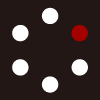
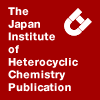
HETEROCYCLES
An International Journal for Reviews and Communications in Heterocyclic ChemistryWeb Edition ISSN: 1881-0942
Published online by The Japan Institute of Heterocyclic Chemistry
e-Journal
Full Text HTML
Received, 29th July, 2013, Accepted, 9th September, 2013, Published online, 19th September, 2013.
DOI: 10.3987/COM-13-S(S)100
■ Synthesis of Functionalized 1-Azabicyclo[3.1.0]hexanes: Studies towards Ficellomycin and Its Analogs
Gang Chen, Zhi He, and Andrei K. Yudin*
Davenport Research Laboratories, Department of Chemistry, University of Toronto, 80 St. George Street., Toronto, Ontario, M5S 3H6, Canada
Abstract
The molecule of antibiotic agent ficellomycin contains an intriguing array of functional groups assembled around a bicyclic aziridine core. To address the challenges of constructing ficellomycin derivatives, we have developed cycloamination methodology for the stereocontrolled transformation of unprotected aziridine-containing olefins. The present paper discusses application of this process towards synthesis of ficellomycin.INTRODUCTION
Ficellomycin was isolated from Streptomyces ficellus in 1976.1 Initial structural assignment (structure 1, Figure 1) was established by NMR and chemical degradation studies.2 The dipeptide molecule was found to possess a rare azabicyclo[3.1.0]hexane core, a structural motif present in only a few natural products.3 The relative stereochemistry of the ficellomycin core was later revised to 2 (Figure 1).4 The cis-relationship between the guanidine substituent and the aziridine methylene group has been suggested in this structural variant. In addition to this apparent ambiguity, the stereochemistry beside the acid group has not been rigorously elucidated. The absolute stereochemistry of the ficellomycin core is not clear either.3b To date, there has been no report on a total synthesis of this naturally occurring antibiotic.
Ficellomycin displays in vivo antibiotic activity against S. aureus and P. oxalicum in mice. Biochemical studies indicate that ficellomycin selectively impairs semiconservative DNA replication in E. coli.5 Although the exact mechanism of action of ficellomycin is presently unknown, covalent modification of DNA has been established as the mode of action for other aziridine-containing natural products such as azinomycins, mitomycins, and FR900482. The azinomycin B/DNA interaction is based on covalent modification of the 3’-CGG-5’ sequence,6 whereas mitomycin C and FR900482 make covalent links with the 5’-CG-3’ sequence.7 The specificity of these interactions can be substantially improved by introducing skeletal modifications. For instance, FK317, a semisynthetic derivative of FR 900482, has shown reduced side effects without diminished potency. A fairly high IC50 value of 0.5 mM for ficellomycin suggests that synthetic modifications may result in more selective compounds and may help elucidate the mechanism of action for this antibiotic. A previously reported synthetic approach to the ficellomycin core by Shipman and co-workers was based on an intramolecular 1,4-addition of an unprotected aziridine to a β-amino α,β-unsaturated ester.8 A 1,4-addition-elimination methodology was earlier employed in an elegant total synthesis of azinomycin by Coleman and co-workers.9 As part of a program directed towards complex amines, we have been interested in stereocontrolled construction of ficellomycin derivatives.10,11
RESULTS AND DISCUSSION
Previously, stereospecific ring-opening reactions of bicyclic aziridines were found to afford piperidine and pyrrolidine heterocycles.11 We were hopeful that this methodology could be further developed to address the ficellomycin core synthesis by one of two strategies (Figure 2). If the aziridine moiety on the bromo- substituted cycloamination product 3 is opened with a nitrogen nucleophile, the resulting bromoamine intermediate can undergo tandem cyclization to afford the ficellomycin core (Route A). If the aziridine ring-opening reaction proceeds in an SN2 fashion, the stereocenter beside the acid group can be controlled by using trans- or cis-aziridine starting materials. The diastereomeric products of cycloamination can lead to either of the two proposed ficellomycin core structures. On the other hand, direct cycloamination of the aziridine-containing olefin 4 can afford the ficellomycin core if the cyclization is followed by a nucleophilic displacement of bromine (Route B). In order to simplify the synthesis and structural analysis, a protected alcohol was used in place of the guanidine group.12 The acid functionality was kept in the ester or protected alcohol form.
