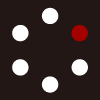
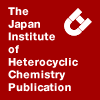
HETEROCYCLES
An International Journal for Reviews and Communications in Heterocyclic ChemistryWeb Edition ISSN: 1881-0942
Published online by The Japan Institute of Heterocyclic Chemistry
e-Journal
Full Text HTML
Received, 13th August, 2013, Accepted, 3rd October, 2013, Published online, 16th October, 2013.
DOI: 10.3987/COM-13-S(S)111
■ Synthesis of Pyrrolidine 3,4-Diol Derivatives with Anticancer Activity on Pancreatic Tumor Cells
Frederic Steimer, Ana T. Carmona, Antonio J. Moreno-Vargas, Irene Caffa, Michele Cea, Fabrizio Montecucco, Alessio Nencioni, Pierre Vogel, and Inmaculada Robina*
Department of Organic Chemistry, University of Seville, Prof. Garcia Gonzalez, 1 Seville-41012, Spain
Abstract
Novel pyrrolidine 3,4-diol derivatives of the type (2R and 2S,3R,4S)-{[((1R)-1-methoxycarbonyl and hydroxylmethyl)-1-arylmethyl)amino]ethyl}-pyrrolidine-3,4-diol have been prepared and evaluated as α-mannosidase inhibitors and assayed for their anticancer activity in vitro. They all exhibit specific but moderate activity as inhibitors towards α-mannosidase from Jack beans. Compounds 7 and 8b bearing hydroxymethyl and trifluoromethylbiphenyl groups show the best antiproliferative effect in two pancreatic cancer cell lines.INTRODUCTION
Glycoproteins are expressed in all cell compartments and have a wide range of roles, including functions as receptors, transporters and structural proteins. In particular, glycoproteins are frequently involved in cell-cell communication, cell-cell recognition and cell adhesion. Iminosugars have previously been shown to effectively interfere with glycoprotein quality control, maturation, transport and secretion, thereby altering cell-cell recognition processes. More than twenty glycoproteins have already been identified as regulators of cell division, differentiation and maturation, and to affect the development of macrophages.1 Impaired cell adhesion and immune recognition are key features of oncogenesis and metastasis. Thus, targeting the metabolism and processing of glycoproteins with selective inhibitors seems to be a viable approach for treating cancer.
Several glycosidase inhibitors, some of which isolated from natural sources, have shown activity as anticancer agents. Castanospermine, an α-glucosidase I inhibitor, has been shown to block platelet aggregation, angiogenesis, tumor colonization and growth.2 The non-natural enantiomer of (+)-lentiginosine (a selective amyloglucosidase inhibitor), (–)-lentiginosine induces apoptosis in various types of cancer cells.3 The α-mannosidase I inhibitor 1-deoxymannojirimycin and swainsonine, an iminoalditol with potent inhibitory activity on lysosomal α-mannosidase and Golgi α-mannosidase II, both inhibit human melanoma cell invasion.4 Unfortunately, phase 1B clinical trials have shown adverse secondary effects for swainsonine. In addition, a phase II study of oral swainsonine in patients gave no evidence of anti-tumor activity for this agent. Several derivatives of swainsonine with substituents at 3-, 5-, 6- and 7 positions were synthesized and their anti-cancer properties evaluated but none of them could reach the promising effects of the parent compound. Albeit less active than swainsonine, these compounds provided important information on the Golgi α-mannosidase II active site allowing the subsequent design of swainsonine-like inhibitors.5
1,4-Dideoxy-1,4-imino-D-ribitol (1) (Figure 1) is a natural product with important biological activities. It was first isolated from the root bark of Mulberry tree (Morus Alba L.). This compound and other polyhydroxylated pyrrolidines have been studied in high details because of their ability to mimic their furanose analogues and, consequently, to interact with carbohydrate-processing enzymes. Iminoribitol 1 is a weak inhibitor6 of several α-D-glucosidases (bovine liver lysosomal, rat liver endoplasmatic reticulum II, rat liver lysosomal) and of α-D-mannosidase from rat liver Golgi II apparatus as well. It also shows a moderate inhibitory activity toward α-D-glucosidase from yeast (Ki = 23 µM).7 In addition to its glycosidase inhibitory activity, 1,4-dideoxy-1,4-imino-D-ribitol strongly inhibits eukaryotic DNA polymerases with almost no activity neither on prokaryotic DNA polymerases, nor on DNA metabolic enzymes.8 These proprieties suggested a potential clinical application of 1 as anti-HIV agent.9 This compound was proposed also to be a potential anti-neoplastic and anti-proliferative agent, but the in vitro studies on cancer cell lines did not evidence any cytotoxic activity, probably because of its high polarity which prevents it from crossing the cell membranes.
For the rapid discovery of α-mannosidase inhibitors, we have developed a combinatorial method based on imines derived from (2R,3R,4S)-2-aminomethyl-3,4-dihydroxypyrrolidine scaffolds. These compounds can be considered as transition state or intermediate structure mimics of the hydrolytic process catalyzed by α-mannosidases.10
This method led to the discovery of a lead compound bearing a 1,4-dideoxy-1,4-imino-D-ribitol moiety and to the corresponding (R)-2-amino-2-phenylethanol derivative 2. Notably, although 2 exhibited a Ki of 135 nM towards Jack beans α-mannosidase, it failed to inhibit cancer cell growth in culture.11 This was ascribed to the hydrophilic nature of this compound that may prevent its cell internalization. This hypothesis is supported by the observation that the more lipophilic compound 3 is able to inhibit both glioblastoma and melanoma cell growth selectively.11
Several 2-methyleneamino derivatives of 1 were synthesized in order to increase its lypophilicity and cell membrane permeability leading to new and selective α-mannosidase inhibitors with promising anti-cancer activity.12 Further studies allowed the identification of biaryl-containing dihydroxypyrrolidine analogues, such as 4, which showed to be potent and selective jack beanss α-mannosidase inhibitor (Ki = 2.7 μM).13 It also displayed potent anticancer activity in cell lines of different histology and in primary leukemic cells (Figure 2). Derivatization of iminosugars with aliphatic side chains has been extensively studied in order to improve their biological activity, lipophilicity and bioavailability.14 For instance, N-butyl-1-deoxynojirimycin (Zavesca) is a drug for the treatment of Gaucher's disease,15 N-nonyl-1-deoxynojirimycin inhibits hepatitis B virus in cell based assays,16 and α-1-C-alkyl-1-deoxynojirimycin derivatives have shown inhibitory activity toward intestinal isomaltase.17 N-Dodecyl-D-fagomine derivatives are α-glucosidase inhibitors that exhibit anticancer activity against stomach, colon, pancreas, and breast cancer cell lines with EC50's ranging between 100 to 250 μM for the dodecyl derivative.18 The α-L-fucosidase inhibitor N-dodecyl hydroxylated pyrrolidine 5 also demonstrated anti-proliferative activity in stomach, colon, pancreas, and breast cancer cell lines at 2 μM.19 5-O-Oleyl 1,4-dideoxy-1,4-imino-D-ribitol (6) and the related 5-O-conjugates (linolenyl, linoleyl and octadecyl) derivatives were evaluated as enzyme inhibitors and for their toxicity on solid and haematological malignancies and indeed exhibited activities in the μM range (Figure 2).19 Although these compounds are not α-mannosidase inhibitors (data not shown), they demonstrated marked cytotoxicity on solid and haematological malignancies in the μM range, their activity essentially correlating with the length of their alkyl side chain. In particular, the oleyl-conjugate 6 showed to be the most effective at killing cancer cells in culture.
