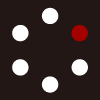
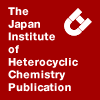
HETEROCYCLES
An International Journal for Reviews and Communications in Heterocyclic ChemistryWeb Edition ISSN: 1881-0942
Published online by The Japan Institute of Heterocyclic Chemistry
e-Journal
Full Text HTML
Received, 7th June, 2013, Accepted, 19th September, 2013, Published online, 30th September, 2013.
DOI: 10.3987/COM-13-S(S)28
■ Asymmetric Synthesis of Tertiary 2-Substituted 5-Oxotetrahydrofuran-2-carboxylic Acids
Anne Paju, Karolin Oja, Katharina Matkevitš, Priit Lumi, Ivar Järving, Tõnis Pehk, and Margus Lopp*
Department of Chemistry, Tallinn University of Technology, Akadeemia tee 15, Tallinn, Estonia
Abstract
3-Substituted 1,2-cyclopentanediones 1 were transformed to 2-substituted 5-oxotetrahydrofuran-2-carboxylic acids 2 using a catalytic process with 0.2-0.3 equivalent of Ti(OiPr)4/tartaric ester/tBuOOH complex in up to 72% isolated yield and up to 94% ee. Different functional groups in the 3-alkyl substituent of 1 like, hydroxy, ether, Boc-amino and ester groups are tolerated. Boc-aminomethyl substituents lead to β-amino acid analogues and Boc-aminoethyl substituent to γ-amino acid analogues as well as spiro-lactone-lactams. A direct, two-step procedure for homocitric acid synthesis is described.INTRODUCTION
Chiral 2-substituted 5-oxotetrahydrofuran-2-carboxylic acids are common structural units in various bioactive natural compounds like lycoperdic acid,1 aspernolides,2 monatins,3 methylisocitrate,4 sartorymensins,5 and other compounds with potential pharmacological6 and other applications.3 The particular biomedicinal interest may have spiro-lactone-lactam structures.5,6f,14
A very simple method for diastereoselective In catalyzed synthesis of tertiary lactone structures has been described by Kumar.7 There are several methods describing the synthesis of chiral tertiary γ-lactone structures, including enzymatic desymmetrization of parent esters for the synthesis of tertiary butenolides8 and protein kinase C ligands.9 In many cases chemical synthesis from natural chiral compounds is used, (e.g for the synthesis of lycoperdic acid).10 There are a few examples of the asymmetric chemical synthesis of related structures by using the chiral auxiliaries in the synthesis of crobarbatic acid and its homologues,11 and other chiral γ-butyrolactones.12 Also, chiral reagents6c or catalysts6g,13 have been used.
We have previously developed a method for the synthesis of enantiomerically enriched 2-alkyl-5-oxotetrahydrofuran-2-carboxylic acids 215 by using the asymmetric oxidation of 3-alkyl-1,2-cyclopentanediones 1 with a stoichiometric amount of Ti(OiPr)4/tartaric ester/tBuOOH complex16 (Scheme 1).
Herein we describe our attempts to develop a catalytic version of the asymmetric oxidation process and broaden of the scope of the reaction by using functionalized substituents in substrate 1, in order to obtain chiral synthons for natural compound synthesis. Using this strategy, homocitric acid lactone and spiro-lactone-lactam were synthesized.
RESULTS AND DISCUSSION
The starting 3-substituted-1,2-cyclopentanediones 1 are easily accessible compounds: the preparation of compound 1a (a R1 = Bn) has been described by us earlier;18 diketones 1b (b R1 = Me) and 1c (c R1 = Et) are commercially available. Other compounds were prepared from 3-substituted cyclopentenes by dihydroxylation, followed by Swern oxidation of the resulted diols by using our common protocols17 (Scheme 2). In many cases the most convenient starting compound for 3-substituted cyclopentenes 4 (R2 = H) was cyclopenten-3-acetic acid 5 (for compounds 1d-1h; d R1 = CH2CH2OH; e R1 = CH2CH2OBn; f R1 = CH2NHBoc; g R1 = CH2CH2NHBoc; h R1 = CH2CO2tAm). Compound 1i (R1 = Bn, R2 = OSiMe2tBu) was prepared from commercially available cis-4-cyclopentene-1,3-diol 6 by mono-acylation, protection of the OH group and Grignard replacement of the acetate, followed again by a standard reaction sequence cited above.
We have previously shown that the oxidation of 1,2-cyclopentanediones 1 consists of three steps: epoxidation (α-hydroxylation), in situ Baeyer-Villiger reaction of the resulted intermediate and acylation (Scheme 3).19 The products that may be isolated are hydroxy diketones from intermediates 7, dioxygenated products - diacids and diacid esters 9, and after hydrolysis and acylation lactone carboxylic acids 2.
Ti species participate in all steps of the cascade, catalyzing not only the oxidation but also the epoxide re-arrangement and acylation reactions. It is obvious that titanium is complexed to both, the products and the reagent. It is straightforward from Scheme 3 that a prerequisite for a catalytic reaction is the existence of equilibrium between Ti that is complexed with reaction products 8 and the substrate 1, allowing the Ti-catalyst to enter the next catalytic cycle.
We made mixtures of the substrate 1a (Figure 1, D) with Ti(OiPr)4 in CH2Cl2 at different substrate/Ti ratios and recorded the NMR spectra of the resulted solutions. The experimental NMR data show that Ti(OiPr)4 forms with substrate 1a with a ratio of 1:2 in single clearly distinguishable complex, the spectrum of which is presented in Figure 1, A. Adding of the oxidation reagent, tBuOOH to the complex not only initiates the oxidation reaction, but also changes the initial complex, releasing free substrate 1a to the reaction medium (Figure 1, B and C). These results may indicate that the complexes of Ti with tBuOOH and the reaction products are more stable than these with substrate 1a.