At the outset, ethyl acetate was treated with lithium diisopropylamide and the resulting lithium enolate was reacted with acrolein to afford the aldol addition product (Scheme 1).13 After protection of the alcohol functionality, ester 5 was reduced to the aldehyde and in situ reacted with the commercially available Horner-Wadsworth-Emmons reagent to afford diene 6.14 Epoxidation of 6 with m-CPBA gave the undesired terminal epoxide. Therefore, 6 was reduced to the allylic alcohol 7 in which the terminal hydroxyl functionality allowed for a regioselective epoxidation. The epoxide was regioselectively installed into 7 to afford intermediate 8. The epoxy alcohol 8 was formed as a mixture of two diastereomers which were then converted into the acid form and esterfied in a one-pot process to give epoxy methyl ester 9 in 71% yield. The epoxide was converted to the desired aziridine using a Staudinger reaction.15 The two-step transformation led to a diasteromeric mixture of 10a and 10b in 76% yield based on recovered starting material.
In order to arrive at the intermediate 4 (Figure 2), the terminal hydroxyl group of 7 was protected as a p-methoxybenzyl ether and the silyl ether was removed to generate 11. The terminal olefin was stereoselectively epoxidized using Sharpless kinetic resolution.16 A single enantiomer of 12 was obtained. After protection of the secondary alcohol and a two-step aziridination, the desired aziridine-containing olefin 14 was prepared as a single enantiomer (Scheme 2). Overall, the desired aziridine-containing olefin starting materials 10 (Scheme 1) and 14 (Scheme 2) were prepared via common intermediate 7.
To construct the ficellomycin core according to route A, the aziridine-containing olefins 10a and 10b were subjected to cycloamination in dichloromethane. We have found that the starting material was converted to the N-Br species 15 in the first few minutes of the reaction (Figure 3). The intermediate 15 is relatively stable and can be purified by column chromatography. We were delighted to isolate and characterize the desired diastereomer 16 as the major product of cyclization of 15. The stereochemistry of compound 16 was confirmed by analysis of the ROESY spectra. The other three diastereomeric products were isolated as an inseparable mixture epi-16 (Scheme 3). About 10% of unreacted starting material 10 was recovered after reductive workup. Interestingly, this material was identified as pure 10b, which appears to be the less reactive diastereomer. The selectivity of this reaction can be explained by considering relative energies of the four diastereomeric transition states A-D. The transition state A, in which all substituents occupy equatorial positions of the pseudo-chair, is the lowest in energy. The isolated yield of 16 is acceptable, considering that only one half of the starting material with correct stereochemistry can be converted to the desired product.
The bicyclic aziridine 16 was subjected to an acid-catalyzed aziridine ring-opening. Under a variety of conditions, the reaction was complicated by decomposition of the aziridine, direct substitution of bromide, and other side reactions. Treatment of 16 with TsOH afforded 17 via clean SN2 aziridine ring-opening with the sulfonate anion followed by an intramolecular substitution of bromide. This regioselectivity was not observed in the previous examples of bicyclic aziridine ring openings and constitutes an attractive route to sterochemically complex piperidines. Efforts to optimize the reaction in order to selectively form the desired five-membered ring product along the SN2 pathway are ongoing.
The other enantiomerically pure aziridine-containing olefin 14 was converted to the corresponding azabicycle in 57% yield following route B (Scheme 4). The two epimers were found to be separable by column chromatography. Analysis of the ROESY spectra of product 18 was used in order to elucidate the relative stereochemistry. Subsequently, nucleophilic displacement of bromide afforded the compound 19. In this approach, the choice of 2,3-anti or 2,3-syn substituted aziridine starting material determines which of the two proposed core structures will be constructed. Due to the anti mode of attack during cyclization, the stereocenter beside the acid group can be controlled by using trans- or cis-olefin in the linear starting material. The compound 19 can be converted to the final structure that will complete the total synthesis through a series of transformations—peptide coupling to a valine residue upon reduction of azide to amine, adjustment of the oxidation state of terminal carbon to acid, and installation of the guanidine group.
In conclusion, our work demonstrates that oxidative cycloamination of aziridine-containing olefins can be applied to the synthesis of the ficellomycin core. Compared to previous studies, the relative stereochemistry of all four stereocenters around the core structure can now be controlled via stereospecific cycloamination methodology. By using different readily available diastereomeric starting materials and nucleophiles, synthesis of ficellomycin along with its analogs and their ring-opened derivatives should be facilitated.
EXPERIMENTAL
General: Anhydrous solvents such as ether, toluene, acetonitrile, and dichloromethane were prepared using the method described by Grubbs.17 Tetrahydrofuran was distilled from sodium-benzophenone ketyl under nitrogen. Unless otherwise stated, all reactions were performed under nitrogen or argon atmosphere using flame-dried glassware. Commercially available reagents were purchased and used without any further purification.