With the hope to improve our understanding of the mode of action of diamines such as 4 both on α-mannosidases and cancer cells, which we proposed to be modelled by the transition state mimic I (Figure I), we decided to explore what would be the effect in changing the distance between both amino moieties that interact with the two carboxylic groups at the α-mannosidase active site. We thus embarked on the synthesis of 7, the homologue of 4, keeping the same absolute configuration (2'R,2R,3R,4S). As we found 7 to be a bad inhibitor of α-mannosidase from Jack beans, we then explored what would be the effect of inverting configuration at C(2) of the pyrrolidine moiety. To our surprise, compound 8b, the 2-epimer of 7 showed α-mannosidase inhibitory activity, but not to the extent of that of 4 (4 (Ki = 2.7 μM) vs 8b (Ki = 120 μM)). As both 7 and 8b inhibited pancreas cell growth in vitro, we prepared more analogues of 7, 8b and 9 with the hope to find a potential drug against pancreas cancer (Figure 3) based on our models for transition state mimics of the hydrolytic process catalyzed by α-mannosidases (Figures 1 and 7).10
Our preliminary results are presented in this report and we shall show that the best tumor cell growth inhibitors are not necessarily the best α-mannosidase inhibitors.
RESULTS AND DISCUSSION
Based on previous results,13 we report herein the preparation and biological evaluation of new biphenylpyrrolidines. These were synthesized under the assumption that the biphenyl group would enhance their lipophilic character and thus facilitate compound internalization through the plasma membrane. The influence of the stereocenter at C-2 and other modifications (at the end of the aromatic moiety and at the side chain) on the ability of these compounds to inhibit α-mannosidase, as well as on their anticancer activity were also studied (Figure 4).
The new compounds were prepared from carbaldehydes 10 and 11 which were obtained from L-arabinose by applying a methodology developed in our group.20
Derivatives of phenyl glycine 12 and 13 were prepared by Suzuki coupling as was previously described by Bello, Vogel and co-workers.13 The final compounds were synthesized from aldehydes 10 and 11 through reductive amination with the corresponding substituted phenylglycines followed by hydrolysis or reduction and hydrolysis (Schemes 1 - 3). All the compounds were obtained in moderate-to-good overall yields.
BIOLOGICAL EVALUATION
The new compounds were tested for their inhibitory activity against 11 glycosidases21 from different sources, and as inhibitors of the growth of cancer cells in two pancreatic cell lines MiaPaCa2 and PK9. All the tested compounds were found to be selective and moderate-to-good Jack beans α-mannosidase inhibitors (see Figure 6 for % of inhibition and IC50).22 Compounds bearing an ester function at the side chain of the pyrrolidine framework (17a-d, 19 and 15) showed a poor inhibitory activity (less than 50% at 1 mM) when compared to the corresponding hydroxy derivatives (8a-d, 9), suggesting the requirement of the hydroxyl group for compound binding to the enzymatic active site.
We attribute the lower α-mannosidase inhibitory activity of 7 compared to that of 4 to the too long distance separating the two amino groups. Its simultaneous interaction with the two carboxylic moieties of the enzyme becomes too costly as shown in transition state model II (Figure 7). On the contrary, in the case of 8b, the 2-epimer of 7, the separation between the two amino groups becomes shorter than that in II and thus 8b can imitate 4 more than 7 as shown with III.
Analogues of 4 in which the p-trifluoromethylbiphenyl group is substituted by other biphenyl or p-substituted phenyl systems were less active than 4 both toward α-mannosidase and cancer cells. However, it is a surprise also to find that compound 8a with a biphenyl group is more active toward α-mannosidase than 7 and 8b. As another surprise, 7 and 8b (EC50 = 12-13 µM) are found to be the most potent pancreas cancer cell growth inhibitors of this study (Table 1). Finally we decided to test the effect of inverting C(2') of the arylethanol group of 8a. This led to the preparation of 9, a compound which appears to inhibit α-mannosidase and the growth of pancreas cancer cells as well as 8a. However this is not the case for 7 and 8b that present the best antiproliferative effect and are weak and moderate α-mannosidase inhibitors.
CONCLUSIONS
A small library of new 3,4-dihydroxypyrrolidine analogues has been prepared starting from commercially available L-arabinose and 4-hydroxyphenylglycine methyl ester. The synthetic steps were straightforward and allowed their synthesis in moderate-to-good overall yields. The new compounds were assessed for their ability to inhibit glycosidases from different sources and for their antiproliferative effect on two pancreatic cancer cell lines. The enzyme inhibition measurements showed good selectivity towards α-mannosidase from Jack beans with respect to other glycosidases. Nevertheless, homologation of the imino-D-ribitol side chain diminished the inhibitory activity which can be assigned to the longer distance separating the two amino groups. This prevents the simultaneous interaction of the amino groups with the two carboxylic moieties of the enzyme according to transition state model II (Figure 7). However, we found better inhibitory activity for the all-cis compounds such as 8 and 9. As interpreted with model III (Figure 7), the distance between the two amino moieties is shortened, thus imitating 4 better and, as a consequence, improving the inhibitory activity.
The best biologically active compounds in this series possess a hydroxymethyl moiety. Compounds 7 and 8b bearing an additionally trifluoromethybiphenyl group exert the best antiproliferative effect on the tested cancer cell lines. The hydroxymethyl moiety is important for the α-mannosidase inhibition while the trifluoromethybiphenyl group is important (as other biphenyl groups) for the cancer cell growth inhibition. This is expected as the biphenyl moieties help the inhibitor to penetrate cell membranes. The absence of correlation of α-mannosidase inhibitory and cell growth inhibitory action might be the result of differences in cell penetration of our compounds. From the data presented in this paper, it is possible that the cell growth inhibitory activity of our compounds is not due to α-mannosidase inhibition. These preliminary studies will be followed by the synthesis of a large library of analogues with the hope to find a chemotherapeutic agent to fight pancreas cancer and other cancers, and to find the mechanism of action of these compounds.
EXPERIMENTAL
General methods.
Optical rotations were measured in a 1.0 cm or 1.0 dm tube with a Perkin–Elmer 241MC spectropolarimeter. 1H and 13C NMR spectra were obtained for solutions in CDCl3, [d6]DMSO and CD3OD; J values are given in Hz and δ in ppm. All the assignments were confirmed by two-dimensional NMR experiments (COSY and HSQC). NMR and Mass spectra were registered in CITIUS (University of Seville). TLC was performed on silica gel HF254 (Merck), with detection by UV light charring with ninhydrine or with Pancaldi reagent [(NH4)6MoO4, Ce(SO4)2, H2SO4, H2O]. Silica gel 60 (Merck, 230 mesh) was used for preparative chromatography. Glycosidases and the corresponding p-nitrophenyl-O-glycoside substrates for inhibition assays were purchased in Sigma-Aldrich.
General procedure for reductive amination of α-aminoesters 12 and 13 with aldehydes 10 and 11.
To a solution of aldehyde 10 (0.285 g, 1.0 mmol) in anhydrous 1,2-dichloroethane (5.5 mL), α-amino ester derivative 12 (1.1 mmol) in 1,2-dichloroethane (4 mL) and NaBH(OAc)3 (0.297 g, 1.4 mmol) were added under Ar. The reaction was stirred overnight at rt. Then, aq. sat. sol. of NaHCO3 was added and the mixture extracted with AcOEt, dried (Na2SO4), filtered, evaporated in vacuo followed by flash column chromatography on silica gel (AcOEt:cyclohexane 1:2).