Having this discouraging information, we made an attempt to perform the oxidation of 3-benzyl-1,2-cyclopentanedione 1a with tBuOOH in the presence of various amounts of Ti(OiPr)4, keeping the ratio of Ti to (+)-diethyl tartrate constant in 1:1.6 . The reaction was quenched with basic water to hydrolyze the formed esters to diacids, and then subjected to acidic treatment to lactonize the hydroxy diacids. The obtained results are presented in Table 1.
The data reveal that by reducing the amount of Ti (together with (+)-diethyl tartrate) to 0.5 equivalents towards substrate 1a from the initial 1:1 amount, a slight reduction of the yield and stereoselectivity occurs (Table 1, Entries 1 and 2). Further reduction of the Ti/substrate ratio to 0.3 caused some additional reduction in the yield and stereoselectivity (Table 1, Entries 4, 5). Even at 0.2 ratio 69% yield and 91% ee were obtained. Also, we observed that anhydrous reaction conditions are preferable for the non-stoichiometric process, affording slightly better selectivity 91% vrs 93% (Table 1, Entries 4 and 5).
Reduction of the substrate Ti/1a ratio from 1 to 0.2 causes 5% reduction in the stereoselectivity and 14% in the yield. Keeping in mind that reduction of the catalyst fivefold may considerably simplify separation of the products, this result may be acceptable for some industrial purposes.
It is clearly seen that 0.1 eq of Ti is not sufficient for an efficient and selective process, causing already a considerable loss in the yield and stereoselectivity. Also, it is not possible to change the ratio of Ti/tartaric ester by reducing the amount of the chiral ligand because of drastic reduction in stereoselectivity and yield (Table 1, Entry 3).
We applied the found conditions (0.3 equivalents of Ti(OiPr)4 and 0.48 equivalents of chiral ligand (+)-DET towards substrate) for oxidation of differently substituted substrates 1. The formed lactone carboxylic acids were isolated and their stereoisomeric purities were determined by means of chiral HPLC. The obtained results are presented in Table 2, together with a reference of the corresponding values for a stoichiometric process.
The asymmetric oxidation method of 3-substituted 1,2-diones with Ti/tartaric ester/tBuOOH complex is quite universal: different functional groups like alkyl, benzyl, hydroxyl, ether, Boc-amino and ester groups are tolerated. In most cases the stoichiometric process affords the isolated yield of lactone acid ~ 70% or higher. The most remarkable feature of the process is its toleration of strong electron donating groups like Boc-aminoalkyl and ester groups. In both cases high selectivity with satisfactory yield was obtained (Table 2, Entries 8-10). The aminomethyl lactone acid 1f has once before been detected and characterized by MS.20 According to the best of our knowledge, aminoethyl lactone acid as well as its Boc-derivative have not been synthesized before.
The catalytic process with 0.3 Ti/substrate ratio affords slightly lower yields and selectivity than the stoichiometric process. However, in all cases the yields and the enantiomeric purities of the products are satisfactory also for the catalytic process (yield ~60% or higher and ee ~90%).
The racemic substrate 1i reveals very high stereoselectivity for the oxidation of the enol double bond for both enantiomers: the resulted diastereomers had high enantiomeric purities. It means that the configuration of the protected OH group did not influence the stereoselectivity of oxidation: both enantiomers reacted with similar rate and the observed ratio of diastereomers was 1:1.
The direct cascade oxidation of 3-substituted 1,2-cyclopentanediones opens an attractive approach for the short and direct synthesis of different chiral compounds of interest. Earlier we have used a stoichiometric oxidation process for the synthesis of a natural compound homocitric acid by using hydroxyethyl-substituted diketone 1d or acetic ester substituted diketone 1h.21 Now we have applied an improved protocol that enables the obtaining of homocitric acid in a two-step process using catalytical reaction conditions. Thus, diketone 1h was subjected to asymmetric oxidation by using a standard catalytic procedure with 0.3 equivalents of Ti(OiPr)4, to afford lactone acid 1h in 58% yield and 94% ee (Table 2, Entry 10). After acidic hydrolysis of the t-amyl ester with conc. HCl, homocitric acid was obtained in 88% yield.
Lactone acids 2f and 2g are prospective candidates for the synthesis of analogues of β- and γ-amino acid analogues, correspondingly. We transformed lactone acid 2g to spiro-γ-lactone-γ-lactam 10 by lactamization of 2g, followed by removal of the Boc-group, in 59% overall yield (Scheme 4).
The obtained structure may serve as a new chiral synthon for further transformations. This type of skeleton is known in many bioactive compounds.
EXPERIMENTAL
General
1H and 13C spectra were recorded in deuterated solvents on a Bruker AMX-500 or Avance II 400 spectrometer. Deuterated solvent peaks were used as references. 2D FT methods were used for the full assignment of 1H and 13C chemical shifts. Mass spectra were measured on a Shimadzu GCMS – QP 2010 spectrometer using EI (70 eV). IR spectra were recorded on a Perkin-Elmer Spectrum BX FTIR spectrometer. Elemental analyses were performed on a Perkin-Elmer 2400 Analyzer. Optical rotations were measured using a Krüss Optronic GmbH polarimeter P 3002 or Anton Paar GWB polarimeter MCP 500. High resolution mass spectra were obtained on an Accurate-Mass Q-TOF LC/MS instrument Agilent Technologies 6450 UHD by using AJ-ESI ionization. TLC was performed using DC-Alufolien Kieselgel 60 F254 (Merck) silica gel plates. For column chromatography silica gel KSK 40-100 μm and 100-160 μm was used. All reactions sensitive to oxygen or moisture were conducted under argon atmosphere in oven-dried glassware. Commercial reagents were generally used as received. THF was distilled from LiAlH4 before use. CH2Cl2 was distilled from CaH2 and stored over 3Ǻ molecular sieve pellets. DMF and tAmOH was distilled from CaH2.
