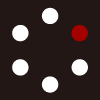
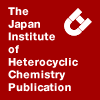
HETEROCYCLES
An International Journal for Reviews and Communications in Heterocyclic ChemistryWeb Edition ISSN: 1881-0942
Published online by The Japan Institute of Heterocyclic Chemistry
e-Journal
Full Text HTML
Received, 9th July, 2013, Accepted, 14th August, 2013, Published online, 27th August, 2013.
DOI: 10.3987/COM-13-S(S)89
■ Chemoenzymatic Approach to Synthesis of Hydroxylated Pyrrolidines from Benzoic Acid
David R. Adams, Johannes van Kempen, Jason R. Hudlicky, and Tomas Hudlicky*
Department of Chemistry, Brock University, 500 Glenridge Ave., St. Catharines, Ontario, L2R 3K4, Canada
Abstract
The fermentation of benzoic acid with R. eutrophus B9 provides the corresponding ipso-diol that serves as a convenient homochiral starting material for the synthesis of oxygenated compounds. In this paper we report the conversion of the ipso-diol to a hydroxylated pyrrolidine, which is found as a subunit in biologically active compounds. The key steps involve the enzymatic oxidation of benzoic acid, the subsequent hetero-Diels-Alder cycloaddition, and the oxidative cleavage, reductive cyclization to form the pyrrolizidine. Experimental and spectral data are provided for all new compounds.INTRODUCTION
Polyhydroxylated pyrrolidines are found as major subunits of numerous biologically active molecules and natural products.1 Structurally they are natural sugar mimics and have found clinical utility in a number of medicinally important molecules that span a range of diseases and disorders, including antidiabetic, antiviral, and anticancer activities.2-4 Hydroxylated pyrrolidines 1 and 2 have been used to prepare compounds 4 (BCX-4208) and 5 (BCX-1777) (Figure 1) and represent a class of selective immunosuppressive agents that are currently in clinical trials as inhibitors of human purine nucleoside phosphorylase (PNP). More recently a series of aza sugar mimics containing the ribose configuration has been reported as part of efforts aimed at screening for more effective hepatitis C virus (HCV) agents.5 Compounds 6 – 8 represent a series of derivatives that contain a stereo defined methyl substituent in the 2’-β position that has been deemed crucial in HCV replication assays.6
We have been involved for some time in the design and development of general methodology toward oxygenated compounds by employing a chemoenzymatic strategy depicted in Figure 2. Homochiral diols of type 9, Figure 2, are accessible via the whole-cell fermentation of aromatic compounds with the recombinant strain JM109 (pDTG601A) that was developed by Gibson7 and that over-expresses toluene dioxygenase. More than 400 of such metabolites are known8 and many have been widely used in the total synthesis of natural products.9 Our program was designed for a fully general approach to inositols,10 aminocyclitols,11 azasugars,12 pseudosugars,13 as well as their fluorinated14 or deuterated analogues,15 and extended to morphine16 and Amaryllidaceae alkaloids.17
The metabolites derived from halobenzenes are ideally suited for further functionalization, such as dihydroxylation of the distal double bond followed by oxidative cleavage-reductive cyclization, a strategy that was shown to be useful in approaches to aza sugars, as demonstrated on the synthesis of mannojirimycin 1018 and kifunensine 11,19 as shown in Figure 2. Carbohydrates containing five-membered rings have also been synthesized.20 Recently, we reported the total synthesis of idesolide21 that originated in the ipso-diol 12 available by enzymatic oxidation of benzoic acid with the mutant strain R.eutrophus B9.21 The ipso-diol is ideally suited for approaches to hydroxylated pyrrolidines containing the angular methyl, such as the recently reported pyrrolidine 3, Figure 1.5 In this report we present the synthesis of an aza sugar derivative 14b from enantiomerically pure ipso diol 12 and indicate the potential use of this compound to approaches to other five-membered polyhydroxylated pyrrolidines.
RESULTS AND DISCUSSION
While the biocatalytic dihydroxylation of halobenzenes to the cis-dihydrodiols 9, Figure 2, has found utility in many synthetic strategies8,9a,9b,10a,10c,22-58 the ipso dihydroxylation of benzoic acids using the mutant organism R. eutrophus B959 has not been exploited to the extent of usage of other mutant organisms, such as E.coli JM109 and organisms expressing other aromatic dioxygenases.60-67 The synthesis of aza-sugar 14b demonstrates the versatility of metabolite 12 as a chiral synthon.
