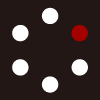
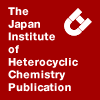
HETEROCYCLES
An International Journal for Reviews and Communications in Heterocyclic ChemistryWeb Edition ISSN: 1881-0942
Published online by The Japan Institute of Heterocyclic Chemistry
e-Journal
Full Text HTML
Received, 23rd May, 2013, Accepted, 25th July, 2013, Published online, 2nd August, 2013.
DOI: 10.3987/COM-13-S(S)17
■ Synthetic Approaches to 3,3’-Biindolyl and 3,3’-Biindazolyl Derivatives
Azadeh Nakhai and Jan Bergman*
Department of Biosciences and Nutrition, Unit for Organic Chemistry, Karolinska Institute, Novum Research Park, SE-141 57 Huddinge, Sweden
Abstract
In this paper new syntheses of 3,3’-biindolyl and 3,3’-biindazolyl derivatives are described. Formation of 3,3’-biindolyl derivatives by oxidative coupling of N-acetylindole with TeCl4 gave a good yield, while attempt to use the same reaction conditions for synthesis of 3,3’-biindazolyl derivatives failed. However, conversion of 3-haloindazole derivatives to its trimethylstannane derivative, followed by palladium-catalyzed Stille cross-coupling reaction, resulted in formation of 3,3’-biindazolyl derivatives.INTRODUCTION
Many naturally existing compounds include aromatic biheterocycles1 as sub-units (Figure 1).
The tetra-pyridine derivative nemertelline 1 is illustrative. This molecule was originally isolated from the marine hoplonemertine worm.2 This compound can be used as an antifouling agent for boats and other marine equipments.3 Another interesting biheterocycle is the classical molecule indigo 2, which is naturally obtained from plants of the genus Indigofera. It has a distinctive blue color and it is primarily used as a dye for cotton fabrics and blue jeans. Furthermore, indolocarbazole derivatives (e.g., staurosporine 3)4 constitute a class of compounds with broad biological activities.5 These 2,2’-biindole derivatives have shown potential in cancer treatment. Interest in these compounds has led to several attempts to produce them synthetically by diverse routes.
One of the most common uses of biheterocycles is as chelating ligands in organometallic chemistry. These biheterocycles have also been used as components in many large supermolecular species such as dendrimers, rotaxanes and catenanes. Although these types of compounds have proven useful in many different fields, detailed knowledge of many other biheterocycle derivatives are limited. This paper describes synthetic approaches to 3,3’-biindolyl and 3,3’-biindazolyl derivatives.
RESULTS AND DISCUSSION
Coupling (spontaneous or induced) via organotellurium compounds are known6 in the literature. Furthermore, Clive7 as reported facile couplings of benzyl halides (to bibenzyls) and allyl halides upon treatment with Te2-. Another interesting oxidative coupling induced by Pd(OAc)2 on indoles and N-acylindoles have earlier been reported.8 For example coupling of N,N’-carbonylindole yielded compound 4 in a reasonable yield. Similar results have been obtained by Itahara9 using related systems.
The 3,3’-biindolyl 5a was first prepared by Gabriel et al. as early as in 192310 and since then, several approaches for the preparation of its derivatives have been reported.1,11-15 Our initial attempts in this project was to investigate the possibility of synthesizing 3,3’-biindolyl derivatives 5 and in this context we contemplated a methodology based on oxidative coupling reaction of indole derivatives with TeCl4. This general synthetic approach for the preparation of 3,3’-biindolyl 5 is summarized in Scheme 1.
The interaction of TeCl4 with N-acetylindole 7 readily afforded N,N’-diacetyl-3,3’-biindolyl 5b in a good yield. Mild hydrolysis of 5b delivered the well-known12 and readily prepared compound 3,3’-biindolyl 5a. Considering the relatively high price of TeCl4 this procedure is suitable only for small scale preparations. N-Benzensulfonylindole did not similarly give the known16 compound 5c. It is not clear if 5b was formed by a direct coupling or via an intermediate organotellurium compound such as di-N-acetyl-indolyl tellurium dichloride 8a. Only a few indole derivatives, (e.g., bis[N-(benzenesulfonyl)-2-indolyl] telluride17 and bis(N-tosyl-2-indolyl) ditelluride18) containing tellurium have been published and repeated attempts now made to prepare 8a or 8b failed. It might be added that di(3-indolyl) ditelluride has earlier been prepared (unpublished results) in this laboratory in a low yield by treating indole with TeCl4 in pyridine followed by a reductive work-up.