(2-Cyclopent-2-enylmethyl)carbamic acid tert-butyl ester 4f
For the synthesis of compound 4f one-pot Curtius rearrangement was used.22 To a solution of cyclopent-2-enylacetic acid 5 (1.134 g, 9 mmol), NaN3 (2.046 g, 33 mmol), TBAB (435 mg, 1.35 mmol) and zinc triflate (108 mg, 0.297 mmol) in THF (90 mL) at 45 °C di-tert-butyl dicarbonate (2.364 g, 9.9 mmol) was added. The reaction mixture was stirred at 45 °C for 20 h. The mixture was cooled to room temperature and 20% aqueous solution of NaNO2 (75 mL) and EtOAc (80 mL) was added. After stirring for 20 min at room temperature the layers were separated and the aqueous phase was extracted with EtOAc (2x50 mL). The combined extracts were washed with saturated NH4Cl (2x40 mL), with saturated aqueous NaHCO3 (50 mL), with brine (50 mL) and dried (Na2SO4). The solvents were removed under reduced pressure and the residue was purified by flash chromatography (silica gel, petroleum ether/ EtOAc 25:1 to 10:1) giving 4f as a white solid (1.225 g, 69%); mp 46-48 °C; 1H NMR (500 MHz, CDCl3): δ 5.82 (qd, J=3x2.2 and 5.6 Hz, 1H, H-3), 5.62 (qd, J=3x2.2 and 5.6 Hz, 1H, H-2), 4.57 (bs, 1H, NH), 3.17 and 3.06 (m, 2H, H-6), 2.87 (m, 1H, H-1), 2.37 and 2.31 (m, 2H, H-4), 2.01 (tdd, J=2x8.8, 5.2 and 13.6 Hz, 1H, H-4), 1.51 (tdd, J=2x5.9, 9.2 and 13.6 Hz, 1H, H-4), 1.44 (s, 9H, H-9); 13C NMR (125 MHz, CDCl3): δ 156.13 (C-7), 132.75 (C-2), 131.85 (C-3), 79.01 (C-8), 46.00 (C-6), 44.81 (C-1), 32.02 (C-3), 28.39 (C-9), 26.93 (C-4); IR (KBr, cm-1): 3339, 3959, 2983, 2865, 1682, 1538, 1437, 1391, 1365, 1276, 1253, 1174, 1141, 1081, 989; MS (m/z): 198, 182, 141, 130, 124, 97, 80, 67, 57 (base); HRMS: Calcd for C11H19NO2Na [M+Na]+ 220.1308, found 220.1317.
(2-Cyclopent-2-enylethyl)carbamic acid tert-butyl ester 4g
To a solution of 2-cyclopent-2-enyl-ethanol17a (2.24 g, 20mmol) in CH2Cl2 (100 mL), Et3N (3.9 mL, 28 mmol) and methanesulfonyl chloride (1.856 mL, 24 mmol) at 0 °C were added and the mixture was stirred for 3.5 h at 0 °C. Water (200 mL) and 1N HCl solution (4 mL) were then added and the aqueous phase was extracted with CH2Cl2 (1x80 mL and 1x60 mL). The combined extracts were washed with brine (80 mL), dried on Na2SO4, the solvent was removed under reduced pressure and the residue was purified by flash chromatography (silica gel, petroleum ether/ EtOAc 5:1) giving 3.61 g (95%) of methanesulfonic acid 2-cyclopent-2-enylethyl ester. The mixture of obtained ester (1.71 g, 9 mmol) and NaN3 (1.024 g, 15.75 mmol) in DMF (13.5 mL) was stirred for 2 h at 50 °C and for 1.5 h at 70 °C. After cooling water (80 mL) was added and the mixture extracted with petroleum ether (80 mL), the extract was dried over Na2SO4. The solvent was removed under reduced pressure to yield a crude azide (1.138 g). For the conversion of azide functionality to Boc protected amine a literature procedure was used.23 To the azide (1.138 g, 8.3 mmol) in the mixture of THF (40 mL) and water (40 mL), triphenylphosphine (4.364 g, 16.6 mmol) was added and the mixture was stirred for 2.5 h at room temperature. Then, the reaction was cooled to 0 °C, Et3N (1.83 mL, 13.3 mmol) and di-tert-butyl dicarbonate (2.69 g, 12.7 mmol) were added dropwise. After stirring for 5.5 h at room temperature, water (40 mL) and Et2O (40 mL) were added, the layers were separated and the aqueous phase was extracted with Et2O (2x40 mL). The combined extracts were washed with brine (40 mL), dried (Na2SO4) and the solvent was removed. The residue was dissolved in pentane (25 mL), the precipitate was removed by filtration and the filtrate was concentrated. Flash chromatography (silica gel, petroleum ether/acetone 100:1 to 25:1) afforded 4g as a colorless oil (1.446 g, 76% from methanesulfonic acid 2-cyclopent-2-enylethyl ester), which physical and spectroscopic properties correspond to the data given in literature.24
Cyclopent-2-enylacetic acid 1,1-dimethylpropyl ester 4h
