, 32.9, 26.9, 25.6, 23.6, 19.1; ESI-MS m/z 769 (M++H, 100), 547 (15); HR-ESI-MS m/z 769.3674 (Calcd for C47H53N2O6Si: M++H, 769.3673); Anal. Calcd for C47H52N2O6Si: C, 73.41; H, 6.82; N, 3.64. Found: C, 73.19; H, 6.90; N, 3.64.
(9H-Fluoren-9-yl)methyl ((2S,3R)-3-(((benzyloxy)carbonyl)amino)-2-((tert-butyldiphenylsilyl)oxy)-4-hydroxybutyl)(methyl)carbamate (5). To a solution of acetonide 4 (1.99 g, 2.59 mmol) in MeCN (13.0 mL) was added BiBr3 (117 mg, 0.26 mmol). After stirring at room temperature for 48 h, the reaction mixture was diluted with AcOEt and Et2O, and then washed with saturated aqueous NaHCO3, H2O, and saturated aqueous NaCl. The organic layer was dried over MgSO4, filtered, and concentrated under reduced pressure. The residue was purified by silica gel column chromatography (hexane : AcOEt = 2 : 1) to recover acetonide 4 (603 mg, 30%) and to give alcohol 5 (1.17 g, 62% yield) as white crystals. mp 57-60 °C; [α]D25 –7.21 (c = 1.00, CHCl3); IR (KBr) 3443, 2931, 1700, 1455, 1111 cm-1; 1H NMR (400 MHz, CDCl3) δ 7.8-7.1 (24H, m), 5.64 (0.75H, m), 5.41 (0.25H, m), 5.09 (2H, m), 4.57 (0.25H, m), 4.42 (0.25H, m), 4.32 (1.5H, m), 4.2-4.0 (3H, m), 3.98 (0.5H, m), 3.79 (0.5H, m), 3.62 (0.5H, m), 3.5-3.2 (1H, m), 2.92 (0.5H, m), 2.7-2.5 (1H, m), 2.40 (3H, m), 1.08 (9H, m); 13C NMR (100 MHz, CDCl3) δ 156.6, 156.0, 143.8, 141.2, 136.6, 136.6, 135.8, 135.8, 132.8, 132.1, 130.3, 130.2, 128.4, 128.0, 127.9, 127.8, 127.7, 127.5, 127.0, 125.0, 124.9, 119.9, 73.0, 67.6, 66.4, 61.6, 53.1, 50.8, 47.1, 33.8, 26.9, 19.3; ESI-MS m/z 729 (M++H, 100), 507 (10); HR-ESI-MS m/z 729.3377 (Calcd for C44H49N2O6Si: M++H, 729.3360); Anal. Calcd for C44H48N2O6Si: C, 72.40; H, 6.77; N, 3.96. Found: C, 72.50; H, 6.64; N, 3.84.
(9H-Fluoren-9-yl)methyl ((2S,3R)-3-(((benzyloxy)carbonyl)amino)-4-((tert-butyldimethylsilyl)oxy)-2-((tert-butyldiphenylsilyl)oxy)butyl)(methyl)carbamate (6). To a solution of aminoalcohol 5 (604 mg, 0.83 mmol) in DMF (1.66 mL) were added imidazole (170 mg, 2.49 mmol) and TBSCl (188 mg, 1.25 mmol). After stirring at room temperature for 10 min, the reaction mixture was diluted with Et2O, and then washed with saturated aqueous NaHCO3, H2O, and saturated aqueous NaCl. The organic layer was dried over MgSO4, filtered, and concentrated under reduced pressure. The residue was purified by silica gel column chromatography (hexane : AcOEt = 2 : 1) to give TBS ether 6 (684 mg, 98% yield) as a colorless oil. [α]D23 +2.02 (c = 0.89, CHCl3); IR (neat) 3335, 2930, 1724, 1705, 1472, 1111 cm-1; 1H NMR (400 MHz, CDCl3) δ 7.8-7.6 (6H, m), 7.55 (2H, d, J = 7.4 Hz), 7.5-7.25 (15H, m), 5.55 (0.5H, m), 5.2-5.0 (2.5H, m), 4.4-3.4 (8H, m), 2.82 (0.5H, m), 2.6-2.2 (3.5H, m), 1.09 (9H, s), 0.85 (9H, s), 0.02 (2H, s), 0.00 (4H, s); 13C NMR (100 MHz, CDCl3) δ 156.6, 156.3, 143.9, 141.3, 135.9, 133.2, 129.9, 128.4, 127.7, 127.7, 127.6, 127.0, 125.0, 119.9, 69.1, 67.6, 66.3, 61.4, 55.6, 50.7, 47.2, 34.1, 26.9, 25.8, 19.3, 18.1, –5.5, –5.5; ESI-MS m/z 865 (M++Na, 100), 843 (M++H, 90), 621 (60); HR-ESI-MS m/z 843.4217 (Calcd for C50H63N2O6Si2 M++H, 843.4225).