A logical extension of the presented results was to introduce N-acetylpyrrole, rather than N-acetylindole, as a reactant. Preliminary experiments using this reactant resulted in formation of N-acetylpolypyrroles 9. We can therefore assume that polypyrrole derivatives 9 can be prepared by this procedure. Polypyrrole itself has been known since 1915.19
To explore the scope of the methodology, we also introduced this method on an azaindole ring system, namely indazole. Despite the fact that indazole is the 2-azabioisostere of indole, repeated attempts to synthesis of 3,3’-biindazolyl derivatives 10 via oxidative coupling with TeCl4 failed. 3,3’-Biindazolyl 10a was first prepared by Hill et al. as early as in 1971.20 They reported two different routes for synthesis of this compound; dehydrogenation of 3,3-bi-tetrahydroindazole, made in two steps from cyclohexanone, and isomerization and cyclization of N,N’-dinitroso-2,2’-diacetamidobibenzyl. Unfortunately, both these approaches failed in our hands. Much later, Servi et al. claimed that reaction between the bisulfite adduct of benzaldehyde and phenylhydrazine, delivers 3,3’-biindazolyl 10a in a high yield (Scheme 2).21 However, since the spectral data (NMR and IR) and the melting point reported by Servi were in strong disagreement with the data reported by Hill, we repeated this reaction and found that the product was in fact the non-cyclic compound 11. This known molecule22-24 could also be made from glyoxal 12 and phenylhydrazine without use of sodium bisulfite.
The Ullmann reaction also failed, when 3-iodoindazoles (N-substituted or not) were used with copper in DMF. Recently, isolation of protected indazolylboronic esters from haloindazoles and bis(pinacolato)diboron have been reported.25 However, the only position that was not claimed was the 3-position of the indazole. Attempts to introduce boronic esters to 3-halo-indazole derivatives using the same conditions were unsuccessful. In contrast, conversion of 3-haloindazole derivatives to its trimethylstannane derivative, followed by palladium-catalyzed Stille cross-coupling reaction has been reported in a German patent.26 Although tin compounds are not convenient to use because of high toxicity, they have become an asset to indazole chemistry. In the absence of other efficient synthetic routes for the synthesis of the 3,3-biindazolyl 10a, we chose to prepare this compound via 3-(trimethylstannyl)indazole derivatives. The synthetic approach is summarized in Scheme 3.
Thus, compound 1327 was treated with sodium tert-butoxide and 2-(trimethylsilanyl)-ethoxymethyl chloride (SEM-Cl) in THF to afford SEM protected 3-iodoindazole 14.25 Treatment of this molecule with hexamethylditin and a suitable palladium catalyst, delivered compound 15. Further, using standard conditions for palladium catalyzed Stille coupling reaction between compound 15 and 14, gave the desired product 10b in accepyable yield. The SEM protected groups could easily be removed using standard basic conditions, TBAF in THF, which resulted in compound 10a in a acceptable yield. It has recently been reported that when 3-iodoindazoles and methyl 2-(acetylamino)acrylate were used in palladium-catalyzed Heck cross-coupling reactions the biindazolyl derivatives (of type 10b) were isolated as by-products in low yields.28 However, since the color of the product and the melting point reported by Crestey et al. were in disagreement with our data, our product was therefore subjected to an X-ray analysis which gave conclusive evidence for structure 10b. A Chem3D representation of the molecule is shown in Figure 5.
In conclusion, the work described here presents synthesis of 3,3´-biindolyl derivatives via oxidative coupling induced by TeCl4. However, attempts to achieving 3,3´-biindazolyl derivatives using TeCl4 failed. In addition, the 3,3´-biindazolyl could be isolated using Stille coupling reaction and the structure of 1,1´-bis[2-(trimethylsilanyl)ethoxymethyl]-[3,3´]biindazolyl was confirmed by X-ray analysis.
EXPERIMENTAL
General experimental procedures
NMR Data were recorded on a Bruker DPX 300 instrument operating at 300.1 MHz for 1H and 75.5 MHz for 13C, respectively, using the residual solvent signal as reference. Assignments are based on standard 1H, ATP, 13C high power decoupling (HPDEC) and 1D NOE-DIFF experiments. IR spectra were acquired on a Thermo Nicolet Avatar 330 FT-IR instrument. Chromatographic separations were performed on silica gel 60 (40-63 µm). HRMS-determintions (FAB) were recorded by Einar Nilsson, University of Lund, Sweden. Elemental analyses were performed by LSM Lab, Uppsala, Sweden. Chemicals and solvents were obtained from commercial sources and used as received with the exception of THF, which was distilled from sodium and benzophenone and 1,4-dioxane and Et2O, which were stored over sodium.