In our initial approach to compounds 13 and 14, both the angular methyl (3, or 13a, and 14a) and the hydroxymethyl derivatives (13b and 14b), we assumed that the amino enones of type 17 could easily be cleaved oxidatively to esters of type 20 by methods such as those employed by Danishefsky in his synthesis of vernolepin.68 The well-known oxidative cleavage of enones results in the loss of the α-carbon to furnish aldehyde-esters69 and these were envisioned as intermediates for eventual transformation to pyrrolidones such as 20, Scheme 1.
After successive methylation and acetalization of the ipso carboxylic acid 12, the diene in methyl ester 15 was converted to the hetero Diels-Alder adducts 16a/b (3:1 a/b) via the cycloaddition with N-acyl nitroso dienophile generated in situ by oxidation of N-Boc hydroxyl amine with sodium periodate, Scheme 1. The isolation of individual adducts 16a/b could not be realized at this stage and the mixture of regioisomers was used in crude state after washing with aqueous base (NaHCO3). The reduction of the N-O bond with molybdenum hexacarbonyl (Mo(CO)6)70 and subsequent oxidation of the amino alcohols (not shown) with Dess-Martin periodinane (DMP) resulted in an isomeric mixture of enones 17a/b that were separable at this point by flash chromatography on silica gel. The dihydroxylation of enone 17a by the conditions reported in the literature68,69 proved troublesome, probably because of the tendency of the electron deficient olefin to oxidatively cleave under standard Upjohn71 reaction conditions (OsO4/NMO) as reported by Plietker and substantiated by the complex mixtures obtained in our experiments. The ruthenium chloride/cesium chloride/sodium periodate redox system developed by Plietker and co-workers72 established a level of oxidative control and diol 18 could be generated by this protocol. The active Ce(IV)-periodate complex was preformed by gently heating a mixture of cerium chloride heptahydrate (CeCl3·7H2O) and sodium periodate (NaIO4) in water until a yellow suspension was formed. At this point an aqueous solution of ruthenium(III) chloride was added followed by addition of substrate. The diastereomeric mixture of diols 18 was used as such, after rapid chromatographic purification, and proved to be fairly sensitive to prolonged exposure to acidic environment. Upon acylation the distal hydroxy group was eliminated to form the α,β-unsaturated intermediate 19. All attempts to oxidatively cleave diol 18 with lead tetraacetate (Pb(OAc)4) with concomitant loss of the α-carbon atom of the ketone only resulted in complex mixtures of unidentifiable material.
Having failed in the planned route to pyrrolidone 21 an alternative strategy to access pyrrolidines of type 14 was pursued. We envisioned that if acetal 27 could be prepared through a series consisting of a reduction, oxidative cleavage, and protecting group manipulations typical in sugar chemistry, that azomethine 29 could be attained and subsequently would yield pyrrolidine tetrol 14b, Scheme 2. The polar nature of many of the intermediates depicted in Scheme 2 made their isolation difficult. The acylation of compound mixtures provided the means for isolation, characterization, and protection of functionality. The initial ozonolysis of isoxazolidines 16a/b is a case in point. The ozonolysis of 16a/b in methylene chloride was rapid but the reduction of the ozonide and methyl ester functionality by addition of solid sodium borohydride (NaBH4) took several hours, even after reaching ambient temperature from -55 ˚C. The reaction mixture was quenched with water prior to concentration in vacuo and the crude ozonolysis reaction mixture was acylated using standard conditions (Ac2O, Et3N, DMAP, CH2Cl2). At this point the regioisomers were separable by silica gel chromatography and afforded the individual isoxazolidine acetates 22a and 22b in yields ranging from 22 – 37% of the desired 22a (from 16a/b). The [latent] vic-1,2-diol in 22a, which would be liberated upon the hydrolysis of acetates and the reduction of the isoxazolidine, was to be oxidatively cleaved in order to furnish acetal 27 following the cyclization onto the aldehyde generated by the cleavage of 25. To this end base hydrolysis was used to provide the corresponding isoxazolidine 23, which was characterized more easily as the fully deprotected isoxazolidine 24 upon treatment with trifluoroacetic acid (TFA). The