Cyclopent-2-enylacetic acid methyl ester was synthesized according to literature procedure26 from cyclopent-2-enylacetic acid 5. For the synthesis of compound 4h transesterification process was used.27 To a solution of cyclopent-2-enylacetic acid methyl ester (1.54 g, 10 mmol) in tAmOH (5.45 mL), Li-pieces (7 mg, 1 mmol) was added and the mixture was heated at 130 °C for 1.5 h. Then the temperature was raised to 140 °C and MeOH/tAmOH azeotropic mixture (1 mL) was removed. The reaction was cooled, tAmOH (1 mL) was added and the mixture was again heated at 130 °C for 1.5 h, followed by removal of azeotrope. This procedure was repeated four times. Finally, the volatiles were removed at 140-150 °C and the reaction mixture was cooled. Water (5 mL) was added and extracted with petroleum ether (3x6 mL), the extracts were washed with water (5 mL), dried (MgSO4) and concentrated. Flash chromatography (silica gel, petroleum ether/Et2O 60:1 to 50:1) afforded 4h as a colourless oil (1.2 g, 61%); 1H NMR (500 MHz, CDCl3): δ 5.73 (qd, J=3x2.2 and 5.6 Hz, 1H, H-3), 5.65 (qd, J=3x2.2 and 5.6 Hz, 1H, H-2), 3.04 (m, 1H, H-1), 2.34 and 2.28 (m, 2H, H-4), 2.29 (dd, J=6.8 and 14.7 Hz, 1H, H-6), 2.19 (dd, J=8.1 and 14.7 Hz, 1H, H-6), 2.10 (m, 1H, H-5), 1.76 (q, J=7.5 Hz, 2H, H-10), 1.45 (m, 1H, H-5), 1.41 (s, 6H, H-9), 0.87 (t, J=7.5 Hz, 3H, H-11); 13C NMR (125 MHz, CDCl3): δ 172.21 (C-7), 133.90 (C-2), 131.12 (C-3), 82.47 (C-8), 42.24 (C-1), 41.73 (C-6), 33.44 (C-10), 31.80 (C-4), 29.53 (C-5), 25.53 (C-9), 8.16 (C-11); IR (neat, cm-1): 3053, 2975, 2852, 1730, 1462, 1368, 1265, 1145, 1061, 948, 836, 724; MS (m/z): 181, 167, 126, 109, 108, 79, 71, 70, 67 (base), 66, 55, 43, 41, 39.
General procedure for the synthesis of 1,2-cyclopentanediones 1f-i
(2-Cyclopent-2-enylmethyl)carbamic acid tert-butyl ester 4f (2.23 g, 11.3 mmol) was dissolved in a tBuOH/H2O mixture 3:1 (38 mL), fiber bound OsO4 catalyst (7.5% OsO4, 38 mg, 0.0113 mmol) and NMO (50 wt. % solution in water, 3.1 mL, 14.7 mmol) were added. The reaction mixture was stirred at 60 °C for 8 days (1-4 days for the other alkenes), the catalyst was filtered off, rinsed with EtOAc (3x10 mL) and aqueous solution of 10% Na2S2O3 (40 mL) was added. The aqueous layer was extracted with EtOAc (2x50 mL and 1x30 mL), the combined extracts were washed with brine (40 mL), dried (Na2SO4) and the solvents were removed under reduced pressure. Purification of the residue by flash chromatography (silica gel, petroleum ether/acetone 10:2 to 10:4) afforded 1.855 g, (71%) of the diol 3f. The same procedure was used for diols 3g-i. Thus, diols 3g (2.153 g, 91%), 3h (1.05 g, 84%) and 3i (2.493 g, 92%) were obtained from alkenes 4g (2.015 g, 9.5 mmol), 4h (1.05 g, 5.4 mmol) and 4i28 (2.419 g, 8.4 mmol), respectively. The diols were oxidized as followed: to a solution of DMSO (1.81 mL, 23 mmol) in CH2Cl2 (108 mL), TFAA (3.25 mL, 19 mmol) was added dropwise at -60 °C. The mixture was stirred for 10 min followed by the addition of the above diol (1.855 g, 8 mmol) in a DMSO/ CH2Cl2 mixture 1:2 (8 mL). After stirring for 1.5 h at -60 °C, Et3N (7.4 mL, 53 mmol) was added at -60 °C and the mixture was stirred for 1.5 h at that temperature. Then the reaction mixture was warmed to ca. 5 °C, poured into a cold 1N HCl solution (220 mL) and the aqueous layer was extracted with CH2Cl2 (2x80 mL). The combined extracts were washed with water (200 mL), dried (Na2SO4) and the solvent was evaporated. The residue was dissolved in CH2Cl2 (80 mL), K2CO3 (373 mg, 2.7 mmol) was added and the mixture was stirred for 21 h at room temperature. Then, 1N HCl solution (45 mL) was added, stirred for 10 min and the aqueous layer was extracted with CH2Cl2 (2x40 mL). The combined extracts were washed with water (50 mL), dried (MgSO4) and the solvent was evaporated. The residue was purified by flash chromatography (silica gel, petroleum ether/ EtOAc 10:5 to 10:6) giving the target compound.
(2-Hydroxy-3-oxocyclopent-1-enylmethyl)carbamic acid tert-butyl ester 1f
Obtained as a white solid (1.458 g, 80%); mp 134-136 °C; 1H NMR (500 MHz, CDCl3): δ 7.30 (bs, 1H, OH), 5.29 (bs, 1H, NH), 4.07 (bd J=6.0Hz, 2H, H-6), 2.47 (m, 2H, H-5, 2.42 (m, 2H, H-4), 1.43 (s, 9H, H-9); 13C NMR (125 MHz, CDCl3): δ 203.76 (C-3), 156.54 (C-7), 149.47 (C-2), 142.99 (C-1), 80.07 (C-8), 38.71 (C-6), 31.91 (C-4), 28.23 (C-9), 23.92 (C-5); IR (KBr, cm-1): 3365, 3337, 2988, 1703, 1684, 1664, 1523, 1410, 1398, 1368,1283, 1251, 1192, 1164, 1111; MS (m/z): 171, 154, 127, 111, 110, 84, 82, 57 (base). Anal. Calcd for C11H17O4N: C, 58.14; H, 7.54; N, 6.16. Found: C, 58.10; H, 7.59; N, 6.15.