N-Acetylindole (7).29,30 Indole (35.1 g, 0.3 mol) in acetic anhydride (40 mL) and triethylamine (10 mL) was heated to reflux temperature for 8 h, whereupon the reaction mixture was concentrated and treated with water and Et2O. The combined organic solvents were washed with a saturated solution of sodium hydrogencarbonate, dried with Na2SO4, filtered and evaporated. The crude product was purified by distillation at 153-154 ºC/15 torr (156 ºC/16 torr)30 to yield 7 (40.9 g, 86%) as an oil. IR (neat) 1698, 1450, 1382, 1366, 1321, 1207, 932, 746 cm-1; 1H NMR (DMSO-d6) δ 8.34 (d, J=7.8 Hz, 1H), 7.84 (d, J=3.5 Hz, 1H), 7.61 (d, J=7.8 Hz, 1H), 7.38-7.19 (m, 2H), 6.73 (d, J=3.5 Hz, 1H), 2.65 (s, 3H); 13C NMR (DMSO-d6) δ 169.6 (s), 134.9 (s), 130.3 (s), 127.2 (d), 124.5 (d), 123.4 (d), 120.9 (d), 115.9 (d), 108.2 (d), 23.8 (q).
N,N’-Diacetyl-3,3’-biindolyl (5b) To a solution of N-acetylindole (2.0 g, 12.6 mmol) and MeCN (25 mL) was TeCl4 (6.8 g, 25.1 mmol) added and the mixture heated to reflux for 2 h. The dark suspension was filtered thought a pad of celite twice and the remaining dark solution was left at rt overnight leading to precipitation of the product. This material was crystallized from MeCN to afford 2.8 g (70%) light green crystalline material, mp 225 °C; IR (neat) 1703, 1530, 1444, 1372, 1327, 1223, 944, 733 cm-1; 1H NMR (DMSO-d6) δ 8.47 (d, J=7.4 Hz, 2H), 8.17 (s, 2H), 7.94 (d, J=7.4 Hz, 2H), 7.50-7.34 (m, 4H), 2.78 (s, 6H); 13C NMR (DMSO-d6) δ 169.7 (s), 135.3 (s), 128.8 (s), 125.3 (d), 124.6 (d), 123.8 (d), 120.3 (d), 116.1 (d), 113.4 (s), 24.0 (q). Anal. Calcd for C20H16N2O2: C, 75.93; H, 5.10; N, 8.86. Found: C, 75.89; H, 5.02, N, 8.76.
3,3’-Biindolyl (5a) mp 280-281 °C (lit. 284-286 °C)11; IR (neat) 3390, 1454, 1409, 1334, 1238, 1109, 1012, 736 cm-1; 1H NMR (DMSO-d6) δ 11.15 (br. s, 1H), 7.78 (d, J=7.8 Hz, 1H), 7.63 (d, J=2.4 Hz, 1H), 7.45 (d, J=8.0 Hz, 1H), 7.19-7.11 (m, 1H), 7.10-7.02 (m, 1H); 13C NMR (DMSO-d6) δ 136.4 (s), 126.0 (s), 121.8 (d), 121.2 (d), 119.6 (d), 118.8 (d), 111.5 (d), 109.7 (s).
3-Iodoindazole (13)27 This compound was prepared from indazole (5.0 g, 42.3 mmol) according to ref.27 The product was obtained as a yellow solid (8.7 g, 84%), mp 139-140 °C (lit. 141 °C)27; IR (neat) 3152, 3088, 2970, 2932, 1620, 1472, 1238, 1012 cm-1; 1H NMR (DMSO-d6) δ 13.50 (br. s, 1H), 7.58-7.52 (m, 1H), 7.46-7.39 (m, 2H), 7.23-7.16 (m, 1H); 13C NMR (DMSO-d6) δ 140.4 (s), 127.2 (d), 126.8 (s), 121.2 (d), 120.4 (d), 110.5 (d), 93.4 (s).