Nuclear magnetic resonance spectra: 1H and 13C NMR spectra were recorded on Varian Mercury-400 spectrometer. 1H NMR spectra were referenced to residual CHCl3 (δ 7.26), 13C NMR spectra were referenced to CDCl3 (δ 77.23), Peak multiplicities are designated by the following abbreviations: s, singlet; bs, broad singlet; d, doublet; t, triplet; q, quartet; m, multiplet; dd, doublet of doublets; dt, doublet of triplets; br, broad; and J, coupling constant in Hz.
Chromatography: Analytical thin layer chromatography (TLC) was performed on Macherey Nagel pre-coated glass-backed TLC plates (SIL G/UV254, 0.25 mm) and visualized by UV lamp (254 nm), potassium permanganate stain, or iodine stain. Solvent ratios for Rf values are reported as v/v. Column chromatography was carried out using 230-400 mesh silica gel.
Preparation of ethyl 3-(tert-butyldiphenylsilyloxy)pent-4-enoate (5): To a flask containing ethyl 3-hydroxypent-4-enoate18 (4.0 g, 28 mmol) in MeCN (25 mL), was added imidazole (3.6 g, 53 mmol) to form a clear yellow solution. To the reaction mixture, tert-butyldiphenylsilyl chloride (10 mL, 38 mmol) was added slowly with ice bath cooling. The mixture was stirred overnight. White precipitate was formed in the course of the reaction. The mixture was filtered and concentrated. The residue was extracted with hexanes and purified on a short silica gel plug to afford 5 (9.1 g, 85%) as a yellow oil. 1H NMR (400 MHz, CDCl3) δ 7.67-7.70 (m, 4H), 7.38-7.43 (m, 6H), 5.80-5.90 (m, 1H), 4.96-5.00 (m, 2H), 4.59-4.62 (m, 1H), 4.03-4.06 (m, 2H), 2.43-2.62 (m, 2H), 1.20 (t, J = 6.8 Hz, 3H), 1.07 (s, 9H); 13C NMR: (100 MHz, CDCl3) δ170.9, 139.6, 136.2, 136.1, 134.0, 129.8, 129.7, 127.7, 127.6, 115.5, 72.0, 60.5, 43.6, 27.1, 19.5, 14.3.
Preparation of (E)-ethyl 5-(tert-butyldiphenylsilyloxy)hepta-2,6-dienoate (6): To a solution of ethyl 3-(tert-butyldiphenylsilyloxy)pent-4-enoate (5, 5.3g, 13.8 mmol) in anhydrous Et2O (50 mL) at -78 oC was added DIBAL-H solution in toluene (1.5 M, 11 mL, 16.5 mmol) dropwise. The reaction mixture was stirred at -78 oC for 1.5 h. To a suspension of NaH (0.55 g, 60% in mineral oil, 13.8 mmol) in anhydrous THF (120 mL) at 0 oC, was added ethyl 2-(diethoxyphosphoryl)acetate (3.0 mL, 15.2 mmol). The resulting solution was added slowly to the above DIBAL-H reaction maintained at -78 oC. The reaction was stirred and allowed to reach room temperature overnight. The reaction was concentrated, extracted with pentane, washed with brine, dried over MgSO4, concentrated again and purified on silica gel to afford 6 (5.2 g, yield 89%). 1H NMR (400 MHz, CDCl3) δ 7.63-7.68 (m, 4H), 7.35-7.42 (m, 6H), 6.80-6.88 (m, 1H), 5.70-5.85 (m, 2H), 4.98-5.06 (m, 2H), 4.28-4.31 (m, 1H), 4.15 (q, J = 7.2, 2H), 2.30-2.32 (m, 2H), 1.26 (t, J = 7.2 Hz, 3H), 1.07 (s, 9H); 13C NMR: (100 MHz, CDCl3) δ 166.5, 144.8, 139.8, 136.1 (2), 135.0, 134.2, 133.9, 130.0, 129.8, 127.8, 127.7, 123.9, 115.4, 73.5, 60.3, 40.8, 27.2, 19.5, 14.4.
Preparation of (E)-5-(tert-butyldiphenylsilyloxy)hepta-2,6-dien-1-ol (7): To a solution of (E)-ethyl 5-(tert-butyldiphenylsilyloxy)hepta-2,6-dienoate (6, 4.0 g, 10 mmol) in DCM (50 mL), was slowly added diisobutylaluminium hydride solution (1.0 M in hexanes, 20 mL) at -78 oC over 30 min. The reaction was stirred overnight and quenched by slow addition of tartaric acid solution (1.0 M in water, 15 mL). The mixture was stirred for 30 min at room temperature until solid has formed on the bottom of flask. The solution was filtered and concentrated. The residue was purified on a short silica gel plug to afford 7 as a colorless oil (3.3 g, yield 90%) 1H NMR (400 MHz, CDCl3) δ 7.63-7.70 (m, 4H), 7.35-7.39 (m, 6H), 5.78-5.82 (m, 1H), 5.12 (m, 2H), 4.98-5.03 (m, 2H), 4.19-4.21 (m, 1H), 4.95-4.99 (m, 2H), 2.18-2.21 (m, 2H), 1.06 (s, 9H); 13C NMR: (100 MHz, CDCl3) δ 140.5, 136.2 (2), 134.6, 134.3, 131.8, 129.9, 129.8, 128.7, 127.7, 127.6 , 114.7, 74.3, 63.9, 40.9, 27.2, 19.6.