The following compounds were obtained:
(2S,3R,4S) N-tert-Butoxycarbonyl-2-{{{(1R)-1-methoxycarbonyl-1-[[1,1’-biphenyl]-4-yl]methyl}-amino}ethyl}-3,4-O-isopropylidenepyrrolidine-3,4-diol (16a)
Reductive amination of 11 and 12a following the general procedure gave pure 16a as a colourless oil (0.343 g, 67% yield). [α]D25 +17.5 (c 0.74, CH2Cl2). IR νmax 3330, 2978, 1737, 1687, 1110, 1083, 867, 698 cm-1. 1H NMR (300 MHz, CDCl3, δ ppm, J Hz, mixture of rotamers) δ 7.59-7.53 (m, 4H, Ar-H), δ 7.48- 7.39 (m, 4H, Ar-H), 7.34 (m, 1H, Ar-H), 4.73-4.62 (m, 2H, H-3, H-4), 4.44 (m, 1H, CHCOOCH3), 4.05-3.93 (m, 1H, H-2), 3.92-3.77 (m, 1H, H-5a) 3.71 (s, 3H, CHCOOCH3), 3.29-3.18 (m, 1H, H-5b), 2.76-2.56 (m, 2H, H-2’a, H-2’b), 2.01-1.88 (m, 3H, H-1’a, H-1’b, NH), 1.49 (m, 3H, C(CH3)2), 1.42 (s, 9H, C(CH3)3), 1.31 (m, 3H, C(CH3)2). 13C NMR (75.4 MHz, CDCl3, δ ppm) δ 173.5, 154.3, 141.0, 140.7, 137.3, 128.8, 127.9, 127.4, 127.4, 127.1, 112.9, 80.0, 79.8, 77.8, 65.3, 57.3, 52.2, 50.1, 44.9, 29.7, 28.4, 26.6, 25.2. CIHRMS m/z found 511.2824, calcd. for C29H39N2O6 (M+H)+: 511.2808.
(2S,3R,4S) N-tert-Butoxycarbonyl-2-{{{(1R)-1-methoxycarbonyl-1-[(4’-(trifluoromethyl)-[1,1’-biphenyl]-4-yl]methyl}amino}ethyl}-3,4-O-isopropylidenepyrrolidine-3,4-diol (16b)
Reductive amination of 11 and 12b following the general procedure gave pure 16b as a colourless oil (0.338 g, 58% yield). [α]D25 +12.3 (c 1.05, CH2Cl2). IR νmax 3345, 2981, 1736, 1684, 1119, 1086, 861, 734 cm-1. 1H NMR (300 MHz, CDCl3, δ ppm, J Hz, mixture of rotamers) δ 7.72-7.63 (m, 4H, Ar-H), δ 7.56 (d, 2H, J = 8.3, Ar-H), 7.49 (d, 2H, J = 8.3, Ar-H), 4.80-4.63 (m, 2H, H-4, H-3), 4.44 (m, 1H, CHCOOCH3), 4.00 (m, 1H, H-2), 3.86 (m, 1H, H-5a) 3.71 (s, 3H, CHCOOCH3), 3.28-3.19 (m, 1H, H-5b), 2.77-2.56 (m, 2H, H-2’a, H-2’b), 2.04-1.85 (m, 3H, H-1’a, H-1’b, NH), 1.53-1.47 (m, 3H, C(CH3)2), 1.40 (s, 9H, C(CH3)3), 1.36-1.28 (m, 3H, C(CH3)2). 13C NMR (75.4 MHz, CDCl3, δ ppm) δ 173.3, 154.3, 144.2, 144.2, 139.5, 128.1, 127.5, 127.3, 125.7, 112.9, 80.0, 79.8, 77.8, 65.1, 57.7, 52.3, 49.5, 44.9, 29.7, 28.3, 26.6, 25.2. CIHRMS m/z found 579.2688, calcd. for C30H38F3N2O6 (M+H)+: 579.2682.
(2S,3R,4S) N-tert-Butoxycarbonyl-2-{{{(1R)-1-methoxycarbonyl-1-{4-[(phenylmethoxy)phenyl]}-methyl}amino}ethyl}-3,4-O-isopropylidenepyrrolidine-3,4-diol (16c)
Reductive amination of 11 and 12c following the general procedure gave pure 16c (0.30 g, 70% yield). [α]D25 +0.3 (c 0.93, CH2Cl2). IR νmax 3326, 2977, 1737, 1689, 1107, 1082, 863, 697 cm-1. 1H NMR (300 MHz, CDCl3, δ ppm, J Hz) δ 7.39-7.18 (m, 7H, Ar-H), δ 6.90- 6.83 (m, 2H, Ar-H), 4.97 (s, 2H, O-CH2-C-arom.), 4.66-4.54 (m, 2H, H-3, H-4), 4.27 (s, 1H, CHCOOCH3), 3.90 (m, 1H, H-2), 3.76 (m, 1H, H-5a), 3.61 (s, 3H, CHCOOCH3), 3.15 (m, 1H, H-5b), 2.63-2.47 (m, 2H, H-2’a, H-2’b), 2.03-1.77 (m, 3H, H-1’a, H-1’b, N-H), 1.42 (s, 3H, C(CH3)2), 1.35 (s, 9H, C(CH3)3), 1.24 (s, 3H, C(CH3)2). 13C NMR (75.4 MHz, CDCl3, δ ppm) δ 173.7, 158.6, 154.3, 136.9, 130.6, 128.6, 128.0, 127.4, 115.0, 112.8, 80.0, 79.8, 77.8, 70.0, 64.8, 57.7, 52.1, 50.1, 44.8, 29.6, 28.4, 26.6, 25.2. CIHRMS m/z found 541.2906, calcd. for C30H41N2O7 (M+H)+: 541.2914.
(2S,3R,4S) N-tert-Butoxycarbonyl-2-{{{(1R)-1-methoxycarbonyl-1-{4-[(prop-2-enyloxy)phenyl]}-methyl}amino}ethyl}-3,4-O-isopropylidenepyrrolidine-3,4-diol (16d)
Reductive amination of 11 and 12d following the general procedure gave pure 16d (0.269 g, 59% yield). [α]D25 +0.3 (c 1.14, CH2Cl2). IR νmax 3330, 2980, 1737, 1690, 1109, 1083, 862 cm-1. 1H NMR (300 MHz, CDCl3, δ ppm, J Hz, mixture of rotamers) δ 7.28 (d, 2H, J = 8.8, Ar-H), 6.86 (d, 2H, J = 8.8, Ar-H), 6.04 (m, 1H, CH2=CHCH2O), 5.39 (dq, 1H, J = 17.4, J = 1.6, CH2=CHCH2O), 5.27 (dq, 1H, J = 10.5, J = 1.5, CH2=CHCH2O), 4.80-4.62 (m, 2H, H-3, H-4), 4.53-4.49 (m, 2H, CH2=CHCH2O), 4.35 (m, 1H, CHCOOCH3), 4.01-3.78 (m, 2H, H-2, H-5a), 3.67 (s, 3H, COOCH3), 3.22 (m, 1H, H-5b), 2.71- 2.51 (m, 2H, H-2’a, H-2’b), 2.01-1.83 (m, 3H, H-1’a, H-1’b, N-H), 1.50 (m, 3H, C(CH3)2), 1.41 (s, 9H, C(CH3)3), 1.33 (2m, 3H, C(CH3)2). 13C NMR (75.4 MHz, CDCl3, δ ppm) δ 173.7, 158.4, 154.3, 133.2, 128.6, 128.6, 117.7, 114.8, 112.8, 80.0, 79.8, 77.8, 68.8, 64.8, 57.7, 52.1, 49.5, 44.8, 29.6, 28.4, 26.6, 25.2. CIHRMS m/z found 491.2755, calcd. for C26H39N2O7 (M+H)+: 491.2757.
(2R,3R,4S) 2-{{{(1R)-2-Hydroxy-1-[(4’-(trifluoromethyl)-[1,1’-biphenyl]-4-yl]ethyl}amino}ethyl}pyrolidine-3,4-diol (7)
Reductive amination of aldehyde 10 and amine 12b afforded 14 (0.48 g, 95% yield). [α]D25 + -16.4 (c 0.79, CH2Cl2). 1H NMR (300 MHz, CDCl3, δ ppm, J Hz) δ 7.64-7.56 (m, 4H, Ar-H), 7.54-7.46 (m, 2H, Ar-H), 7.46-7.36 (m, 2H, Ar-H), 4.60 (m, 1H, H-4), 4.38-4.30 (m, 2H, CHCOOCH3, H-3), 4.08 (m, 1H, H-2), 3.82 (m, 1H, H-5a) 3.64 (s, 3H, CHCOOCH3), 3.17 (m, 1H, H-5b), 2.70-2.40 (m, 2H, H-2’a, H-2’b), 1.90 (m, 1H, N-H), 1.64-1.47 (m, 2H, H-1’a, H-1’b), 1.36 (2s, 9H, C(CH3)2, C(CH3)3), 1.21 (s, 3H, C(CH3)2). CIHRMS m/z found 579.2690, calcd. for C30H38F3N2O6 (M+H)+: 579.2682.