(9H-Fluoren-9-yl)methyl (2S,3R)-3-amino-4-(tert-butyldimethylsilyloxy)-2-(tert-butyldiphenylsilyloxy)butyl(methyl)carbamate (7). To a solution of carbamate 6 (13.2 mg, 15.7 µmol) in AcOEt (1.57 mL) was added 10% Pd/C (13.2 mg). After stirring at room temperature for 5 h under H2 atmosphere, the reaction mixture was filtered through a celite pad. The filtrate was concentrated under reduced pressure and the residue was purified by silica gel column chromatography (hexane : AcOEt = 1 : 1) to give amine 7 (10.4 mg, 94% yield) as a pale yellow oil. [α]D20 +6.47 (c = 0.90, CHCl3); IR (neat) 3383, 2953, 2929, 2957, 1703, 1472, 1110 cm-1; 1H NMR (400 MHz, CDCl3) δ 7.77 (2H, d, J = 7.6 Hz), 7.69 (4H, m), 7.56 (2H, m), 7.5-7.2 (10H, m), 4.35 (2H, m), 4.2-3.95 (2H, m), 3.7-3.2 (4H, m), 3.0-2.9 (1H, m), 2.64 (3H, s), 1.58 (2H, br s), 1.08 (9H, s), 0.9-0.8 (9H, m), 0.0- –0.1 (6H, m); 13C NMR (100 MHz, CDCl3) δ 156.6, 156.2, 144.0, 141.3, 135.9, 133.5, 129.8, 129.8, 127.7, 127.7, 127.6, 127.0, 125.0, 119.9, 72.7, 67.4, 64.6, 56.0, 50.7, 50.0, 47.3, 35.0, 29.7, 27.1, 25.9, 19.4, 18.1, –5.5; ESI-MS m/z 709 (M++H, 100); HR-ESI-MS m/z 709.3824 (Calcd for C42H57N2O4Si: M++H, 709.3857).
1-((3aR,4R,6R,6aR)-6-((E)-3-Hydroxyprop-1-enyl)-2,2-dimethyltetrahydrofuro[3,4-d][1,3]dioxol-4-yl)-3-(4-methoxybenzyl)pyrimidine-2,4(1H,3H)-dione (9). To a cold (–78 °C) solution of α,β-unsaturated ester 8 (5.12 g, 14.5 mmol) in CH2Cl2 (145 mL) was added dropwise DIBAH (43.5 mL, 43.5 mmol, 1.0 M in toluene). After stirring at –78 °C for 5 min, Et2O and Na2SO4•10H2O were added to the reaction mixture. The mixture was stirred at room temperature for 6 h and then filtered through a celite pad. The filtrate was concentrated under reduced pressure to give the crude aldehyde. The crude aldehyde was then used in the next reaction without purification.
To a cold (0 °C) solution of the above crude aldehyde in MeOH (19.3 mL) was added portionwise NaBH4 (219 mg, 5.80 mmol). After stirring at room temperature for 6 h, saturated aqueous NH4Cl was added to the reaction mixture. The mixture was diluted with CHCl3, and then washed with H2O and saturated aqueous NaCl. The organic layer was dried over MgSO4, filtered, and concentrated under reduced pressure to give the crude alcohol. The crude alcohol was then used in the next reaction without purification.