Preparation of ((2S*,3S*)-3-(2-(tert-butyldiphenylsilyloxy)but-3-enyl)oxiran-2-yl) methanol (8): To a solution of 7 (2.0 g, 5.5 mmol) and VO(acac)2 (160 mg, 10 mol %) in DCM (80 mL) at 0 oC, was added tert-butyl hydroperoxide solution in decane (1.14 mL, 6.0 mmol). The reaction was allowed to reach room temperature and was stirred overnight. After completion of the reaction, sodium sulfite (5.0 g) was added. The mixture was filtered. The filtrate was concentrated and purified on silica gel to afford 8 as a mixture of two diastereomers (1.9 g, yield 90%). 1H NMR (400 MHz, CDCl3) δ 7.62-7.68 (m, 4H), 7.35-7.38 (m, 6H), 5.58-5.95 (m, 1H), 4.95-5.10 (m, 2H), 4.31-4.42 (m, 1H), 3.25-3.36 (m, 1H), 3.47-3.57 (m, 1H), 2.95-2.98 (m, 1H), 2.74-2.80 (m, 2H), 1.58-1.82 (m, 3H), 1.08 (s, 9H); 13C NMR: (100 MHz, CDCl3) δ (140.4, 140.1), (136.2,136.0), (134.0, 133.9), (130.0, 129.9), (127.8, 127.7), (115.3, 115.0), (72.7, 72.6), (61.8, 61.7), (58.9, 58.7), (52.9, 25.7), (40.2, 39.9), 27.2, (19.6, 19.5).
Preparation of (2R,3S)-methyl 3-(2-(tert-butyldiphenylsilyloxy)but-3-enyl)oxirane-2-carboxylate (9): To a solution of oxalyl chloride (182.7 µL, 2.1 mmol) in anhydrous DCM (6 mL) at -78 oC was added DMSO (297 µL, 4.2 mmol). The reaction was stirred for 5 min, ((2S*,3S*)-3-(2-(tert- butyldiphenylsilyloxy)but-3-enyl)oxiran-2-yl) methanol (8, 680 mg, 1.78 mmol in 6 mL of DCM) was added dropwise at -78 oC followed by addition of triethylamine (1.79 mL, 13 mmol) after 15 min. The reaction was allowed to reach room temperature and water (10 mL) was added. The aqueous layer was extracted. The combined organic layer was washed with brine, dried, and concentrated to yield the crude intermediate aldehyde. To the aldehyde intermediate (500 mg, 1.31 mmol) in t-BuOH (10 mL) were added cyclohexene (689 mg, 8.4 mmol) and aqueous solution of NaH2PO4 (216 mg, 1.8 mmol) and NaClO2 (163 mg, 1.8 mmol) in 5 mL water. The reaction was stirred overnight at room temperature. The reaction was diluted with brine (50 mL), extracted with hexanes and EtOAc (1:1), the aqueous layer was acidified to pH 2, and extracted one more time. The combined organic layer was concentrated, and dissolved in DCM / MeOH (4:1, 10 mL). To the solution was added TMSCHN2 (2.0 M in hexanes, 1.0 mL 2.0 mmol). The reaction was quenched by addition of AcOH (0.5 mL), washed with NaHCO3 solution and concentrated. The residue was purified on silica gel to afford 9 (490 mg, yield 67%) as a mixture of two diastereomers. 1H NMR (400 MHz, CDCl3) δ 7.64-7.69 (m, 4H), 7.36-7.42 (m, 6H), 5.76-5.90 (m, 1H), 4.98-5.10 (m, 2H), 4.17-4.20 (m, 1H), 3.75 (s, 3H), 3.07-3.22 (m, 2H), 1.62-1.81 (m, 2H), 1.07 (s, 9H); 13C NMR: (100 MHz, CDCl3) δ 169.7, (139.7, 139.4), (136.1, 136.0), (134.0, 133.8), (130.0, 129.9), (127.9, 127.7), (115.8, 115.6), (72.4, 72.3), (55.5, 55.3), (53.3, 53.2), 52.6 (2C), (39.9, 39.7), 27.2, (19.5, 19.4).