Reduction of 14 afforded protected alcohol (2R,3R,4S) N-tert-butoxycarbonyl-2-{{{(1R)-2-hydroxy-1-[(4’-(trifluoromethyl)-[1,1’-biphenyl]-4-yl]ethyl}amino}ethyl}-3,4-O-isopropylidenepyrrolidine-3,4-diol (0.079 g, 53% yield). [α]D25 -9.1 (c 0.86, CH2Cl2). IR νmax 3437, 2977, 1686, 1070, 823, 732 cm-1. 1H NMR (300 MHz, CDCl3, δ ppm, J Hz, mixture of rotamers) δ 7.72-7.62 (m, 4H, Ar-H), 7.69-7.50 (m, 2H, Ar-H), 7.47-7.35 (m, 2H, Ar-H), 4.66 (m, 1H, H-4), 4.38 (m, 1H, H-3), 4.30 (m, 1H, H-2), 4.13-3.52 (m, 4H, H-5a, CHCH2OH, CH2OH), 3.23 (m, 1H, H-5b), 2.77-2.15 (m, 4H, H-2’a, H-2’b, N-H, O-H), 1.71-1.53 (m, 2H, H-1’a, H-1’b), 1.44 (m, 12H, C(CH3)2, C(CH3)3), 1.28 (s, 3H, C(CH3)2). CIHRMS m/z found 551.2739, calcd. for C29H38F3N2O5 (M+H)+: 551.2733.
Subsequent deprotection of the above protected alcohol (0.1 mmol) afforded 7 in quantitative yield. [α]D25 +4.7 (c 0.65, MeOH). IR νmax 3341, 2934, 1127, 1068, 823 cm-1. 1H NMR (300 MHz, MeOD, δ ppm, J Hz) δ 7.90-7.81 (m, 4H, Ar-H), δ 7.81-7.75 (m, 2H, Ar-H), 7.53-7.68 (m, 2H, Ar-H), 4.52 (m, 1H, H-4), 4.24 (m, 1H, H-3), 4.09-3.97 (m, 3H, CHCH2OH, CH2OH), 3.58-3.42 (m, 2H, H-2, H-5a), 3.30-3.05 (m, 3H, H-2’a, H-2’b, H-5b), 2.46-2.23 (m, 2H, H-1’a, H-1’b). 13C NMR (75.4 MHz, MeOD, δ ppm) δ 133.9, 130.4, 129.3, 128.7, 126.9, 77.0, 70.5, 65.2, 63.3, 59.0, 49.9-48.2, 44.2, 28.1. CIHRMS m/z found 411.1908, calcd. for C21H26F3N2O3 (M+H)+: 411.1896.
(2S,3R,4S) N-tert-Butoxycarbonyl-2-{{{(1S)-1-methoxycarbonyl-1-[[1,1’-biphenyl]-4-yl]methyl}-amino}ethyl}-3,4-O-isopropylidenepyrrolidine-3,4-diol (18)
Reductive amination of aldehyde 11 and amine 13 afforded 18 (0.186 g, 36% yield). [α]D25 + 37.0 (c 1.19, CH2Cl2). IR νmax 2979, 1737, 1691, 1160, 1078, 866, 699 cm-1. 1H NMR (300 MHz, CDCl3, δ ppm, J Hz, mixture of rotamers) δ 7.60-7.53 (m, 4H, Ar-H), δ 7.48-7.39 (m, 4H, Ar-H), 7.34 (m, 1H, Ar-H), 4.72-4.62 (m, 2H, H-3, H-4), 4.44 (m, 1H, CHCOOCH3), 3.99 (m, 1H, H-2), 3.84 (m, 1H, H-5a) 3.71 (s, 3H, CHCOOCH3), 3.24 (m, 1H, H-5b), 2.77-2.56 (m, 2H, H-2’a, H-2’b), 2.09-1.88 (m, 3H, H-1’a, H-1’b, NH), 1.49 (m, 3H, C(CH3)2), 1.42 (s, 9H, C(CH3)3), 1.31 (m, 3H, C(CH3)2). 13C NMR (75.4 MHz, CDCl3, δ ppm) δ 173.5, 154.3, 141.0, 140.7, 137.3, 128.8, 128.5, 127.9, 127.4, 127.1, 112.8, 80.1, 80.0, 77.7, 65.3, 57.7, 52.2, 50.2, 50.0, 29.7, 28.4, 26.6, 25.2. CIHRMS m/z found 511.2800, calcd. for C29H39N2O6 (M+H)+: 511.2808.
General procedure for acidic deprotection of compounds 14, 16 and 18
Pyrrolidines 14, 16 or 18 (0.1 mmol) were dissolved in a mixture of HCl(4M):THF 2:1 (4.5 mL) and stirred at rt overnight. Evaporation of the solvent quantitatively afforded compounds 15, 17 or 19.
The following compounds were obtained:
(2S,3R,4S) 2-{{{(1R)-1-Methoxycarbonyl-1-[[1,1’-biphenyl]-4-yl]methyl}amino}ethyl}pyrrolidine-3,4-diol (17a)
Compound 17a (·HCl) was obtained in quantitative yield as a white solid. [α]D25 -2.8 (c 0.78, MeOH). IR νmax 3367, 2949, 1736, 1234, 1134, 756, 697 cm-1. 1H NMR (300 MHz, MeOD, δ ppm, J Hz) δ 7.84-7.76 (m, 2H, Ar-H), δ 7.70- 7.60 (m, 4H, Ar-H), 7.53-7.44 (m, 2H, Ar-H), 7.40 (m, 1H, Ar- H), 5.41 (s, 1H, CHCOOCH3), 4.42 (m, 1H, H-4), 4.16 (m, 1H, H-3), 3.86 (s, 3H, CHCOOCH3), 3.69 (m, 1H, H-2), 3.45 (m, 1H, H-5a), 3.25 (m, 1H, H-2’a), 3.19-3.04 (m, 2H, H-2’b, H-5b), 2.56-2.14 (m, 2H, H-1’a, H-1’b). 13C NMR (75.4 MHz, MeOD, δ ppm) δ 169.6, 145.0, 140.9, 130.6, 130.1, 129.9, 129.4, 129.3, 128.1, 71.9, 71.3, 64.2, 60.4, 54.4, 49.9-48.2, 44.3, 25.0. CIHRMS m/z found 371.1967, calcd. for C21H27N2O4 (M+H)+: 371.1971.
(2S,3R,4S) 2-{{{(1R)-1-Methoxycarbonyl-1-[(4’-(trifluoromethyl)-[1,1’-biphenyl]-4-yl]methyl}amino)ethyl)pyrrolidine-3,4-diol (17b).
Compound 17b (·HCl) was obtained in quantitative yield. [α]D25 -9.9 (c 0.67, MeOH). IR νmax 3359, 2951, 1745, 1325, 1111, 1070, 825 cm-1. 1H NMR (300 MHz, MeOD, δ ppm, J Hz) δ 7.88 (d, 4H, J = 8.2, Ar-H), δ 7.79 (d, 2H, J = 8.2, Ar-H), 7.70 (d, 2H, J = 8.2, Ar-H), 5.42 (s, 1H, CHCOOCH3), 4.42 (m, 1H, H-4), 4.15 (m, 1H, H-3), 3.86 (s, 3H, CHCOOCH3), 3.74 (m, 1H, H-2), 3.40 (m, 1H, H-5a), 3.30-3.04 (m, 3H, H-2’a, H-2’b, H-5b), 2.55-2.18 (m, 2H, H-1’a, H-1’b). 13C NMR (75.4 MHz, MeOD, δ ppm) δ 169.4, 144.7, 143.2, 131.2, 130.8, 129.7, 128.8, 127.0, 71.9, 71.3, 64.1, 60.3, 54.4, 49.9-48.2, 44.3, 25.0. CIHRMS m/z found 439.1850, calcd. for C22H26F3N2O4 (M+H)+: 439.1845.