characterization of this compound was easier than that of isoxazolidine 23, which consisted of a mixture of rotamers (Boc carbamate). Tetrol 25 was prepared from acetate 22a by hydrolysis and immediate reduction after workup of the N-O bond by Mo(CO)6. The reduction with Mo(CO)6 to yield 25 proved moderately difficult and many experiments were performed in attempts to improve the reproducibility and yields. In order to establish a moderate level of reproducibility for the reduction of the N-O bond several details had to be addressed. The purity of the Mo(CO)6 was critical and sublimation of the reagent was required to eliminate the batch variation in commercially available Mo(CO)6. Also, the in situ formation of the chemically active Mo(MeCN)3(CO)373,74 species generated from ligand exchange between acetonitrile (MeCN) and carbon monoxide (CO) had to be monitored (visible by TLC) as the reaction progressed. It was observed that the reduction of 23 could proceed much faster if the active catalyst Mo(MeCN)3(CO)3 was generated separately, isolated, and then used in subsequent reactions. However, the lack of confidence in the purity and stability of Mo(MeCN)3(CO)3 prevented its usage in the direct transformation of 23 to 25 and Mo(CO)6 was used instead. Another crucial aspect of this reaction pertained to the liberation of substrate 25 from the complexation with molybdenum. It was found that upon the completion of the reaction the mixture had to be treated with sodium bicarbonate and exposed to air with vigorous stirring for several hours (> 12 h) in order to allow for the decomplexation of the product-molybdenum species. Following this protocol led to the isolation of 25 in yields up to 87%.
The oxidative cleavage of the free vicinal diol in 25 with sodium periodate (NaIO4) in a biphasic NaHCO3(aq)/CH2Cl2 mixture provided an equilibrium mixture of a hemiacetal (not shown) and the corresponding hydroxy aldehyde isomer (as evidenced by NMR, not isolated). The oxidative cleavage of 25 was found to proceed much more smoothly and with better purity profile when a silica gel-supported periodate reagent was used.76 After filtration of the periodate/silica gel support the hemiacetal was immediately subjected to acylation and the bis acetate 27 was isolated in 41% overall yield from isoxazolidine 22a; the isolation of pure intermediates during this sequence was further detrimental to the yield. The Boc-protected amine 27 was treated with trifluoroacetic acid (TFA) to furnish 28 as a stable TFA salt. The hydrolysis of 28 under basic methanolic (anhydrous) conditions provided pyrroline 29 in 50% yield from 27. The impurity profile consisted only of potassium acetate and potassium trifluoroacetate salts and pyrroline 29 was obtained by trituration of the mixture with acetone. Hydrogenation of 29 proceeded smoothly under standard conditions (1 atm) to provide acetonide protected pyrrolidine 30 in quantitative yield. The hydrolysis of acetonide 30 proved to be sluggish at room temperature and required up to four days under these conditions to achieve full conversion. Alternatively, the use of trifluoroacetic acid in water provided pyrrolidine 14b within 24 hours but the handling and increased solubility of the TFA salt made purification more difficult than that of the corresponding crystalline HCl salt of 14b. Ultimately, treatment of acetonide 30 with HCl/MeOH at 40 ˚C provided pyrrolidine 14b as an easily crystallizable HCl salt in 75% yield.
CONCLUSIONS
We have demonstrated that the ipso-diol 12, derived enzymatically from benzoic acid, can be used for the synthesis of hydroxylated pyrrolidines by sequential hetero Diels-Alder cycloaddition followed by reductive cleavage and eventual recyclization. Future work in this area will examine the generality of this method as applicable to other targets.
ACKNOWLEDGEMENTS
We are grateful to the following agencies for financial support of this work: Natural Sciences and Engineering Research Council of Canada (NSERC) (Idea to Innovation and Discovery Grants), Canada Research Chair Program, Canada Foundation for Innovation (CFI), TDC Research, Inc., TDC Research Foundation, Brock University, and the Ontario Partnership for Innovation and Commercialization (OPIC). We also thank Professor Andrew Myers (Harvard University) and Professor Simon Lewis (University of Bath) for providing us with fresh cultures of R.eutrophus B9.