[2-(2-Hydroxy-3-oxocyclopent-1-enyl)ethyl]carbamic acid tert-butyl ester 1g
Diketone 1g was obtained from diol 3g (1.114 g, 4.55 mmol) as a white solid (0.722 g, 66%), which physical and spectroscopic properties correspond to the data given in literature.25
(2-Hydroxy-3-oxocyclopent-1-enyl)acetic acid 1,1-dimethylpropyl ester 1h
Diketone 1h was obtained from diol 3h (1.48 g, 6.43 mmol) as a white solid (0.766 g, 53%), mp 71-72 °C; 1H NMR (500 MHz, CDCl3): δ 6.85 (s, 1H, OH), 3.38 (s, 2H, CH2CO), 2.53 (m, 2H, H-5), 2.43 (m, 2H, H-4), 1.75 (q, J=7.3Hz, 2H, CH2CH3), 1.42 (s, 6H, (CH3)2), 0.86 (t, J=7.3Hz, 3H, CH2CH3). 13C NMR (125 MHz, CDCl3): δ 203.16 (C-3), 168.80 (COO), 150.04 (C-2), 138.61 (C-1), 84.24 (OC(Me)2), 35.42 (CH2CO), 33.36 (CH2CH3), 32.01 (C-4), 25.36 (OC(Me)2 and C-5), 8.09 (CH3CH2).; IR (KBr, cm-1): 3316, 2979, 2938, 2885, 1727, 1700, 1665, 1465, 1415, 1386, 1346, 1193, 1150, 1007, 844, 696; MS (m/z): 226, 156, 139, 111, 71, 55, 43 (base); HRMS: Calcd for C12H18O4Na [M+Na]+ 249.1097, found 249.1101.
3-Benzyl-4-(tert-butyldimethylsilanyloxy)-2-hydroxycyclopent-2-enone 1i
Dikeone 1i was obtained from diol 3i (2.075 g, 6.4 mmol) as a white solid (1.532g, 75%) , mp 92-96 °C, 1H NMR (400 MHz, CDCl3): δ 7.36 – 7.15 (m, 5H, Bn), δ 6.22 (s, 1H, OH), δ 4.67 (m, 1H, H-4), δ 3.76 (dd, J = 186.1, 14.4 Hz, 2H, Bn-CH2), δ 2.74 and 2,27 (both dd, J = 19.5 Hz, 2H, 5-H), δ 0.90 (s, 9H, tBu), δ 0.03 (s, 6H, 2 CH3), 13C NMR (101 MHz, CDCl3) δ 200.19 (C-1), 149.56 (C-2), 145.17 (C-3), 137.80 (sBn), 129.13 (o-Bn), 128.71 (m-Bn), 126.66 (p-Bn), 66.97 (C-4), 43.35 (C-5), 31.18 (Bn-CH2), 25.87 (tBu CH3), 18.10 (tBu C), -4.30 (CH3), -4.85 (CH3).; IR (KBr, cm-1): 3248, 3084, 1711, 1671, 1601, 1454, 1256, 1112, 1072, 835, 778, 757, 696; MS (m/z, %):186, 158 (base), 129, 115, 105, 91, 77, 66, 51, 41; Anal. Calcd for C18H26O3Si: C, 67.88; H, 8.23. Found: C, 67.76; H, 8.30.
General procedure for catalytic asymmetric oxidation of 1,2-cyclopentanediones 1a-i
To a solution of Ti(OiPr)4 (0.18 mL, 0.6 mmol) and 4Å powdered molecular sieves (200 mg) in CH2Cl2 (5 mL), (+)-DET (0.164 mL, 0.48 mmol) was added at -20 °C and the mixture was stirred for 15 min. Then, cyclopentanedione (2 mmol) in CH2Cl2 (3.0 mL) was added and the reaction mixture was stirred for 30 min. Next tBuOOH (0.85 mL, 5 mmol, 5.85M solution in decane) was added and the reaction was kept at -20 °C for 48 h. Water (6.0 mL) was added and the mixture was stirred for 1 h at room temperature, then 1.8 mL of 30% aqueous NaOH in saturated aqueous NaCl solution was added and the mixture was again stirred at room temperature for an additional 1 h. The CH2Cl2 layer was removed and the mixture was acidified with 1M HCl solution (pH=1-2) and extracted with EtOAc. The combined extracts were dried over MgSO4 and the solvent was evaporated. The residue was dissolved in CH2Cl2 (40 mL) and concentrated HCl solution (0.4 mL) was added (in the case of 2h a catalytic amount of pTsOH was used as the acid and for 2f, 2g, 2i thermal cyclization in toluene was used) and the mixture was stirred for 2 h at room temperature. Then, 20 mL of water was added and the CH2Cl2 layer was separated. The water layer was extracted with EtOAc and the combined extracts were dried over MgSO4. After evaporation of the solvents, the residue was purified by flash chromatography to give the corresponding γ-lactone acids 2. Lactone acid 1a-e correspond to the data given in literature.15b,15c,16b
(2R)-2-(tert-Butoxycarbonylaminomethyl)-5-oxotetrahydrofuran-2-carboxylic acid 2f
Lactone acid 2f was obtained as a white solid (196 mg, 38%); mp 138-139 °C; [α]D22 +9.3 (c 2.3, CHCl3-MeOH 1:1); ee 92%; 1H NMR (500 MHz, CDCl3+CD3OD): δ 3.61 and 3.56 (2d, J = 14.6 Hz, 2H, H-6), 2.64 (td, J = 2x9.7 and 18.1 Hz, 1H, H-4,), 2.54 (ddd, J = 4.0, 9.9 and 18,1 Hz, 1H, H-4), 2.36 (ddd, J = 4.0, 9.7 and 13.5 Hz, 1H, H-3), 2.27 (ddd, J = 9.7, 9.9 and 13.5 Hz, 1H, H-3), 1.38 (s, 9H, H-9); 13C NMR (125 MHz, CDCl3+CD3OD): δ 177.07 (C-5), 172.34 (COOH), 156.85 (C-7), 86.07 (C-2), 80.28 (C-8), 44.69 (C-6), 28.29 (C-9), 28.26 (C-3), 28.21 (C-4); IR (KBr, cm-1): 3386, 2984, 2939, 2613, 1797, 1785, 1748, 1656, 1537, 1464, 1392, 1290, 1260, 1185, 1161, 1098956, 922. 854, 774; HRMS: Calcd. for C11H16NO6 [M-H]- 258.0983, found 258.0990.