Preparation of (2S*,3R*)-methyl 3-(2-(tert-butyldiphenylsilyloxy)but-3-enyl)aziridine-2-carboxylate (10): To a solution of (2R*,3S*)-methyl 3-(2-(tert-butyldiphenylsilyloxy)but-3-enyl)oxirane-2- carboxylate (9, 450 mg, 1.1 mmol) in MeOH (15 mL) were added NaN3 (715 mg, 11 mmol) and NH4Cl (594 mg, 11 mmol). The mixture was stirred at room temperature for 60 h. TLC indicated approximate 50% conversion to product. The mixture was filtered and concentrated under reduced pressure. The residue was diluted with brine and extracted with EtOAc. The organic layer was dried over MgSO4 and concentrated. The crude mixture was dissolved in MeCN (15 mL) followed by slow addition of triphenylphosphine (222 mg, 0.8 mmol). The reaction was heated at 90 oC for 1 h until all azido-alcohol was consumed. The reaction was concentrated and purified on a silica gel column to afford 10 (185 mg, yield 41%) and recovered starting material (210 mg, 46%). 1H NMR (400 MHz, CDCl3) δ 7.63-7.70 (m, 4H), 7.35-7.46 (m, 6H), 5.78-5.89 (m, 1H), 4.97-5.07 (m, 2H), 4.38-4.40 (m, 1H), 3.72 (s, 3H), 2.05-2.30 (m, 2H), 1.45-1.78 (m, 2H), 0.95-1.22 (m, 1H), 1.07 (s, 9H); 13C NMR: (100 MHz, CDCl3) δ 173.2, (140.5, 140.0), (136.2, 136.1), 134.3, (129.9, 129.8), (127.8, 127.7), (115.3, 115.0), (73.3, 73.2), 52.6, (41.3, 40.8), (36.4, 36.1), (35.7, 35.4), 27.2 (2), (19.6, 19.5). HR-MS (EI) m/z: calcd. for C24H31NO3Si (M+) 409.2073, obsd 352.1362 (loss of t-butyl group).
Preparation of (E)-7-(4-methoxybenzyloxy)hepta-1,5-dien-3-ol (11): A solution of (E)-5-(tert-butyldiphenylsilyloxy)hepta-2,6-dien-1-ol (7, 18.3 g, 50 mmol) in THF (70 mL) was added to suspension of NaH (3.0 g, 60% in mineral oil, 75 mmol) in THF (125 mL) under nitrogen at 0 oC. The reaction was stirred for 30 min followed by a slow addition of 4-methoxybenzyl chloride (9.4 g, 60 mmol) in THF (50 mL). n-Bu4NI (1.85 g, 5 mmol) was added to the stirred mixture. The reaction was allowed to reach room temperature and stirred for 48 h until all of the starting material 7 was consumed. The reaction was concentrated, diluted with EtOAc (500 mL), and washed with brine (300 mL). The combined organic layers were dried over MgSO4, concentrated and purified on a short silica gel plug to give a yellow oil, which was redissolved in THF (250 mL). Tetrabutylammonium fluoride (TBAF, 1.0 M solution in THF, 60 mL, 60 mmol) was added to the solution dropwise. The reaction mixture was stirred overnight and concentrated. The residue was purified on a silica gel column to afford 11 as colorless oil (11.1 g, yield 90% in two steps) 1H NMR (400 MHz, CDCl3) δ 7.27-7.25 (m, 2H), 6.88-6.86 (m, 2H), 5.92-5.84 (m, 1H), 5.72-5.70 (m, 2H), 5.27-5.23 (m, 1H), 5.14-5.11 (m, 1H), 4.43 (s, 2H), 4.16 (dd, J = 12.5 Hz, J = 7.0 Hz, 1H), 3.97-3.96 (m, 2H), 3.80 (s, 3H), 2.34-2.30 (m, 2H), 1.84 (br, 1H); 13C NMR: (100 MHz, CDCl3) δ 159.4, 140.5, 130.5, 130.4, 129.6, 115.0, 114.0, 72.2, 71.9, 70.5, 55.5, 40.4.