(2S,3R,4S) 2-{{{(1R)-1-Methoxycarbonyl-1-{4-[(phenylmethoxy)phenyl]}methyl}amino}ethyl}pyrrolidine-3,4-diol (17c)
Compound 17c (·HCl) was obtained in quantitative yield. [α]D25 -29.8 (c 0.67, MeOH). IR νmax 3353, 2930, 1736, 1231, 1181, 738, 696 cm-1. 1H NMR (300 MHz, MeOD, δ ppm, J Hz) δ 7.53-7.28 (m, 6H, Ar-H), δ 7.19-7.09 (m, 2H, Ar-H), 6.90 (m, 1H, Ar-H), 5.23 (s, 1H, CHCOOCH3), 5.15 (s, 2H, O-CH2-Ph), 4.41 (m, 1H, H-4), 4.14 (m, 1H, H-3), 3.83 (s, 3H, CHCOOCH3), 3.64 (m, 1H, H-2), 3.46 (m, 1H, H-5a), 3.28-2.94 (m, 3H, H-2’a, H-2’b, H-5b), 2.52-2.10 (m, 2H, H-1’a, H-1’b). 13C NMR (75.4 MHz, MeOD, δ ppm) δ 169.6, 162.0, 138.1, 131.6, 129.6, 129.1, 128.6, 117.2, 71.9, 71.3, 71.2, 64.2, 60.4, 49.9-48.2, 25.0. CIHRMS m/z found 401.2068, calcd. for C22H29N2O5 (M+H)+: 401.2076.
(2S,3R,4S) 2-{{{(1R)-1-Methoxycarbonyl-1-{4-[(prop-2-enyloxy)phenyl]}methyl}amino}ethyl}pyrrolidine-3,4-diol (17d)
Compound 17d (·HCl) was obtained in quantitative yield. [α]D25 -36.9 (c 1.05, MeOH). IR νmax 3357, 2948, 1742, 1610, 1229, 1182, 1130, 1134, 836, 792 cm-1. 1H NMR (300 MHz, MeOD, δ ppm, J Hz) δ 7.53-7.35 (m, 2H, Ar-H), δ 7.11- 6.96 (m, 2H, Ar-H), 6.04 (m, 1H, CH=CH2), 5.38 (m, 1H, CH=CH2), 5.30-5.12 (m, 2H, CH=CH2, CHCOOCH3), 4.64-4.50 (m, 2H, CH2CH=CH2), 4.38 (m, 1H, H-4), 4.11 (m, 1H, H-3), 3.79 (s, 3H, CHCOOCH3), 3.64 (m, 1H, H-2), 3.41 (m, 1H, H-5a), 3.23-2.89 (m, 3H, H-2’a, H-2’b, H-5b), 2.48-2.09 (m, 2H, H-1’a, H-1’b). 13C NMR (75.4 MHz, MeOD, δ ppm) δ 169.7, 161.9, 134.4, 131.6, 123.0, 118.0, 117.0, 71.9, 71.4, 64.2, 60.6, 54.4, 49.9-48.2, 44.4, 25.0. CIHRMS m/z found 351.1932, calcd. for C18H27N2O5 (M+H)+: 351.1920.
(2S,3R,4S) 2-{{{(1S)-1-Methoxycarbonyl-1-[[1,1’-biphenyl]-4-yl]methyl}amino}ethyl}pyrrolidine-3,4-diol (19)
Compound 19 was obtained in quantitative yield. [α]D25 +15.3 (c 1.40, MeOH). IR νmax 3388, 2953, 1738, 1236, 1136, 759, 697 cm-1. 1H NMR (300 MHz, MeOD, δ ppm, J Hz) δ 7.84-7.76 (m, 2H, Ar-H), 7.70-7.60 (m, 4H, Ar-H), 7.53-7.44 (m, 2H, Ar-H), 7.40 (m, 1H, Ar-H), 5.38 (s, 1H, CHCOOCH3), 4.42 (m, 1H, H-4), 4.16 (m, 1H, H-3), 3.86 (s, 3H, CHCOOCH3), 3.69 (m, 1H, H-2), 3.46 (m, 1H, H-5a), 3.25 (m, 1H, H-2’a), 3.19-3.03 (m, 2H, H-2’b, H-5b), 2.57-2.14 (m, 2H, H-1’a, H-1’b). 13C NMR (75.4 MHz, MeOD, δ ppm) δ 169.5, 144.9, 140.9, 130.6, 130.1, 130.0, 129.9, 129.3, 128.1, 71.8, 71.3, 64.0, 60.3, 54.3, 49.9-48.2, 44.2, 24.8. CIHRMS m/z found 371.1966, calcd. for C21H27N2O4 (M+H)+: 371.1971.
(2S,3R,4S) 2-{{{(1S)-1-Methoxycarbonyl-1-[(4’-(trifluoromethyl)-[1,1’-biphenyl]-4-yl]methyl}amino}ethyl}pyrrolidine-3,4-diol (15)
Compound 15 was obtained in quantitative yield. [α]D25 +0.9 (c 0.87, MeOH). IR νmax 3387, 2925, 1743, 1325, 1166, 1110, 824 cm-1. 1H NMR (300 MHz, MeOD, δ ppm, J Hz) δ 7.95-7.49 (m, 8H, Ar-H), 5.41 (s, 1H, CHCOOCH3), 4.20 (m, 1H, H-4), 4.00 (m, 1H, H-3), 3.84 (s, 3H, CHCOOCH3), 3.61-3.40 (m, 2H, H-2, H-5a), 3.28-3.05 (m, 3H, H-2’a, H-2’b, H-5b), 2.45-2.20 (m, 2H, H-1’a, H-1’b). 13C NMR (75.4 MHz, MeOD, δ ppm) δ 169.4, 143.2, 131.1, 130.9, 129.7, 128.9, 127.0, 77.1, 70.5, 64.2, 58.9, 51.5, 49.9-48.2, 44.7, 28.1. CIHRMS m/z found 439.1857, calcd. for C22H26F3N2O4 (M+H)+: 439.1845.
General procedure for the preparation of compounds 8 (or 9) from compounds 16 (or 18).
Ester 16 (0.30 mmol) was added portionwise to a cooled suspension (-15 °C) of LiAlH4 (25 mg, 0.74 mmol) in anhydrous THF (8.0 mL). The solution was stirred at rt for 15 min, then H2O was added dropwise (10 mL) and the mixture was extracted with AcOEt (3x30 mL). After solvent evaporation in vacuo and flash column chromatography on silica gel (CH2Cl2:MeOH 20:1), Boc-protected alcohol was obtained, that was subsequently deprotected. The above protected alcohol (0.1 mmol) was then dissolved in a mixture of HCl(4M):THF 2:1 (4.7 mL) and stirred at rt overnight. Evaporation of the solvent quantitatively afforded 8 (·HCl).