(2R)-2-(2-tert-Butoxycarbonylaminoethyl)-5-oxotetrahydrofuran-2-carboxylic acid 2g
Cyclopentanedione 1g (237 mg, 0.98 mmol) was oxidized according to general procedure to give 2g as a white solid (177 mg, 66%); mp 139-141 °C; [α]D25 -14.0 (c 2.51, MeOH); ee 92%; 1H NMR (500 MHz, CDCl3): δ 6.99 (bt, J = 5.7 Hz, 1H, NH), 3.29 and 3.18 (2m, 2H, H-7), 2.70 and 1.69 (2m, 2H, H-6), 2.53 and 2.52 (2m, 2H, H-4), 2.49 and 2.15 (2m, 2H, H-3), 1.43 (s, 9H, H-10); 13C NMR (125 MHz, CDCl3): δ 175.93 (C-5), 174.93 (COOH), 158.33 (C-8), 83.66 (C-2), 81.61 (C-9), 36.91(C-7), 36.70 (C-6), 32.99 (C-3), 27.98 (C-10), 27.72 (C-4); IR (KBr, cm-1): 3406, 2982, 2591, 1784, 1526, 1370, 1254, 1174, 1197, 1029, 901, 865, 780; HRMS: Calcd. for C12H18NO6 [M-H]- 272.1140, found 272.1142.
(2R)-2-(1,1-Dimethylpropoxycarbonylmethyl)-5-oxotetrahydrofuran-2-carboxylic acid 2h
Lactone acid 2h was obtained as a white solid (301 mg, 58%); mp 38-40 °C; [α]D25 -18.3 (c 4.74, CHCl3); ee 94%; 1H NMR (400 MHz, CDCl3): δ 9.91 (br s, 1H, OH), 3.11 and 2.86 (2d, 2H, J = 16.8 Hz, CH2CO), 2.76-2.53 (m, 3H, H-4, H-3), 2.36-2.28 (m, 1H, H-3), 1.75 (q, J = 7.5 Hz, 2H, CH2CH3), 1.39 (s, 6H, (CH3)2), 0.85 (t, J = 7.5 Hz, CH2CH3); 13C NMR (101 MHz, CDCl3) δ 175.84 (C-5), 175.56 (COOH), 167.81 (CH2CO), 85.33 (OC(CH3)2), 82.91 (C-2), 42.84 (CH2CO), 33.33 (CH2CH3), 31.41 (C-3), 27.82 (C-4), 25.52 (CH2CH3), 25.50 (CH2CH3), 8.25 (CH3CH2); IR (KBr, cm-1): 3445, 2981, 2945, 2886, 2595, 1786, 1730, 1464, 1388, 1371, 1187, 1152, 1069, 947, 842; HRMS: Calcd. for C12H17O6 [M-H]- 257.1031, found 257.1040.
(2S,3R)-2-Benzyl-3-(tert-butyldimethylsilanyloxy)-5-oxotetrahydrofuran-2-carboxylic acid 2i and (2S,3S)-2-Benzyl-3-(tert-butyldimethylsilanyloxy)-5-oxotetrahydrofuran-2-carboxylic acid 2i’
Cyclopentanedione 1i (414 mg, 1.3 mmol) was oxidized according to the general procedure to give 2i and 2i’ as white solids in 1:1 ratio (238 mg, 52%). 2i: mp 99-102 °C; [α]D25 -23.5 (c 2.07, CHCl3); ee 95%; 1H NMR (400 MHz, CDCl3) δ 7.44-7.10 (m, 5H, Bn), 4.52-4.34 (m, 1H, H-3), 3.28 (dd, J = 81.6, 14.5 Hz, 2H, Bn-CH2), 2.46 and 2.25 (2dd, J = 24.2 Hz, 2H, H-4), 0.87 (s, 9H, t-Bu), 0.09 (d, J = 23.5 Hz, 6H, 2 Si-CH3). 13C NMR (101 MHz, CDCl3) δ 174.36 (C-5), 170.51 (COOH), 133.99 (p-Bn), 130.54 (m-Bn), 128.67 (o-Bn), 127.49 (s-Bn), 91.04 (C-2), 72.44 (C-3), 40.12 (Bn-CH2), 38.03 (C-4), 25.44 (t-Bu CH3), 17.79 (t-Bu C), -4.74 (Si-CH3), -5.20 (Si-CH3). IR (KBr, cm-1): 3034, 2860, 1498, 1262, 1082, 781. Anal. Calcd for C18H26O5Si: C, 61.69; H, 7.48. Found: C, 61.65; H, 7.52.