Preparation of (S,E)-5-(4-methoxybenzyloxy)-1-((R)-oxiran-2-yl)pent-3-en-1-ol (12):16 A flask charged with molecular sieves (4Å, powder, 3.5 g) was stirred and heated at 160 oC under vacuum overnight. To the activated molecular sieves, was added DCM (150 mL) and cooled to -20 oC, L-(+)-diisopropyl tartrate (1.18 mL, 5.6 mmol), titanium (IV) isopropoxide (1.10 mL, 3.76 mmol) and tert-butyl hydroperoxide (4.8 mL, 5.5 M solution in decane, 26.4 mmol) were added under nitrogen. The resulting mixture was stirred at -20 oC for 30 min. (E)-7-(4-Methoxybenzyloxy)hepta-1,5-dien-3-ol (11, 10.7 g, 43 mmol) was then added slowly to the mixture with the temperature maintained between -20 and -15 oC. The reaction was moved to a freezer (-20 oC) and stirred for 3 days. The reaction was poured into a mixture of FeSO4/tartaric acid solution (50 mL) in an ice bath. The mixture was stirred for 10 min. The organic layer was separated and aqueous layer was extracted with Et2O (500 mL). The combined organic layer was stirred with aqueous solution of NaOH (0.9g) and NaCl (0.2g) in 3 mL water at 0 oC for 1 h, then washed with water, dried over MgSO4, and concentrated. The residue was purified on a silica gel column to afford the unreacted enantiomer (5.5 g) and 12 (5.1 g, yield 45%) 1H NMR (400 MHz, CDCl3) δ 7.27-7.25 (m, 2H), 6.89-6.86 (m, 2H), 5.76-5.74 (m, 2H), 4.44 (s, 2H), 3.97 (d, J = 4.8 Hz, 2H), 3.81 (m, 1H), 3.80 (s, 3H), 3.02 (m, 1H), 2.79 (m, 1H), 2.74(m, 1H); 2.42-2.32 (m, 2H), 1.96 (s, 1H); 13C NMR: (100 MHz, CDCl3) δ 159.4, 130.6, 130.4, 129.8, 128.9, 114.0, 72.1, 70.4, 68.6, 55.6, 54.3, 44.0, 36.9.
Preparation of (R)-2-((S,E)-1,5-bis(4-methoxybenzyloxy)pent-3-enyl)oxirane (13): 13 was prepared according to the same procedure as preparation of 11 using 12 as the starting material (yield 85%). 1H NMR (400 MHz, CDCl3) δ 7.26-7.22 (m, 4H), 6.88-6.83 (m, 4H), 5.78-5.59 (m, 2H), 4.56 (d, J = 11.2 Hz, 1H), 4.45 (d, J = 11.2 Hz, 1H), 4.42 (s, 2H), 3.96 (m, 2H), 3.79 (s, 3H), 3.78 (s, 3H), 3.31-3.29 (m, 1H), 2.97-2.95 (m, 1H), 2.77 (dd, J = 5.2 Hz, J = 4.0 Hz, 1H), 2.70 (dd, J = 2.6 Hz, J = 5.2 Hz, 1H), 2.44-2.37 (m, 2H);13C NMR: (100 MHz, CDCl3) δ 159.4, 159.3, 130.6, 130.6, 130.5, 130.0, 129.6, 129.5, 129.4, 113.9, 77.6, 72.0, 71.7, 70.6, 55.4, 53.3, 45.9, 35.9.
Preparation of (S)-2-((S,E)-1,5-bis(4-methoxybenzyloxy)pent-3-enyl)aziridine (14): 14 was prepared according to the same procedure of preparation of 10 using 13 as the starting material (yield 50%, in two steps). 1H NMR (400 MHz, CDCl3) δ 7.28-7.24 (m, 4H), 6.88-6.84 (m, 4H), 5.82-5.63 (m, 2H), 4.68 (d, J = 11.2 Hz, 1H), 4.56 (d, J = 11.2 Hz, 1H), 4.42 (s, 2H), 3.95 (dd, J = 6.0 Hz, J = 0.8 Hz, 2H), 3.80 (s, 3H), 3.78 (s, 3H), 3.05 (m, 1H), 2.42-2.39 (m, 2H), 2.10-2.04 (m, 1H), 1.73-1.74 (m, 1H), 1.39 (s, 1H); 13C NMR: (100 MHz, CDCl3) δ 159.3 (2), 130.7, 130.1, 129.5, 129.4, 129.3, 113.9, 80.6, 71.8, 71.4, 70.6, 55.4, 36.9, 33.4, 21.6.