The following compounds were obtained:
(2S,3R,4S) 2-{{{(1R)-2-Hydroxy-1-[[1,1’-biphenyl]-4-yl]ethyl}amino}ethyl}pyrolidine-3,4-diol (8a)
Reduction of 16a afforded protected alcohol (2S,3R,4S) N-tert-butoxycarbonyl-2-{{{(1R)-2-hydroxy-1-[[1,1’-biphenyl]-4-l]ethyl}amino}ethyl}-3,4-O-isopropylidenepyrrolidine-3,4-diol as a colourless oil (56.5 mg, 39% yield). [α]D25 +25.8 (c 0.74, CH2Cl2). IR νmax 3329, 2971, 1689, 1081, 840, 699 cm-1. 1H NMR (300 MHz, CDCl3, δ ppm, J Hz, mixture of rotamers) δ 7.60-7.53 (m, 4H, Ar-H), 7.47-7.30 (m, 5H, Ar-H), 4.74-4.62 (m, 2H, H-3, H-4), 4.05 (m, 1H, H-2), 3.90-3.72 (m, 3H, H-5a, CH2OH), 3.63 (m, 1H, CHCH2OH), 3.24 (m, 1H, H-5b), 2.89-2.45 (m, 4H, H-2’a, H-2’b, N-H, O-H), 2.01-1.83 (m, 2H, H-1’a, H-1’b), 1.48 (m, 3H, C(CH3)2), 1.41 (m, 9H, C(CH3)3), 1.31 (m, 3H, C(CH3)2). 13C NMR (75.4 MHz, CDCl3, δ ppm) δ 154.5, 140.8, 140.6, 137.3, 128.8, 127.8, 127.4, 127.3, 127.1, 112.9, 80.1, 80.0, 77.9, 66.4, 64.4, 57.4, 50.1, 44.3, 29.7, 28.4, 26.5, 25.1. CIHRMS m/z found 483.2863, calcd. for C28H39N2O5 (M+H)+: 483.2859.
Subsequent deprotection of the above protected alcohol afforded 8a (·HCl) in quantitative yield as a white solid.[α]D25 +1.1 (c 0.79, MeOH). IR νmax 3342, 2926, 1132, 1061, 1038, 839, 767, 694 cm-1. 1H NMR (300 MHz, MeOD, δ ppm, J Hz) δ 7.81-7.74 (m, 2H, Ar-H), δ 7.71-7.63 (m, 4H, Ar-H), 7.53-7.46 (m, 2H, Ar-H), 7.40 (m, 1H, Ar-H), 4.49 (t, 1H, J = 6.0, CHCH2OH), 4.40 (m, 1H, H-4), 4.12 (m, 1H, H-3), 4.09-3.98 (m, 2H, CH2OH), 3.67 (m, 1H, H- 2), 3.48 (m, 1H, H-5a), 3.29-2.99 (m, 3H, H-2’a, H-2’b, H-5b), 2.54-2.13 (m, 2H, H-1’a, H-1’b). 13C NMR (75.4 MHz, MeOD, δ ppm) δ 144.0, 141.2, 132.8, 130.2, 129.0, 128.0, 71.8, 71.3, 65.2, 63.3, 60.5, 49.9-48.2, 43.8, 24.9. CIHRMS m/z found 343.2021, calcd. for C20H27N2O3 (M+H)+: 343.2022.
(2S,3R,4S) 2-{{{(1R)-2-Hydroxy-1-[(4’-(trifluoromethyl)-[1,1’-biphenyl]-4-yl]ethyl}amino}ethyl}pyrolidine-3,4-diol (8b)
Reduction of 16b afforded protected alcohol (2S,3R,4S) N-tert-butoxycarbonyl-2-{{{(1R)-2-hydroxy-1-[(4’-(trifluoromethyl)-[1,1’-biphenyl]-4-yl]ethyl}amino}ethyl}-3,4-O-isopropylidenepyrrolidine-3,4-diol (0.057 g, 35% yield). [α]D25 +7.1 (c 1.18, CH2Cl2). IR νmax 3441, 2987, 1671, 1070, 824, 730 cm-1. 1H NMR (300 MHz, CDCl3, δ ppm, J Hz, mixture of rotamers) δ 7.72-7.63 (m, 4H, Ar-H), 7.60-7.53 (m, 2H, Ar-H), 7.46-7.38 (m, 2H, Ar-H), 4.74-4.65 (m, 2H, H-3, H-4), 4.05 (m, 1H, H-2), 3.90-3.71 (m, 3H, H-5a, CH2OH), 3.60 (m, 1H, CHCH2OH), 3.23 (m, 1H, H-5b), 2.80-2.52 (m, 4H, H-2’a, H-2’b, N-H, O-H), 1.99-1.82 (m, 2H, H-1’a, H-1’b), 1.48 (m, 3H, C(CH3)2), 1.41 (m, 9H, C(CH3)3), 1.31 (m, 3H, C(CH3)2). 13C NMR (75.4 MHz, CDCl3, δ ppm) δ 154.5, 144.3, 140.8, 140.6, 139.0, 128.0, 127.5, 126.0, 125.7, 113.0, 80.0, 79.8, 78.0, 66.3, 64.5, 57.2, 49.9, 44.3, 29.9, 28.4, 26.5, 25.2. CIHRMS m/z found 551.2715, calcd. for C29H38F3N2O5 (M+H)+: 551.2733.
Subsequent deprotection of the above protected alcohol afforded 8b (·HCl) in quantitative yield. [α]D25 -2.2 (c 0.82, MeOH). IR νmax 3328, 2936, 1326, 1122, 1070, 823 cm-1. 1H NMR (300 MHz, MeOD, δ ppm, J Hz) δ 7.92-7.62 (m, 8H, Ar-H), 4.59-4.26 (m, 2H, CHCH2OH, H-4), 4.18-3.92 (m, 3H, H-3, CH2OH), 3.66 (m, 1H, H-2), 3.42 (m, 1H, H-5a), 3.25-2.89 (m, 3H, H-2’a, H-2’b, H-5b), 2.52-2.10 (m, 2H, H-1’a, H-1’b). 13C NMR (75.4 MHz, MeOD, δ ppm) δ 145.1, 142.3, 130.7, 129.4, 128.8, 127.0, 72.1, 71.6, 65.2, 63.4, 60.8, 49.9-48.2, 44.6, 25.1. CIHRMS m/z found 411.1891, calcd. for C21H26F3N2O3 (M+H)+: 411.1896.
(2S,3R,4S) 2-{{{(1R)-2-Hydroxy-1-{4-[(phenylmethoxy)phenyl]}ethyl}amino}ethyl}pyrrolidine-3,4-diol (8c)
Reduction of 16c afforded protected alcohol (2S,3R,4S)-N-tert-butoxycarbonyl-2-{{{(1R)-2-hydroxy-1-{4-[(phenylmethoxy)phenyl]}ethyl}amino}ethyl}-3,4-O-isopropylidenepyrrolidine-3,4-diol (0.06 g, 41% yield). [α]D25 +4.3 (c 0.79, CH2Cl2). IR νmax 3345, 2972, 1689, 1082, 829, 696 cm-1. 1H NMR (300 MHz, CDCl3, δ ppm, J Hz, mixture of rotamers) δ 7.48-7.31 (m, 5H, Ar-H), 7.28 (d, 2H, J = 8.1, Ar-H), 6.96 (d, 2H, J = 8.1, Ar-H), 5.05 (s, 2H, O-CH2-Ph), 4.75-4.63 (m, 2H, H-3, H-4), 4.13-3.62 (m, 7H, H-5a, H-2, CHCH2OH, N-H, CH2OH, O-H), 3.33 (m, 1H, H-5b), 2.81-2.55 (m, 2H, H-2’a, H-2’b), 2.08-1.83 (m, 2H, H-1’a, H-1’b), 1.47 (m, 3H, C(CH3)2), 1.41 (s, 9H, C(CH3)3), 1.31 (m, 3H, C(CH3)2). 13C NMR (75.4 MHz, CDCl3, δ ppm) δ 158.6, 154.6, 136.9, 130.8, 128.7, 128.6, 128.0, 127.5, 115.1, 113.1, 80.2, 79.8, 78.0, 70.1, 65.7, 64.3, 57.1, 50.0, 44.0, 29.7, 28.4, 26.5, 25.1. CIHRMS m/z found 513.2954, calcd. for C29H41N2O6 (M+H)+: 513.2965.