To a solution of the above crude alcohol in MeCN (30.4 mL) were added DBU (2.04 mL, 13.7 mmol) and MPMCl (1.48 mL, 10.9 mmol). The mixture was stirred at 60 °C for 3 h. The reaction mixture was cooled to room temperature, diluted with AcOEt and Et2O, and then washed with 1M HCl, saturated aqueous NaHCO3, H2O, and saturated aqueous NaCl. The organic layer was dried over MgSO4, filtered, and concentrated under reduced pressure. The residue was purified by silica gel column chromatography (hexane : AcOEt = 1 : 4) to give MPM amide 9 (2.24 g, 36% yield, 3 steps) as pale yellow crystals. mp: 45-47 °C; [α]D23 +52.9 (c = 1.00, CHCl3); IR (KBr) 3446, 2990, 2932, 1712, 1668, 1513, 1455, 1386, 1249, 1087 cm-1; 1H NMR (400 MHz, CDCl3) δ 7.42 (2H, d, J = 8.7 Hz), 7.18 (1H, d, J = 8.0 Hz), 6.81 (2H, d, J = 8.7 Hz), 5.94 (1H, dt, J = 15.6, 4.6 Hz), 5.83 (1H, dd, J = 15.6, 7.5 Hz), 5.74 (1H, d, J = 8.0 Hz), 5.62 (1H, s), 5.01 (2H, s), 4.96 (1H, dd, J = 6.4, 1.5 Hz), 4.73 (1H, dd, J = 6.4, 4.3 Hz), 4.55 (1H, dd, J = 7.5, 4.3 Hz), 4.13 (2H, d, J = 4.2 Hz), 3.76 (3H, s), 1.91 (1H, br s), 1.56 (3H, s), 1.34 (3H, s); 13C NMR (100 MHz, CDCl3) δ 162.5, 159.1, 150.5, 139.8, 134.1, 130.7, 128.7, 127.2, 114.4, 113.6, 102.0, 95.0, 87.9, 84.9, 84.2, 62.3, 55.2, 43.5, 27.1, 25.3; ESI-MS m/z 431 (M++H, 100), 413 (20); HR-ESI-MS m/z 431.1801 (Calcd for C22H27N2O7: M++H, 431.1818).
1-((3aR,4R,6R,6aR)-6-((2R,3S)-3-(Hydroxymethyl)oxiran-2-yl)-2,2-dimethyltetrahydrofuro[3,4-d][1,3]dioxol-4-yl)-3-(4-methoxybenzyl)pyrimidine-2,4(1H,3H)-dione (10). To a cold (–20 °C) suspension of 4A molecular sieves (2.5 g) in CH2Cl2 (20 mL) were added L-(+)-DIPT (0.16 mL, 0.78 mmol) and Ti(OiPr)4 (0.15 mL, 0.52 mmol). After stirring at –20 °C for 10 min, TBHP (0.69 mL, 10.8 mmol, 15.6 M in CH2Cl2) was added to the mixture. After stirring for 30 min at the same temperature, a solution of allylic alcohol 9 (2.24 g, 5.21 mmol) in CH2Cl2 (32.0 mL) was added to the mixture. The reaction temperature was slowly raised to room temperature. After stirring at the same temperature for 12 h, 30% NaOH solution in saturated aqueous NaCl was added to the reaction mixture. The mixture was diluted with Et2O and stirred for 30 min. A mixture of MgSO4 and celite (8 : 1) was then added. After stirring for 15 min, the mixture was filtered through a celite pad and the filtrate was concentrated under reduced pressure. The residue was purified by silica gel column chromatography (hexane : AcOEt = 1 : 7) to give epoxyalcohol 10 (2.02 g, 87% yield) as white crystals. mp 52-55°C; [α]D22 +5.95 (c = 1.04, CHCl3); IR (KBr) 3458, 2989, 2937, 1711, 1669, 1514, 1456, 1388, 1249, 1081 cm-1; 1H NMR (400 MHz, CDCl3) δ 7.42 (2H, d, J = 8.7 Hz), 7.24 (1H, d, J = 8.0 Hz), 6.82 (2H, d, J = 8.7 Hz), 5.78 (1H, d, J = 8.0 Hz), 5.73 (1H, d, J = 1.6 Hz), 5.03 (1H, d, J = 13.6 Hz), 4.99 (1H, d, J = 13.6 Hz), 4.91 (1H, dd, J = 6.6, 1.3 Hz), 4.77 (1H, m), 4.21 (1H, t, J = 4.0 Hz), 3.89 (1H, br d, J = 12.9 Hz), 3.78 (3H, s), 3.64 (1H, m), 3.35 (1H, m), 3.09 (1H, m), 1.90 (1H, br s), 1.54 (3H, s), 1.34 (3H, s); 13C NMR (100 MHz, CDCl3) δ 162.4, 159.1, 150.6, 139.4, 130.7, 128.7, 114.5, 113.7, 102.3, 94.7, 86.7, 84.8, 79.9, 60.7, 56.6, 55.2, 53.9, 43.5, 27.0, 25.2; ESI-MS m/z 447 (M++H, 100); HR-ESI-MS m/z 447.1799 (Calcd for C22H27N2O8: M++H, 447.1767).
(9H-Fluoren-9-yl)methyl (2S,3R)-4-(tert-butyldimethylsilyloxy)-2-(tert-butyldiphenylsilyloxy)-3-((2R,3S)-3-((3aR,4R,6R,6aR)-6-(3-(4-methoxybenzyl)-2,4-dioxo-3,4-dihydropyrimidin-1(2H)-yl)-2,2-dimethyltetrahydrofuro[3,4-d][1,3]dioxol-4-yl)oxirane-2-carboxamido)butyl(methyl)carbamate (11). To a cold (0 °C) solution of alcohol 10 (241 mg, 0.540 mmol) in acetone (1.2 mL) were added 5% aqueous NaHCO3 (1.5 mL), KBr (6.43 mg, 54.0 µmol), TEMPO (8.44 mg, 54.0 µmol), and NaClO (2.08 mL, 1.62 mmol, 0.78 M in H2O). After stirring at room temperature for 2 h, the reaction mixture was diluted with Et2O and AcOEt, and then washed with saturated aqueous NaH2PO4, H2O, and saturated aqueous NaCl. The organic layer was dried over MgSO4, filtered, and concentrated under reduced pressure to give the crude carboxylic acid. The crude carboxylic acid was then used in the next reaction without purification.
To a solution of the above crude carboxylic acid and amine 7 (255 mg, 0.54 mmol) in THF (0.72 mL) were added EDC (104 mg, 0.54 mmol) and HOBt (73.0 mg, 0.54 mmol). After stirring at room temperature for 12 h, the reaction mixture was diluted with Et2O and AcOEt, and then washed with saturated aqueous NaHCO3, saturated aqueous NH4Cl, H2O, and saturated aqueous NaCl. The organic layer was dried over MgSO4, filtered, and concentrated under reduced pressure. The residue was purified by silica gel column chromatography (hexane : AcOEt = 1 : 1) to give epoxyamide 11 (314 mg, 75% yield) as a colorless oil. [α]D22 +20.2 (c = 0.97, CHCl3); IR (neat) 2953, 2930, 1698, 1671, 1455, 1249, 1105 cm-1; 1H NMR (400 MHz, CDCl3) δ 7.8-7.6 (6H, m), 7.52 (2H, d, J = 7.4 Hz), 7.5-7.2 (13H, m), 6.82 (2H, d, J = 8.6 Hz), 5.80 (1H, br s), 5.74 (1H, d, J = 8.0 Hz), 5.08 (1H, d, J = 13.6 Hz), 4.96 (1H, d, J = 13.6 