Preparation of (2S*,3S*,5R*,6S*)-methyl 2-(bromomethyl)-3-(tert-butyldiphenylsilyloxy)-1-aza-bicyclo[3.1.0]hexane-6-carboxylate (16): To a solution of 10 (122 mg, 0.3 mmol) in anhydrous DCM (50 mL) was added NBS (59 mg, 0.32 mmol). TLC indicated that the starting material 10 disappeared within 2 min to form the N-Br species. The mixture was heated at 40 oC for 48 h in the presence of 4Å molecular sieves. The reaction mixture was filtered, washed with Na2SO3 aqueous solution, and dried. The extract was concentrated and purified on a silica gel column to afford recovered starting material (13 mg), and a mixture of 16-epi (71 mg, 55% bsm, Rf 0.42 in 3:7 EtOAc/hexane) and the single diastereomer 16 (40 mg, 30% bsm, Rf 0.30 in 3:7 EtOAc/hexane). 16: 1H NMR (400 MHz, CDCl3) δ 7.62-7.68 (m, 4H), 7.40-7.44 (m, 6H), 4.33 (t, J = 4.8 Hz, 1H), 3.75 (s, 3H) 3.62-3.65 (m, 1H), 3.55 (d, J = 4.8 Hz, 2H) 3.14 (d, J = 2.4 Hz, 1H), 2.7-2.75 (m, 1H), 2.20 (d, J = 15.2 Hz, 1H), 2.02 (m, 1H), 1.10 (s, 9H); 13C NMR: (100 MHz, CDCl3) δ 170.9, 136.3, 136.2, 133.7, 132.2, 130.4, 130.2, 128.0, (2) 72.5, 71.2, 52.5, 45.8, 36.7, 27.9, 27.3, 19.4. HR-MS (ESI) m/z: calcd. for C24H31BrNO3Si [(M+H)+] 488.1251, obsd 488.1259.
Preparation of (2R*,3R*,5S*,6R*)-methyl 5-(tert-butyldiphenylsilyloxy)-3-(tosyloxy)-1-azabicyclo-[4.1.0]heptane-2-carboxylate (17): To a solution of 16 (55 mg, 0.11 mmol) in DCM (2 mL), was added p-toluenesulfonic acid monohydrate (26.6 mg, 0.14 mmol). The mixture was stirred at room temperature for 16 h. The reaction mixture was concentrated and purified on a silica gel column to afford 17 (40 mg, yield 61%) 1H NMR (400 MHz, CDCl3) δ 7.68-7.67 (m, 2H), 7.58-7.57 (m, 4H), 7.47-7.45 (m, 2H), 7.40-7.37 (m, 4H), 7.28-7.27 (m, 2H), 4.42-4.38 (m, 1H), 3.91-3.89 (m, 1H), 3.77-3.52 (m, 3H), 3.60 (s, 3H), 3.02-2.99 (m, 1H), 2.42 (s, 3H), 1.98-1.95 (m, 1H), 1.79-1.76 (m, 1H). 13C NMR: (100 MHz, CDCl3) δ 170.3, 145.0, 135.9, 135.8, .133.9, 133.2, 132.9, 130.4, 130.3, 130.0, 128.2, 128.1, 128.0, 68.3, 57.5, 56.1, 52.8, 35.4, 31.8, 31.0, 27.1, 21.9, 19.4.
Preparation of (2S,4S,5S)-2-((S)-1-bromo-2-(4-methoxybenzyloxy)ethyl)-4-(4-methoxybenzyloxy)-1-azabicyclo[3.1.0]hexane (18). To a mixture of 14 (200 mg, 0.52 mmol) in anhydrous DCM (150 mL) at ice bath temperature, was added NBS (112 mg, 0.63 mmol). The mixture was stirred for 1 h at ice bath temperature. TLC indicated all of the starting material was converted to the N-Br intermediate. To the reaction mixture was added trifluoroacetic acid (24 µL, 0.313 mmol). The mixture was stirred continuously at ice bath temperature for 8 h until most of the N-Br intermediate was consumed. Triethylamine (30 µL, 0.22 mmol) was added. The reaction mixture was concentrated and purified on a silica gel column to afford 18 (90 mg, the other diastereomeric product 62 mg, combined yield 57%). 1H NMR (400 MHz, CDCl3) δ 7.31-7.27 (m, 4H), 6.90-6.87 (m, 4H), 4.57-4.40 (m, 4H), 4.09-3.99 (m, 2H), 3.81 (s, 3H), 3.80 (s, 3H), 3.80 (m, 1H), 3.62-3.59 (m, 1H), 2.60-2.59 (m, 1H), 2.22-2.18 (m, 1H), 1.86-1.85 (m, 1H), 1.54-1.42 (m, 2H), 13C NMR: (100 MHz, CDCl3) δ 159.6, 159.5, 130.2, 130.1, 129.7, 129.6, 114.1, 114.0, 78.1, 73.2, 72.5, 63.3, 55.5 (2C), 54.6, 41.3, 31.3, 22.0 HR-MS (ESI) m/z: calcd. for C23H29BrNO4 462.1274, obsd 462.1282.