EXPERIMENTAL
All non-aqueous reactions were conducted in an argon atmosphere using standard Schlenk techniques for the exclusion of moisture and air. All solvents were distilled unless otherwise noted. Analytical thin layer chromatography was performed on EMD Silica gel 60 Å 250 μm TLC plates with F-254 indicator. Flash column chromatography was performed using Silicycle SiliaFlash P60 (230-400 mesh). Melting points were recorded on a Hoover Unimelt apparatus and are uncorrected. IR spectra were obtained on a Perkin-Elmer One FT-IR spectrometer. Optical rotation was measured on a Perkin-Elmer 341 polarimeter at a wavelength of 589 nm. H1 and C13 spectra were recorded on a 300 MHz and 600 MHz Bruker spectrometer. All chemical shift are referenced to TMS or residual non-deuterated solvent. Data for proton spectra are reported as follows: chemical shift (multiplicity [singlet (s), doublet (d), triplet (t), quartet (q) and multiplet (m)], coupling constants [Hz], integration). Carbon spectra were recorded with complete proton decoupling and the chemical shifts are reported in ppm (C) relative to TMS. Mass spectra and high resolution mass spectra were performed by the analytical division at Brock University. Combustion analyses were performed by Atlantic Microlabs, Atlanta, GA.
(1S,2R)-1,2-Dihydroxycyclohexa-3,5-diene-1-carboxylic acid (12).
The whole-cell biotransformation of benzoic acid was performed based on a modified procedure established by Mihovilovic and co-workers.63 LB(II) medium (100 mL) was inoculated with a single colony of Ralstonia eutrophus B9 that was grown on LB(II) agar plates at 30 ˚C for two days. The inoculated medium was incubated at 30 ˚C on an orbital shaker at 185 RPM until OD256 = 4.8 (1:10 dilution; ~ 24 h). At this point the cellular suspension (80 mL) was used as a preculture and added to a 15 L Sartorius Biostat C bioreactor that contained HMB medium (8.4 L) at pH = 7.4, aerated with sterile air at 3 L/min, agitation speed of 300 RPM, and D-fructose (50 mL of a 1.5 M aq. solution) concentration of 0.009 M. The culture was grown until an OD256 = 2.8 (1:10 dilution) was achieved (~ 20 h) and then induced with sodium benzoate (12 mL of a 1.5 M aq. solution; 18 mmol) and D-fructose (53 mL of a 1.5 M aq. solution; 80 mmol). After 6 h consumption of benzoate was observed by UV analysis (265 nm) and a repetitive feeding program was initiated at which 15 min feeding of an aq. solution of sodium benzoate (22 mL, 1.5 M; 1.5 mL/min feed rate) and aq. D-fructose (22 mL, 1.5 M; 1.5 mL/min feed rate) was performed every 3 h over the course of 4 days; a total of approximately 170 g of sodium benzoate was fed. After the feeding regime was completed the broth was drained and separated from cell matter by centrifugation at 5 ˚C (10,000 RPM). The dark brown ferment broth was concentrated in vacuo at 35 ˚C to dryness to obtain several lots of ipso diene diol carboxylate as the mixed potassium/sodium salts, was contaminated with other inorganic salts. NMR spectroscopy assay was used to establish a weight-weight percentage of the crude material with an internal standard (potassium benzoate). A total mass of 261.5 g of crude material was obtained and corresponded to 177 g of the salt of ipso diol 12 that could be stored at room temperature without any observable degradation (several months). 1H NMR (600 MHz, D2O) δ 6.03 (dd, J = 9.5, 5.2 Hz, 1H), 5.93 – 5.81 (m, 1H), 5.71 – 5.64 (m, 2H), 4.77 (s, 1H).75 The free acid 12 was obtained by the acidification of a cold aqueous solution of the salts with 6M HCl and extraction with EtOAc.
12: 1H NMR (300 MHz, D2O) δ 6.12 (dd, J = 9.3, 5.1 Hz, 1H), 6.05 – 5.90 (m, 1H), 5.77 (d, J = 9.6 Hz, 2H), 4.86 (s, 1H).
(3aS,7aR)-Methyl 2,2-dimethyl-3a,7a-dihydrobenzo[d][1,3]dioxole-3a-carboxylate (15).