Hz), 4.79 (1H, m), 4.65 (1H, m), 4.4-4.1 (6H, m), 3.76 (3H, s), 3.75-3.6 (3H, m), 3.4-3.2 (3H, m), 2.85-2.5 (1H, m), 2.46 (3H, s), 1.55 (3H, s), 1.33 (3H, s), 1.08 (9H, s), 0.83 (9H, s), 0.00 (3H, s), –0.03 (3H, s); 13C NMR (100 MHz, CDCl3) δ 166.6, 162.3, 159.1, 156.6, 150.6, 143.9, 141.2, 138.5, 136.0, 135.9, 133.4, 132.9, 130.8, 130.0, 129.9, 128.8, 127.8, 127.7, 127.6, 127.0, 127.0, 124.9, 119.9, 114.9, 113.7, 102.5, 93.7, 93.7, 85.4, 84.8, 79.2, 68.8, 67.7, 60.8, 57.0, 55.2, 53.3, 53.3, 50.7, 47.1, 43.6, 34.1, 29.7, 27.1, 26.9, 25.8, 25.3, 19.3, 18.0, –5.5, –5.6; ESI-MS m/z 1151 (M++H, 100), 929 (90); HR-ESI-MS m/z 1151.5270 (Calcd for C64H79N4O12Si2: M++H, 1151.5233); Anal. Calcd for C64H78N4O12Si2: C, 66.76; H, 6.83; N, 4.87. Found: C, 66.62; H, 6.99; N, 4.90.
(2R,3S)-N-((5S,6R)-2,2,9,9,10,10-Hexamethyl-5-((methylamino)methyl)-3,3-diphenyl-4,8-dioxa-3,9-disilaundecan-6-yl)-3-((3aR,4R,6R,6aR)-6-(3-(4-methoxybenzyl)-2,4-dioxo-3,4-dihydropyrimidin-1(2H)-yl)-2,2-dimethyltetrahydrofuro[3,4-d][1,3]dioxol-4-yl)oxirane-2-carboxamide (12). To a solution of epoxyamide 11 (220 mg, 0.191 mmol) in CH2Cl2 (0.95 mL) was added Et3N (0.95 mL). After stirring at room temperature for 4 days, the reaction mixture was diluted with Et2O and AcOEt, and then washed with saturated aqueous NH4Cl, H2O, and saturated aqueous NaCl. The organic layer was dried over MgSO4, filtered, and concentrated under reduced pressure. The residue was purified by silica gel column chromatography (AcOEt) to give amine 12 (156 mg, 90% yield) as a pale yellow oil. [α]D22 +8.00 (c = 0.93, CHCl3); IR (neat) 3329, 2954, 2929, 2856, 1713, 1671, 1514, 1105 cm-1; 1H NMR (400 MHz, CDCl3) δ 8.61 (1H, d, J = 7.7 Hz), 7.65 (4H, m), 7.5-7.3 (9H, m), 7.22 (1H, d, J = 8.0 Hz), 6.82 (2H, d, J = 8.7 Hz), 5.81 (1H, d, J = 1.8 Hz), 5.77 (1H, d, J = 8.0 Hz), 5.09 (1H, d, J = 13.6 Hz), 4.97 (1H, d, J = 13.6 Hz), 4.86 (1H, d, J = 6.5 Hz), 4.72 (1H, m), 4.27 (1H, m), 4.16 (1H, m), 4.08 (1H, m), 3.77 (3H, s), 3.64 (1H, dd, J = 10.2, 4.6 Hz), 3.40 (2H, m), 3.33 (1H, m), 2.64 (2H, d, J = 3.2 Hz), 2.24 (3H, s), 1.56 (3H, s), 1.35 (3H, s), 1.06 (9H, s), 0.77 (9H, s), –0.07 (3H, s), –0.07 (3H, s); 13C NMR (100 MHz, CDCl3) δ 166.8, 162.3, 159.1, 150.7, 138.7, 135.8, 135.6, 133.9, 133.1, 130.8, 129.9, 129.8, 128.7, 127.7, 127.7, 114.8, 113.7, 102.5, 94.0, 85.6, 84.8, 79.3, 69.8, 61.9, 57.0, 55.4, 55.2, 53.4, 53.3, 43.6, 36.5, 27.1, 27.0, 25.7, 25.3, 19.3, 18.0, –5.5, –5.8; ESI-MS m/z 929 (M++H, 100), 457 (35); HR-ESI-MS m/z 929.4568 (Calcd for C49H69N4O10Si2: M++H, 929.4552).