Preparation of (2S,4S,5S)-2-((R)-1-azido-2-(4-methoxybenzyloxy)ethyl)-4-(4-methoxybenzyloxy)-1-azabicyclo[3.1.0]hexane (19): To a solution of 18 (25 mg, 0.054 mmol) in DMF (1 mL) was added NaN3 (35 mg, 0.54 mmol). The mixture was stirred at room temperature for 48 h. The reaction mixture was diluted with brine (3 mL) and EtOAc (5 mL). The organic layer was separated, dried over MgSO4 and concentrated. The resulting residue was purified on a silica gel column to afford 19 (20 mg, yield 88%). 1H NMR (400 MHz, CDCl3) δ 7.27-7.26 (m, 4H), 6.89-6.87 (m, 4H), 4.68-4.61 (m, 1H), 4.52-4.42 (m, 4H), 4.25-4.21 (m, 1H) 3.80 (s, 3H), 3.72 (s, 3H), 3.70-3.66 (m, 2H), 3.58-3.52 (m, 1H), 2.60-2.58 (m, 1H), 2.15-2.10 (m, 1H), 1.82 (d, J = 5.2 Hz, 1H), 1.72-1.67 (m, 2H) 13C NMR: (100 MHz, CDCl3) δ 159.6 (2), 130.5, 130.0, 129.6 (2), 114.1(2), 79.6, 73.1, 72.4, 71.7, 57.9, 55.5 (2), 42.7, 31.6, 28.3. HR-MS (ESI) m/z: calcd. for C23H29N4O4 425.2183, obsd 465.2180.
ACKNOWLEDGEMENTS
We thank NSERC, Canada Foundation for Innovation, ORDCF, and the University of Toronto for financial support.
References
1. A. D. Argoudelis, F. Reusser, and H. A. Whaley, J. Antibiot., 1976, 29, 1001. CrossRef
2. M.-S. Kuo, D. A. Yurek, and S. A. Mizsak, J. Antibiot., 1989, 42, 357. CrossRef
3. (a) I. D. G. Watson, L. Yu, and A. K. Yudin, Acc. Chem. Res., 2006, 39, 194; CrossRef (b) P. Lowden, in Aziridines and Epoxides in Organic Synthesis; ed. by A. K. Yudin; Wiley-VCH: Weinheim, Germany, 2006, p. 399. CrossRef
4. Z. Zhao, Ph.D. thesis, University of California, Los Angeles, CA, 1993.
5. F. Reusser, Biochemistry, 1977, 16, 3406. CrossRef
6. R. S. Coleman, R. J. Perez, C. H. Burk, and A. Navarro, J. Am. Chem. Soc., 2002, 124, 13008. CrossRef
7. (a) J. T. Millard, M. F. Weidner, S. Raucher, and P. B. Hopkins, J. Am. Chem. Soc., 1990, 112, 36387; (b) R. M. Williams and S. R. Rajski, Tetrahedron Lett., 1992, 33, 2929; CrossRef (c) J. Woo, S. T. Sigurdsson, and P. B. Hopkins, J. Am. Chem. Soc., 1993, 115, 1199. CrossRef
8. D. Paumier, M. Garcia, M. Shipman, and J. C. Muir, Synlett, 2004, 2212. CrossRef
9. (a) R. S. Coleman, J. Li, and A. Navarro, Angew. Chem. Int. Ed., 2001, 40, 1736; CrossRef (b) R. S. Coleman, J.-S. Kong, and T. E. Richardson, J. Am. Chem. Soc., 1999, 121, 9088. CrossRef
10. M. Sasaki and A. K. Yudin, J. Am. Chem. Soc., 2003, 125, 14242. CrossRef
11. G. Chen, M. Sasaki, X. Li, and A. K. Yudin, J. Org. Chem., 2006, 71, 6067. CrossRef
12. For alcohol to guanidine transformation, see: D. S. Dodd and A. P. Kozikowski, Tetrahedron Lett., 1994, 35, 977. CrossRef
13. R. Zibuck and J. M. Streiber, J. Org. Chem., 1989, 54, 4717. CrossRef
14. (a) M. Sasaki and A. K. Yudin, Synlett, 2004, 2443; CrossRef (b) T. R. Hoye, L. C. Kopel, and T. D. Ryba, Synthesis, 2006, 1572. CrossRef
15. J. Legters, L. Thijs, and B. Zwanenburg, Tetrahedron Lett., 1989, 30, 4881. CrossRef
16. Y. Gao, R. M. Hanson, J. M. Klunder, S. Y. Ko, H. Masamune, and K. B. Sharpless, J. Am. Chem. Soc., 1987, 109, 5765. CrossRef
17. A. B. Pangborn, M. A. Giardello, R. H. Grubbs, R. K. Rosen, and F. J. Timmers, Organometallics, 1996, 15, 1518. CrossRef
18. R. Zibuck and J. M. Streiber, J. Org. Chem., 1989, 54, 4717. CrossRef