Subsequent deprotection of the above protected alcohol afforded 8c (·HCl in quantitative yield. [α]D25 -4.8 (c 0.72, MeOH). IR νmax 3339, 2931, 1611, 1515, 1454, 1248, 1132, 1024, 834, 737, 696 cm-1. 1H NMR (300 MHz, MeOD, δ ppm, J Hz) δ 7.48-7.27 (m, 5H, Ar-H), 7.28 (d, 2H, J = 8.1, Ar-H), 6.96 (d, 2H, J = 8.1, Ar-H), 5.12 (s, 2H, O-CH2-Ph), 4.43-4.24 (m, 2H, H-3, H-4), 4.08 (br s, 1H, CHCH2OH), 3.95 (br s, 2H, CH2OH), 3,60 (br s, 1H, H-2), 3.41 (br s, 1H, H-5a), 3.21-2.89 (m, 3H, H-5b, H-2’a, H-2’b), 2.45-2.08 (m, 2H, H-1’a, H-1’b). 13C NMR (75.4 MHz, MeOD, δ ppm) δ 161.4, 138.3, 131.1, 129.6, 129.0, 128.6, 116.8, 71.8, 71.3, 71.1, 65.1, 63.3, 60.6, 49.8-48.1 (under MeOD), 43.7, 24.9. CIHRMS m/z found 373.2112, calcd. for C21H29N2O4 (M+H)+: 373.2127.
(2S,3R,4S) 2-{{{(1R)-2-Hydroxy-1-{4-[(prop-2-enyloxy)phenyl]}ethyl}amino}ethyl}pyrrolidine-3,4-diol (8d)
Reduction of 16d afforded protected alcohol (2S,3R,4S) N-tert-butoxycarbonyl-2-{{{(1R)-2-hydroxy-1-{4-[(prop-2-enyloxy)phenyl]}ethyl}amino}ethyl}-3,4-O-isopropylidenepyrrolidine-3,4-diol (0.08 g, 56% yield). [α]D25 +2.4 (c 1.05, CH2Cl2). IR νmax 3442, 2979, 2931, 1688, 1610, 1083, 830, 731 cm-1. 1H NMR (300 MHz, CDCl3, δ ppm, J Hz) δ 7.20 (d, 2H, J = 8.8, Ar-H), 6.87 (d, 2H, J = 8.8, Ar-H), 6.04 (m, 1H, CH2=CHCH2O), 5.40 (dq, 1H, J = 17.3, J = 1.6, CH2=CHCH2O), 5.27 (dq, 1H, J = 10.5, J = 1.5, CH2=CHCH2O), 4.72-4.62 (m, 2H, H-3, H-4), 4.45 (dt, 2H, J = 5.4, J = 1.5, CH2=CHCH2O), 4.02 (m, 1H, H-2), 3.83 (m, 1H, H-5a), 3.77-3.63 (m, 2H, CH2OH, CHCH2OH), 3.54 (m, 1H, CH2OH), 3.22 (m, 1H, H-5b), 2.77-2.46 (m, 4H, H-2’a, H-2’b, N-H, O-H), 1.97-1.80 (m, 2H, H-1’a, H-1’b), 1.47 (s, 3H, C(CH3)2), 1.41 (s, 9H, C(CH3)3), 1.30 (s, 3H, C(CH3)2). 13C NMR (75.4 MHz, CDCl3, δ ppm) δ 158.1, 154.4, 133.3, 132.5, 128.3, 117.7, 114.8, 113.0, 80.0, 79.8, 77.9, 68.9, 66.3, 64.2, 57.3, 49.9, 44.2, 29.6, 28.4, 26.5, 25.1. CIHRMS m/z found 463.2797, calcd. for C25H39N2O6 (M+H)+: 463.2808.
Subsequent deprotection of the above protected alcohol (0.1 mmol) afforded 8d (·HCl) in quantitative yield. [α]D25 -13.2 (c 0.98, MeOH). IR νmax 3343, 2940, 1610, 1132, 1054, 1018, 833 cm-1. 1H NMR (300 MHz, MeOD, δ ppm, J Hz) δ 7.49 (m, 2H, J = 8.6, Ar-H), 7.04 (m, 2H, J = 8.6, Ar-H), 6.08 (m, 1H, CH=CH2), 5.41 (dd, 1H, J = 17.4, J = 1.7, CH=CH2), 5.27 (dd, 1H, J = 10.5, J = 1.6, CH=CH2), 4.59 (dt, 2H, J = 5.2, J = 1.5, CH2CH=CH2), 4.44-4.30 (m, 2H, H-4, CHCH2OH), 4.09 (m, 1H, H-3), 3.97 (d, 2H, J = 6.0, CH2OH), 3.62 (m, 1H, H-2), 3.43 (m, 1H, H-5a), 3.20-2.92 (m, 3H, H-2’a, H-2’b, H-5b), 2.46-2.14 (m, 2H, H-1’a, H-1’b). 13C NMR (75.4 MHz, MeOD, δ ppm) δ 161.2, 134.6, 131.0, 125.8, 117.8, 116.6, 71.8, 71.3, 69.9, 65.0, 63.3, 60.5, 49.9-48.2, 43.5, 24.9. CIHRMS m/z found 323.1965, calcd. for C17H27N2O4 (M+H)+: 323.1971.
(2S,3R,4S) 2-{{{(1S)-2-Hydroxy-1-[[1,1’-biphenyl]-4-yl]ethyl}amino}ethyl}pyrolidine-3,4-diol (9)
Reduction of 18 afforded protected alcohol (2S,3R,4S) N-tert-butoxycarbonyl-2-{{{(1S)-2-hydroxy-1-[[1,1’-biphenyl]-4-l]ethyl}amino}ethyl}-3,4-O-isopropylidenepyrrolidine-3,4-diol (0.060 g, 40% yield). [α]D25 +34.2 (c 0.84, CH2Cl2). IR νmax 3408, 2979, 1684, 1083, 839, 698 cm-1. 1H NMR (300 MHz, CDCl3, δ ppm, J Hz, mixture of rotamers) δ 7.60-7.52 (m, 4H, Ar-H), 7.48-7.30 (m, 5H, Ar-H), 4.74-4.62 (m, 2H, H-3, H-4), 4.04 (m, 1H, H-2), 3.90-3.72 (m, 3H, H-5a, CH2OH), 3.62 (m, 1H, CHCH2OH), 3.24 (m, 1H, H-5b), 2.82-2.53 (m, 4H, H-2’a, H-2’b, N-H, O-H), 2.01-1.83 (m, 2H, H-1’a, H-1’b), 1.48 (m, 3H, C(CH3)2), 1.41 (s, 9H, C(CH3)3), 1.31 (m, 3H, C(CH3)2). 13C NMR (75.4 MHz, CDCl3, δ ppm) δ 154.5, 140.8, 140.6, 139.5, 128.8, 127.7, 127.7, 127.4, 127.3, 113.0, 80.1, 80.1, 80.0, 66.4, 64.4, 57.4, 50.1, 44.4, 29.7, 28.4, 26.5, 25.2. CIHRMS m/z found 483.2858, calcd. for C28H39N2O5 (M+H)+: 483.2859.
Subsequent deprotection of the above protected alcohol (0.1 mmol) afforded 9 (·HCl) in quantitative yield. [α]D25 +11.1 (c 0.70, MeOH). IR νmax 3337, 2933, 1132, 1045, 1039, 838, 766, 694 cm-1. 1H NMR (300 MHz, MeOD, δ ppm, J Hz) δ 7.76-7.51 (m, 6H, Ar-H), δ 7.51-7.25 (m, 3H, Ar-H), 4.51-4.26 (m, 2H, CHCH2OH, H-4), 4.08 (m, 1H, H-3), 4.03-3.90 (m, 2H, CH2OH), 3.60 (m, 1H, H-2), 3.40 (m, 1H, H-5a), 3.23-2.86 (m, 3H, H-2’a, H-2’b, H-5b), 2.53-2.06 (m, 2H, H-1’a, H-1’b). 13C NMR (75.4 MHz, MeOD, δ ppm) δ 144.0, 141.2, 132.7, 130.4, 130.1, 129.0, 128.1, 71.9, 71.5, 65.3, 63.5, 60.7, 49.9-48.2, 44.2, 25.0. CIHRMS m/z found 343.2020, calcd. for C20H27N2O3 (M+H)+: 343.2022.