1-((3aR,4R,6R,6aR)-6-((R)-((2S,5R,6S)-5-((tert-Butyldimethylsilyloxy)methyl)-6-(tert-butyldiphenyl-silyloxy)-1-methyl-3-oxo-1,4-diazepan-2-yl)(hydroxy)methyl)-2,2-dimethyltetrahydrofuro[3,4-d][1,3]dioxol-4-yl)-3-(4-methoxybenzyl)pyrimidine-2,4(1H,3H)-dione (13). A solution of epoxyamine 12 (6.4 mg, 6.89 µmol) in THF (6.9 mL) was refluxed for 3 days. After cooling to room temperature, the mixture was concentrated under reduced pressure. The residue was purified by silica gel column chromatography (hexane : AcOEt = 1 : 1) to give diazepanone 13 (4.2 mg, 66% yield) as a colorless oil. [α]D22 –17.0 (c = 0.77, CHCl3); IR (neat) 2953, 2931, 2857, 1707, 1668, 1456, 1249, 1101 cm-1; 1H NMR (400 MHz, CDCl3) δ 7.8-7.65 (5H, m), 7.5-7.3 (9H, m), 6.81 (2H, d, J = 8.6 Hz), 6.04 (1H, d, J = 2.5 Hz), 5.73 (1H, d, J = 8.1 Hz), 5.10 (1H, d, J = 13.6 Hz), 4.98 (1H, d, J = 13.6 Hz), 4.93 (1H, m), 4.68 (1H, m), 4.65 (1H, m), 4.32 (1H, br s), 4.07 (1H, m), 3.94 (1H, m), 3.76 (3H, s), 3.74 (1H, m), 3.64 (2H, m), 3.39 (1H, m), 2.9-2.8 (2H, m), 2.25 (3H, s), 1.61 (3H, s), 1.39 (3H, s), 1.05 (9H, s), 0.88 (9H, s), 0.05 (3H, s), 0.03 (3H, s); 13C NMR (100 MHz, CDCl3) δ 175.6, 162.7, 159.0, 151.0, 138.4, 135.9, 135.7, 133.5, 132.4, 130.8, 130.3, 130.0, 129.1, 128.0, 127.8, 113.9, 113.6, 101.7, 92.0, 86.4, 85.6, 78.9, 73.0, 68.1, 66.0, 63.2, 58.3, 55.3, 55.2, 43.5, 35.0, 27.4, 26.8, 25.9, 25.6, 19.5, 18.3, –5.2, –5.5; ESI-MS m/z 929 (M++H, 100), 457 (70); HR-ESI-MS m/z 929.4512 (Calcd for C49H69N4O10Si2: M++H, 929.4552).