1-((2-Trimethylsilyl)ethoxy)methyl-3-iodo-1H-indazole (14)27 To a solution of 3-iodoindazole (2 g, 8.2 mmol) in anhydrous THF (25 mL) at 0 °C under Ar-gas was NaOtBu (0.95 g, 9.8 mmol) added. After stirring at 0 °C for 30 min, SEM-Cl (1.74 mL, 9.8 mmol) was added slowly. After complete addition, the mixture was stirred at 0 °C for 1 h and thereafter an additional hour at room temperature. The mixture was diluted with EtOAc and quenched with water. The layers were separated, and the organic layer was washed with brine, dried over MgSO4, filtered, and concentrated. The residue was purified using column chromatography (gradient: 0-15% hexane/EtOAc) to afford 14 (1.9 g, 61%) as a pale yellow oil. IR (neat) 2951, 1614, 1459, 1315, 1247, 1076, 832, 742 cm-1; 1H NMR (DMSO-d6) δ 7.75 (d, J=8.5 Hz, 1H), 7.57-7.42 (m, 2H), 7.27 (t, J=7.5 Hz, 1H), 5.75 (s, 2H), 3.57-3.45 (m, 2H), 0.84-0.73 (m, 2H), -0.12 (s, 9H); 13C NMR (DMSO-d6) δ 140.2 (s), 128.2 (s), 127.8 (d), 122.1 (d), 120.9 (d), 110.3 (d), 94.7 (s), 76.8 (t), 65.7 (t), 17.1 (t), -1.4 (q).
1-((2-Trimethylsilyl)ethoxy)methyl-3-(trimethylstannyl)-1H-indazole (15) A mixture of 14 (0.8 g, 2.9 mmol), hexamethyldistannane (1.05 g, 3.2 mmol) and (PPh3)2PdCl2 (41 mg, 0.06 mmol) in dry toluene (10 mL) was heated to reflux for 45 min under argon gas. The suspension was filtered through a layer of celite and removal of the solvent left a crude product, which was purified by column chromatography (hexane/EtOAc) to afford 15 (0.83 g, 70%) as an unstable solid. IR (neat) 2952, 1460, 1304, 1248, 1167, 1073, 833, 741 cm-1; 1H NMR (CDCl3) δ 7.75 (d, J=8.2 Hz, 1H), 7.60 (d, J=8.2 Hz, 1H), 7.45-7.36 (m, 1H), 7.22-7.13 (m, 1H), 5.80 (s, 2H), 3.63-3.53 (m, 2H), 0.95-0.85 (m, 2H), 0.48 (s, with small side signals, 9H), -0.06 (s, 9H); 13C NMR (CDCl3) δ 148.9 (s), 139.9 (s), 132.8 (s), 126.3 (d), 122.1 (d), 120.7 (d), 109.7 (d), 77.3 (t), 66.3 (t), 17.8 (t), -1.4 (q), -8.8 (q).
1,1´-Bis[2-(trimethylsilanyl)ethoxymethyl][3,3´]biindazolyl (10b) A mixture of 15 (0.83 g, 2.03 mmol), 14 (0.61 g, 2.23 mmol), (PPh3)4Pd (120 mg, 0.1 mmol) and CuI (40 mg, 0.2 mmol) in anhydrous THF (10 mL) was heated to reflux overnight under argon gas. The dark suspension was filtered thought a pad of celite and removal of the solvent left a crude product, which was purified by column chromatography (gradient: 0-15% hexane/EtOAc) to afford 10b (0.53 g, 53%) as a white solid, mp 90.5-92.2 °C (lit. 82-84 °C)28; IR (neat) 2952, 1612, 1463, 1297, 1247, 1073, 831, 742 cm-1; 1H NMR (DMSO-d6) δ 8.47 (d, J=8.1 Hz, 1H), 7.83 (d, J=8.1 Hz, 1H), 7.58-7.50 (m, 1H), 7.37-7.29 (m, 1H), 5.91 (s, 2H), 3.66 (t, J=8.0 Hz, 2H), 0.86 (t, J=8.0 Hz, 2H), -0.13 (s, 9H); 13C NMR (DMSO-d6) δ 140.6 (s), 138.9 (s), 127.2 (d), 122.4 (d), 121.9 (d), 121.9 (s), 110.2 (d), 76.8 (t), 65.8 (t), 17.1 (t), -1.5 (q).