Viability assays
MiaPaCa2 or PK-9 cells were cultured in RPMI-1640 medium containing 10% FBS and antibiotics at 37 °C in 5% CO2. 3 x 105 cells/well were plated in 96-well plates and allowed to adhere overnight. Thereafter, the compounds were added at concentrations ranging from 1 μM to 1 mM in triplicate wells and viability was detected 72h later with Celltiter96 Aqueous1 (Promega). Compound EC50 was estimated using GraphPad (GraphPad Software, Inc, Lajolla, CA, USA).
ACKNOWLEDGEMENTS
We thank the European Community’s Seventh Framework Programme FP7-2007-2013 (grant HEALTH-F2-2011-256986, PANACREAS), the Ministerio de Ciencia e Innovación of Spain (CTQ2012-31247) and Junta de Andalucía (FQM 345) for financial support. We also acknowledge Erasmus Agreement EPFL-University of Seville for a fellowship, the Associazione Italiana per la Ricerca sul Cancro (AIRC grant #6108 to A.N.), the Italian Ministry of Health (project GR-2008-1135635 to A.N.) and the Fondazione Umberto Veronesi (to A.N.).
References
1. A. Helenius and M. Aebi, Science, 2001, 291, 2364. CrossRef
2. L. H. Hu, H. B. Zou, J. X. Gong, H. B. Li, L. X. Yang, W. Cheng, C. X. Zhou, H. Bai, F. Guéritte, and Y. Zhao, J. Nat. Prod., 2005, 68, 342. CrossRef
3. I. Pastuszak, R. J. Molyneaux, L. F. James, and A. D. Elbein, Biochemistry, 1990, 29, 1886; CrossRef B. Macchi, A. Minutolo, S. Grelli, F. Cardona, F. M. Cordero, A. Mastino, and A. Brandi, Glycobiology, 2010, 20, 500. CrossRef
4. T. Kino, N. Inamura, K. Nakahara, S. Kiyoto, T. Goto, H. Terano, M. Kohsaka, H. Aoki, and H. Imanaka, J. Antibiot., 1985, 38, 936; CrossRef P. M. Rudd and R. A. Dwek, Crit. Rev. Biochem. Mol. Biol., 1997, 32, 1. CrossRef
5. J. W. Dennis, S. L. White, A. M. Freer, and D. Dime, Biochem. Pharmacol., 1993, 46, 1459; CrossRef W. H. Pearson, Tetrahedron Lett., 2001, 42, 8273; CrossRef W. H. Pearson and L. Guo, Tetrahedron Lett., 2001, 42, 8267. CrossRef
6. N. Asano, K. Oseki, H. Kizu, and K. Matsui, J. Med. Chem., 1994, 37, 3701. CrossRef
7. N. Asano, T. Yamashita, K. Yasuda, K. Ikeda, H. Kizu, Y. Kameda, A. Kato, R. J. Nash, H. S. Lee, and K. S. Ryu, J. Agric. Food Chem., 2001, 49, 4208. CrossRef
8. Y. Mizushina, X. Xu, N. Asano, N. Kasai, A. Kato, M. Takemura, H. Asahara, S. Linn, F. Sugawara, H. Yoshida, and K. Sakaguchi, Biochem. Biophys. Res. Comm., 2003, 304, 78. CrossRef
9. G. W. J. Fleet, A. Karpas, R. A. Dwek, L. E. Fellows, A. S. Tyms, S. Petursson, S. K. Namgoong, N. G. Ramsden, P. W. Smith, J. C. Son, F. Wilson, D. R.Witty, G. S. Jacob, and T. W. Rademacher, FEBS Lett., 1988, 237, 128. CrossRef
10. S. Gerber-Lemaire, F. Popowycz, E. Rodríguez-Garcia, A. T. Carmona, and I. Robina, ChemBioChem, 2002, 5, 466. CrossRef
11. F. Popowycz, S. Favre, C. Schütz, P. Vogel, S. Gerber-Lemaire, and L. Juillerat-Jeanneret, J. Med. Chem., 2005, 48, 4237. CrossRef
12. F. Popowycz, S. Gerber-Lemaire, C. Schütz, and P. Vogel, Helv. Chim. Acta, 2004, 87, 800; CrossRef F. Popowycz, S. Gerber-Lemaire, E. Rodriguez-Garcia, C. Schütz, and P. Vogel, Helv. Chim. Acta, 2003, 86, 1914. CrossRef
13. C. Bello, M. Cea, G. Dal, A. Garuti, I. Rocco, G. Cirmena, E. Moran, A. Nahimana, M. A. Duchosal, F. Fruscione, P. Pronzato, F. Grossi, F. Patrone, A. Ballestrero, M. Dupuis, B. Sordat, A. Nencioni, and P. Vogel, Bioorg. Med. Chem., 2010, 18, 3320. CrossRef
14. Y. Mrestani, A. Härtl, and R. H. H. Neubert, Int. J. Pharm., 2006, 309, 67; CrossRef I. Gomez-Orellana, Exp. Opin. Drug Deliv., 2005, 2, 419; CrossRef R. T. Borchardt, Controlled Release, 1999, 62, 231. CrossRef
15. T. D. Butters, R. A. Dwek, and F. M. Platt, Curr. Top. Med. Chem., 2003, 3, 561; CrossRef T. D. Butters, R. A. Dwek, and F. M. Platt, Chem. Rev., 2000, 100, 4683; CrossRef T. Cox, R. Lachmann, C. Hollak, J. Aert, S. van Weely, M. Hrebicek, F. Platt, T. Butters, R. Dwek, C. Moyses, I. Gow, D. Elstein, and A. Zimran, The Lancet, 2000, 355, 1481. CrossRef
16. A. Mehta, N. Zitzmann, P. M. Rudd, T. M. Block, and R. A. Dwek, FEBS Lett., 1998, 430, 17. CrossRef
17. G. Godin, P. Compain, O. R. Martin, K. Ikeda, L. Yu, and N. Asano, Bioorg. Med. Chem. Lett., 2004, 14, 5991. CrossRef
18. M. Padró, J. A. Castillo, L. Gomez, J. Joglar, P. Clapés, and C. de Bolós, Glycoconj. J., 2010, 27, 277. CrossRef
19. C. Bello and P. Vogel, 2009: Novel Dihydroxypyrrolidine Derivatives as Anti-Cancer Agents PTC Int. Appl. WO2009118712, A2 20091001; C. Bello, G. Dal Bello, M. Cea, A. Nahimana, D. Aubry, A. Garuti, G. Motta, E. Moran, F. Fruscione, P. Pronzato, F. Grossi, F. Patrone, A. Ballestrero, M. Dupuis, B. Sordat, K. Zimmermann, J. Loretan, M. Wartmann, M. A. Duchosal, A. Nencioni, and P. Vogel, Bioorg. Med. Chem., 2011, 19, 7720. CrossRef
20. F. Cardona, I. Robina, and P. Vogel, J. Carbohydr. Chem., 2000, 19, 555; CrossRef A. T. Carmona, F. Popowycz, S. Gerber-Lemaire, E. Rodríguez-García, C. Schütz, P. Vogel, and I. Robina, Bioorg. Med. Chem., 2003, 11, 4897; CrossRef E. Moreno-Clavijo, A. T. Carmona, Y. Vera-Ayoso, A. J. Moreno-Vargas, C. Bello, P. Vogel, and I. Robina, Org. Biomol. Chem., 2009, 7, 1192. CrossRef
21. α-L-fucosidase from Jack beans, α-galactosidases from coffee beans, β-galactosidases from Escherichia coli and Aspergillus oryzae, α-glucosidases from yeast and from rice, amyloglucosidases from Aspergillus niger, β-glucosidases from almonds, α-mannosidases from Jack beans, β-mannosidases from snail, and β-N-acetylglucosaminidases from Jack beans.
22. IC50 of inhibitors showing good activity (higher than 80% inhibition at 1 mM) were determined.