2i’: mp 110-113 °C; [α]D25 -13.3 (c 0.72, CHCl3); ee 95%; 1H NMR (400 MHz, CDCl3) δ 7.41 – 7.13 (m, 5H, Bn), 4.77-4.63 (m, 1H, H-3), 3.43 – 3.08 (m, 2H, Bn-CH2)), 2.81 and 2.39 (2dd, J = 19.1Hz, 2H, H-4), 0.96 (s, 9H), 0.20 (d, J = 15.4 Hz, 6H, 2 Si-CH3). 13C NMR (101 MHz, CDCl3) δ 175.13 (C-5), 171.66 (COOH), 134.73 (p-Bn), 130.08 (m-Bn), 127.83 (o-Bn), 126.67 (s-Bn), 91.58 (C-2), 72.96 (C-3), 38.44 (C-4), 37.52 (Bn-CH2), 25.22 (t-Bu CH3), 17.74 (t-Bu C), -5.39 (Si-CH3), -5.50 (Si-CH3). IR (KBr, cm-1): 3483, 1770, 1705, 1497, 1254, 1085, 833, 706; HRMS: Calcd. for C18H27O5Si [M+H]+ 351.1622, found 351.1631.
(R)-1-Oxa-7-azaspiro[4.4]nonane-2,6-dione 10
(2R)-2-(tert-Butoxycarbonylamino-methyl)-5-oxotetrahydrofuran-2-carboxylic acid 2g (76 mg, 0.26 mmol) was dissolved in toluene and boiled at reflux for 9 h. After cooling, the toluene was removed under reduced pressure and the residue was purified by flash chromatography (silica gel, petroleum ether/acetone 10:2) giving Boc-protected spirolactam (46 mg). To the solution of obtained protected compound (46 mg, 0.18 mmol) in CH2Cl2 (10 mL) concentrated HCl solution (0.1 mL) was added and the mixture was stirred for 23 hours at room temperature. Then, 6 mL of water was added and the water layer was extracted several portions with CH2Cl2, dried (Na2SO4) and concentrated. Flash chromatography (silica gel, petroleum ether/ acetone 10:5 to 10:6) gave 10 as a white solid (24 mg, 59%) from lactone acid 2g; mp 158-160 °C; [α]D22 +124.2 (c 2.63, CHCl3); ee 92%; 1H NMR (400 MHz, CDCl3): δ 7.26 (br s, 1H, NH), 3.53-3,47 (m, 1H, H-8), 3.39-3.33 (m, 1H, H-8), 2.97-2.88 (m, 1H, H-3) ,2.61-2.50 (m, 3H, H-3, H-4, H-9), 2.28-2.11 (m, 2H, H-9, H-4); 13C NMR (101 MHz, CDCl3) δ 176.11 (C-2), 174.83 (C-6), 84.81 (C-5), 38.84 (C-8), 33.45 (C-9), 29.82 (C-4), 28.74 (C-3); IR (KBr, cm-1): 3253, 2966, 1776, 1717, 1672, 1459, 1437, 1301, 1257, 1197, 1179, 1138, 1113, 1076, 1053, 1012, 982, 912, 769, 690; MS (m/z): 155, 137, 127, 113, 110, 100, 98, 84, 70, 56 (base), 55; HRMS: Calcd. for C7H10NO3 [M+H]+ 156.0655, found, 156.0651.
ACKNOWLEDGEMENTS
The authors are grateful to Prof. Victor Sniečkus for helpful discussions, suggestions and encouragement during a long period of time. The authors thank the Estonian Ministry of Education and Research (Grants No. 0140060s12 and ESF8880) and EU European Regional Development Fund (3.2.0101.08-0017) for financial support.