References
1. (a) K. Isono, M. Uramoto, H. Kusakabe, K. Kimura, K. Izaki, C. C. Nelson, and J. A. McCloskey, J. Antibiot., 1985, 38, 1617; CrossRef (b) M. Ubukata, K. Isono, K. Kimura, C. C. Nelson, and J. A. McCloskey, J. Am. Chem. Soc., 1988, 110, 4416; CrossRef (c) K. Kimura, N. Miyata, G. Kawanishi, Y. Kamio, K. Izaki, and K. Isono, Agric. Biol. Chem., 1989, 53, 1811; CrossRef (d) M. Ubukata, K. Kimura, K. Isono, C. C. Nelson, J. M. Gregson, and J. A. McCloskey, J. Org. Chem., 1992, 57, 6392; CrossRef (e) M. Muroi, K. Kimura, H. Osada, M. Inukai, and A. Takatsuki, J. Antibiot., 1997, 50, 103; CrossRef (f) K. Kimura, S. Kagami, Y. Ikeda, H. Takahashi, M. Yoshihama, H. Kusakabe, H. Osada, and K. Isono, J. Antibiot., 1998, 51, 640; CrossRef (g) K. Kimura, Y. Ikeda, S. Kagami, M. Yoshihama, M. Ubukata, Y. Esumi, H. Osada, and K. Isono, J. Antibiot., 1998, 51, 647; CrossRef (h) K. Kimura, Y. Ikeda, S. Kagami, M. Yoshihama, K. Suzuki, H. Osada, and K. Isono, J. Antibiot., 1998, 51, 1099; CrossRef (i) S. Kagami, K. Kimura, Y. Ikeda, H. Takahashi, H. Kusakabe, H. Osada, K. Isono, and M. Yoshihama, Actinomycetol, 1998, 12, 110. CrossRef
2. (a) M. Igarashi, N. Nakagawa, N. Doi, S. Hattori, H. Naganawa, and M. Hamada, J. Antibiot., 2003, 56, 580; CrossRef (b) M. Igarashi, Y. Takahashi, T. Shitara, H. Nakamura, H. Naganawa, T. Miyake, and Y. Akamatsu, J. Antibiot., 2005, 58, 327; CrossRef (c) S. Siebenberg, L. Kaysser, E. Wemakor, L. Heide, B. Gust, and B. Kammerer, Rapid Commun. Mass Spectrom., 2011, 25, 495. CrossRef
3. (a) M. R. Spade, M. Ubukata, and K. Isono, Heterocycles, 1992, 34, 1147; CrossRef (b) S. Knapp, S. Nandan, and L. Resnick, Tetrahedron Lett., 1992, 33, 5485; CrossRef (c) K. S. Kim, I. H. Cho, Y. H. Ahn, and J. I. Park, J. Chem. Soc., Perkin Trans. 1, 1995, 1783; CrossRef (d) W. J. Moore and F. A. Luzzio, Tetrahedron Lett., 1995, 37, 6599; (e) K. S. Kim, C. S. Cheong, J. S. Hahn, and J. I. Park, Bull. Korean Chem. Soc., 1997, 18, 465; (f) Y. L. Merrer, C. Gravier-Pelletier, M. Gerrouache, and J.-C. Depezay, Tetrahedron Lett., 1998, 39, 385; CrossRef (g) K. S. Kim and Y. H. Ahn, Tetrahedron: Asymmetry, 1998, 9, 3601; CrossRef (h) S. Knapp, G. J. Morriello, S. R. Nandan, T. J. Emge, G. A. Doss, R. T. Mosley, and L. Chen, J. Org. Chem., 2001, 66, 5822; CrossRef (i) C. Gravier-Pelletier, M. Milla, Y. L. Merrer, and J.-C. Depezay, Eur. J. Org. Chem., 2001, 3039; (j) S. Knapp, G. J. Morriello, and G. A. Doss, Org. Lett., 2002, 4, 603; CrossRef (k) S. Knapp, G. J. Morriello, and G. A. Doss, Tetrahedron Lett., 2002, 43, 5797; CrossRef (l) N. Nakajima, T. Isobe, S. Irisa, and M. Ubukata, Heterocycles, 2003, 59, 107; CrossRef (m) B. Drouillat, O. Poupardin, Y. Bourdreux, and C. Greck, Tetrahedron Lett., 2003, 44, 2781; CrossRef (n) F. Sarabia, L. Martín-Ortiz, and F. J. López-Herrera, Org. Lett., 2003, 5, 3927; CrossRef (o) C. Gravier-Pelletier, M. Ginisty, and