3,3’-Biindazolyl (10a)20 To a mixture of 10b (0.2 g, 0.4 mmol) and ethane-1,2-diamine (0.27 mL, 4.0 mmol) was TBAF (1M in THF, 8.0 mL, 8.0 mmol) added and the mixture was refluxed overnight. The reaction mixture was diluted with EtOAc (30 mL) and washed with H2O (2 x 40 mL) and brine (30 mL). The organic layer was dried (MgSO4), filtered and concentrated in vacuo. The residue was purified using column chromatography (hexane/EtOAc) to afford 10a (61 mg, 65%) as a yellow solid mp 330-332 °C (lit. 328-330 °C).20 1H NMR (DMSO-d6) δ 13.27 (br. s, 1H), 8.45 (d, J=8.1 Hz, 1H), 7.61 (d, J=8.4 Hz, 1H), 7.48-7.38 (m, 1H), 7.28-7.20 (m, 1H); 13C NMR (DMSO-d6) δ 140.8 (s), 139.5 (s), 126.4 (d), 122.3 (d), 120.9 (d), 120.6 (s), 110.2 (d).
References
1. P. J. Steel, Adv. Het. Chem., 1997, 67, 1.
2. W. R. Kem, K. N. Scott, and J. H. Duncan, Experientia, 1976, 32, 684. CrossRef
3. US Pat. 0235901 (2004).
4. S. Omura, Y. Iwai, A. Hirano, A. Nakagawa, J. Awaya, H. Tsuchya, Y. Takahashi, and R. Masuma, J. Antibiot., 1977, 30, 275. CrossRef
5. T. Janosik, N. Wahlstroem, and J. Bergman, Tetrahedron, 2008, 64, 9159. CrossRef
6. J. Bergman, Tetrahedron, 1972, 28, 3323. CrossRef
7. D. L. J. Clive, P. C. Anderson, N. Moss, and A. Singh, J. Org. Chem., 1982, 47, 1641. CrossRef
8. J. Bergman and N. Eklund, Tetrahedron, 1980, 36, 1439. CrossRef
9. T. Itahara, Heterocycles, 1986, 24, 2557. CrossRef
10. S. Gabriel, W. Gerhard, and R. Wolter, Ber. Dtsch. Chem. Ges., 1923, 56, 1024. CrossRef
11. W. R. Carpenter, M. S. Grant, and H. R. Snyder, J. Am. Chem. Soc., 1960, 82, 2739. CrossRef
12. J. Bergman, Acta Chem. Scand., 1971, 25, 1277. CrossRef
13. K. Fujino, E. Yanase, Y. Shinoda, and S.-i. Nakatsuka, Biosci. Biotechnol. Biochem., 2004, 68, 764. CrossRef
14. Y. Li, W.-H. Wang, S.-D. Yang, B.-J. Li, C. Feng, and Z.-J. Shi, Chem. Commun., 2010, 46, 4553. CrossRef
15. C. Ramesh, V. Kavala, C.-W. Kuo, B. R. Raju, and C.-F. Yao, Eur. J. Org. Chem., 2010, 3796. CrossRef
16. J. Bergman and N. Eklund, Chem. Scr., 1982, 19, 193.
17. L. Engman, D. Stern, M. Pelcman, and C. M. Andersson, J. Org. Chem. , 1994, 59, 1973. CrossRef
18. L. Engman and M. P. Cava, Organometallics, 1982, 1, 470. CrossRef
19. P. Baker, D. Matthews, and A. Hope, Aust. J. Chem., 1994, 47, 1. CrossRef
20. J. H. M. Hill, D. M. Berkowitz, and K. J. Freese, J. Org. Chem., 1971, 36, 1563. CrossRef
21. S. Servi and Z. R. Akgun, Synth. Commun., 2002, 32, 3399. CrossRef
22. K. Kindler, Ber. Dtsch. Chem. Ges., 1921, 54B, 647.
23. A. S. Roy, H. M. Tuononen, S. P. Rath, and P. Ghosh, Inorg. Chem., 2007, 46, 5942. CrossRef
24. Y.-H. Liu, G. Li, and L.-M. Yang, Tetrahedron Lett., 2009, 50, 343. CrossRef
25. F. Crestey, E. Lohou, S. Stiebing, V. Collot, and S. Rault, Synlett, 2009, 615. CrossRef
26. DE Pat. 1964232 (1998).
27. V. Collot, P. Dallemagne, P. R. Bovy, and S. Rault, Tetrahedron, 1999, 55, 6917. CrossRef
28. F. Crestey, V. Collot, S. Stiebing, and S. Rault, Synthesis, 2006, 3506. CrossRef
29. G. W. Gribble, L. W. Reilly, Jr., and J. L. Johnson, Org. Prep. Proc. Int., 1977, 9, 271. CrossRef
30. K. Nickisch, W. Klose, and F. Bohlmann, Chem. Ber., 1980, 113, 2036. CrossRef