References
1. N. R-Banga, A. Welter, J. Jadot, and J. Casimir, Phytochemistry, 1979, 18, 482.
2. R. R. Parvatkar, C. D’Souza, A. Tripathi, and C. G. Naik, Phytochemistry, 2009, 70, 128. CrossRef
3. R. Vleggaar, L. G. J. Ackerman, and P. S. Steyn, J. Chem. Soc., Perkin Trans. 1, 1992, 3095. CrossRef
4. S. Textor, V. F. Wendisch, A. A. DeGraaf, U. Müller, M. I. Linder, and W. Buckel, Arch. Microbiol., 1997, 168, 428. CrossRef
5. S. Buttachon, A. Chandrapatya, L. Manoch, A. Silva, L. Gales, C. Bruyère, R. Kiss, and A. Kijjoa, Tetrahedron, 2012, 68, 3262. CrossRef
6. a) P. Macheboeuf, D. S. Fischer, T. Brown, A. Zervosen, A. Luxen, B. Joris, A. Dessen, and C. J. Schofield, Nature Chem. Biol., 2007, 3, 565; CrossRef b) F. Wångsell, F. Russo, J. Sävmarker, Å. Rosenquist, B. Samuelsson, and M. Larhed, Bioorg. Med. Chem. Lett., 2009, 19, 4711; CrossRef c) O. Tamura, T. Shiro, M. Ogasawara, A. Toyao, and H. Ishibashi, J. Org. Chem., 2005, 70, 4569; CrossRef d) M. Witkowska, Immun. Therap. Exper., 1972, 20, 787; e) A. Pelczarska, Arch. Immun. Therap. Exper., 1967, 15, 271; f) D. L. J. Clive, D. M. Coltart, and Y. Zhou, J. Org. Chem., 1999, 64, 1447; CrossRef g) T. Janecki, E. Błaszczyk, K. Studzian, M. Rózalski, U. Krajewska, and A. Janecka, J. Med. Chem., 2002, 45, 1142; CrossRef h) J. Lee, S. Wang, G. W. A. Milne, R. Sharma, N. E. Lewin, P. M. Blumberg, and V. E. Marquez, J. Med. Chem., 1996, 39, 29. CrossRef
7. P. Singh, A. Mittal, P. Kaur, and S. Kumar, Tetrahedron, 2006, 62, 1063. CrossRef
8. G. Pitacco, A. Sessanta, O. Santi, and E. Valentin, Tetrahedron: Asymmetry, 2000, 11, 3263. CrossRef
9. R. Chênevert, D. Duguay, F. Touraille, and D. Caron, Tetrahedron: Asymmetry, 2004, 15, 863. CrossRef
10. a) K. Makino, K. Shintani, T. Yamatake, O. Hara, K. Hatano, and Y. Hamada, Tetrahedron, 2002, 58, 9737; CrossRef b) H. Masaki, T. Mizozoe, T. Esumi, Y. Iwabuchi, and S. Hatakeyama, Tetrahedron Lett., 2000, 41, 4801; CrossRef c) J. L. Cohen and A. R. Chamberlin, J. Org. Chem., 2007, 72, 9240. CrossRef
11. a) T. J. Donohoe, C. A Stevenson, M. Helliwell, R. Irshad, and T. Ladduwahetty, Tetrahedron: Asymmetry, 1999, 10, 1315; CrossRef b) D.-P. Jang, J.-W. Chang, and B.-J. Uang, Org. Lett., 2001, 3, 983; c) M-.Y. Chen and J.-M. Fang, J. Org. Chem., 1992, 57, 2937.
12. a) L. C. Dias, I. B. D. de Castro, L. J. Steil, and T. Augusto, Tetrahedron Lett., 2006, 47, 213; CrossRef b) J.-M. Garnier, S. Robin, R. Guillot, and G. Rousseau, Tetrahedron: Asymmetry, 2007, 18, 1434. CrossRef
13. a) J-H. Kang, M. A. Siddiqui, D. M. Sigano, K. Krajewski, N. E. Lewin, Y. Pu, P. M. Blumberg, J. Lee, and V. E. Marquez, Org. Lett., 2004, 6, 2413; CrossRef b) A. Armstrong, C. Ashraff, H. Chung, and L. Murtagh, Tetrahedron, 2009, 65, 4490. CrossRef
14. S. Rana and A. Natarajan, Org. Biomol. Chem., 2013, 11, 244. CrossRef
15. a) A. Paju, T. Kanger, T. Pehk, and M. Lopp, Tetrahedron Lett., 2000, 41, 6883; CrossRef b) A. Paju, T. Kanger, T. Pehk, R. Lindmaa, A.-M. Müürisepp, and M. Lopp, Tetrahedron: Asymmetry, 2003, 14, 1565; CrossRef c) A. Paju, M. Laos, A. Jõgi, M. Päri, R. Jäälaid, T. Pehk, T. Kanger, and M. Lopp, Tetrahedron Lett., 2006, 47, 4491. CrossRef
16. Y. Cao, R. M. Hanson, J. M. Klunder, S. Y. Ko, H. Masamune, and K. B. Sharpless, J. Am. Chem. Soc., 1987, 109, 5765. CrossRef
17. a) A. Paju, T. Kanger, T. Pehk, A.-M. Müürisepp, and M. Lopp, Tetrahedron: Asymmetry, 2002, 13, 2439; CrossRef b) A. Paju, T. Kanger, O. Niitsoo, T. Pehk, A.-M. Müürisepp, and M. Lopp. Tetrahedron: Asymmetry, 2003, 14, 2393. CrossRef
18. A. Jõgi, M. Ilves, A. Paju, T. Pehk, T. Kailas, A.-M. Müürisepp, and M. Lopp, Tetrahedron: Asymmetry, 2008, 19, 628. CrossRef
19. I. Reile, A. Paju, A.-M. Müürisepp, T. Pehk, and M. Lopp, Tetrahedron, 2011, 67, 5942. CrossRef
20. E. M. Tanner and A. Miao, Tetrahedron Lett., 1994, 35, 4073. CrossRef
21. a) A. Paju, T. Kanger, T. Pehk, M. Eek, and M. Lopp, Tetrahedron, 2004, 60, 9081; CrossRef b) M. Lopp, A. Paju, M. Eek, M. Laos, T. Pehk, and R. Jäälaid, 2007, WO2007137593 A1 20071206.
22. H. Lebel and O. Leogane, Org. Lett., 2005, 7, 4107. CrossRef
23. A. Kamal, A. A. Shaik, M. Sandbhor, M. S. Malik, and S. Azeeza, Tetrahedron: Asymmetry, 2006, 17, 2876. CrossRef
24. M. B. Bertrand and J. P. Wolfe, Tetrahedron, 2005, 61, 6447. CrossRef
25. I. Reile, A. Paju, M. Eek, T. Pehk, and M. Lopp, Synlett, 2008, 347. CrossRef
26. O. L. Chapman, K. C. Mattes, R. S. Sheridan, and J. A. Klun, J. Am. Chem. Soc., 1978, 100, 4878. CrossRef
27. U. Frei and R. Kirchmayr, 1988, EP0278914 A2 19880817.
28. T. Hyodo, Y. Kiotsuka, and Y. Kobayashi, Org. Lett., 2009, 11, 1103. CrossRef