Y. L. Merrer, Tetrahedron: Asymmetry, 2004, 15, 189; CrossRef (p) S. Fukunishi, M. Ubukata, and N. Nakajima, Heterocycles, 2005, 66, 129; CrossRef (q) M. Ginisty, C. Gravier-Pelletier, and Y. L. Merrer, Tetrahedron: Asymmetry, 2006, 17, 142; CrossRef (r) B. Drouillat, Y. Bourdreux, D. Perdon, and C. Greck, Tetrahedron: Asymmetry, 2007, 18, 1955; CrossRef (s) S. Fukunishi, M. Ubukata, and N. Nakajima, Heterocycles, 2007, 73, 627; CrossRef (t) O. Monasson, M. Ginisty, J. Mravljak, G. Bertho, C. Gravier-Pelletier, and Y. L. Merrer, Tetrahedron: Asymmetry, 2009, 20, 2320; CrossRef (u) J. Mravljak, O. Monasson, B. Al-Dabbagh, M. Crouvoisier, A. Bouhss, C. Gravier-Pelletier, and Y. L. Merrer, Eur. J. Med. Chem., 2011, 46, 1582; CrossRef (v) F. Sarabia, C. Vivar-García, C. García-Ruiz, L. Martín-Ortiz, and A. Romero-Carrasco, J. Org. Chem., 2012, 77, 1328. CrossRef
4. (a) S. Hirano, S. Ichikawa, and A. Matsuda, Angew. Chem. Int. Ed., 2005, 44, 1854; CrossRef (b) S. Hirano, S. Ichikawa, and A. Matsuda, J. Org. Chem., 2007, 72, 9936; CrossRef (c) S. Hirano, S. Ichikawa, and A. Matsuda, J. Org. Chem., 2008, 73, 569; CrossRef (d) S. Hirano, S. Ichikawa, and A. Matsuda, Bioorg. Med. Chem., 2008, 16, 428; CrossRef (e) S. Hirano, S. Ichikawa, and A. Matsuda, Bioorg. Med. Chem., 2008, 16, 5123; CrossRef (f) K. Ii, S. Ichikawa, B. Al-Dabbagh, A. Bouhss, and A. Matsuda, J. Med. Chem., 2010, 53, 3793; CrossRef (g) A. P. Spork, S. Koppermann, B. Dittrich, R. Herbst-Irmer, and C. Ducho, Tetrahedron: Asymmetry, 2010, 21, 763; CrossRef (h) C. Tsukano, S. Yokouchi, A.-L. Girard, T. Kuribayashi, S. Sakamoto, T. Enomoto, and Y. Takemoto, Org. Biomol. Chem., 2012, 10, 6074; CrossRef (i) P. Gopinath, T. Watanabe, and M. Shibasaki, J. Org. Chem., 2012, 77, 9260. CrossRef
5. (a) M. Sabat and C. R. Johnson, Org. Lett., 2000, 2, 1089; CrossRef (b) R. C. Yanagita, Y. Nakagawa, N. Yamanaka, K. Kashiwagi, N. Saito, and K. Irie, J. Med. Chem., 2008, 51, 46. CrossRef
6. R. Curci, A. Dinoi, and M. F. Rubio, Pure Appl. Chem., 1995, 67, 811. CrossRef
7. J. L. Koviach, M. D. Chappell, and R. L. Halcomb, J. Org. Chem., 2001, 66, 2318. CrossRef
8. X. Cong, F. Hu, K.-G. Liu, Q. J. Liao, and Z. J. Yao, J. Org. Chem., 2005, 70, 4514. CrossRef
9. Primary amine 7 was chosen as amine segment D, because the chemical yield of the condensation reaction of amine and nucleoside segment was considered.
10. (a) J. M. Tronchet and M. J. Valero, Helv. Chim. Acta, 1979, 62, 2788; CrossRef (b) J. A. Montgomery and H. J. Thomas, J. Org. Chem., 1981, 46, 594; CrossRef (c) K. S. Kim, C. M. Kang, S. J. Kim, K. S. Jung, and C. S. Hahn, Bull. Korean Chem. Soc., 1985, 6, 350; (d) K. S. Kim, S. J. Kim, J. H. Kim, and C. S. Hahn, Bull. Korean Chem. Soc., 1989, 10, 116.
11. P. L. Anelli, C. Biffi, F. Montanari, and S. Quici, J. Org. Chem., 1987, 52, 2559. CrossRef
12. L. A. Carpino, J. Am. Chem. Soc., 1993, 115, 4397. CrossRef