To a slurry of mixed sodium/potassium carboxylate of 12 (61.6 g (81.1 g at 76 wt% purity), 317 mmol) in 2,2-dimethoxypropane (790 mL, 0.4 m) at 0 ˚C with vigorous stirring was added trifluoroacetic acid (146 mL, 1.90 mol, 6.0 equiv.) dropwise over 6 min while the internal temperature was monitored; no significant exotherm observed. The reaction mixture was warmed up to room temperature and stirred until the consumption of 12 was observed by TLC (4:1 EtOAc/MeOH) and/or HPLC analysis (254 nm) at approximately 6 h. The reaction mixture was filtered over a pad of Celite and the filtrate concentrated in vacuo to a dark brown/black oil. The crude oily mixture was dissolved in H2O (100 mL) and extracted twice with CH2Cl2. The organic phase was then extracted with an aqueous NaHCO3 solution (sat’d, 3 x 100 mL) and concentrated to dryness in vacuo to provide, as a solid, the sodium salt of (3aS,7aR)-2,2-Dimethyl-3a,7a-dihydrobenzo[d][1,3]dioxole-3a-carboxylate, contaminated with other inorganic salts. The crude product was then dissolved in MeOH and the heterogeneous mixture containing undissolved inorganic salts was filtered. The filtrate was concentrated in vacuo to yield 62.08 g (90%) of sodium salt acetonide as a slightly off-white solid. The storage of the sodium carboxylate was convenient in that its shelf life at room temperature exceeded several months with no loss in purity as indicated by NMR analysis.
(3aS,7aR)-2,2-Dimethyl-3a,7a-dihydrobenzo[d][1,3]dioxole-3a-carboxylate, sodium salt: 1H NMR (300 MHz, D2O) δ 6.13 (dd, J = 5.6, 9.5 Hz, 1H), 6.06 (dd, J = 5.5, 9.6 Hz, 1H), 5.92 (dd, J = 4.3, 9.5 Hz, 1H), 5.71 (dd, J = 9.9, 4.5 Hz, 1H), 4.80 (d, J = 4.2 Hz, 1H), 1.33 (s, 3H), 1.33 (s, 3H).
The corresponding free acid was obtained by the acidification of a cold aqueous solution of the salts with 6M HCl and extraction with EtOAc.
(3aS,7aR)-2,2-Dimethyl-3a,7a-dihydrobenzo[d][1,3]dioxole-3a-carboxylic acid: 1H NMR (300 MHz, CDCl3) δ 10.23 (bs, 1H), 6.16 (m, J = 3.6 Hz, 1H), 6.04 (dd, J = 4.7, 9.6 Hz, 1H), 5.77 (q, J = 3.2 Hz, 1H), 4.93 (d, J = 4.2 Hz, 1H), 1.49 (s, 3H), 1.44 (s, 3H).60
To a clear light brown solution of freshly extracted (3aS,7aR)-2,2-dimethyl-3a,7a-dihydrobenzo[d][1,3]dioxole-3a-carboxylic acid (67 g, 341 mmol) in CH2Cl2 (1.7 L, 0.2 M) at 10 ˚C was added MeOH (34 mL, 853 mmol, 2.5 equiv.), dimethylaminopyridine (4.2 g, 34.1 mmol, 0.1 equiv.), and N,N’-dicyclohexylcarbodiimide (77.4 g, 375 mmol, 1.1 equiv.) under an atmosphere of argon and with vigorous stirring; almost immediately a white slurry was generated and the reaction was stirred to room temperature and monitored by TLC analysis (5:1 hexanes/EtOAc). After 12 h the reaction mixture was filtered and the filter cake rinsed with CH2Cl2 (2x). The filtrate was slightly concentrated, which allowed for additional precipitation of dicyclohexylurea and the material was filtered again. The filtrate was concentrated to dryness in vacuo and provided crude material (71.92 g) as a brown oil that was contaminated by dicyclohexylurea. The crude material was subjected to silica gel chromatography (5:1 → 4:1 hexanes/EtOAc) and yielded 42.2 g (67%) of known methyl ester 15 as a yellow oil that solidified to a low-melting, white crystalline solid at low temperature (5 ˚C).
15: Rf = 0.51 (hexanes/EtOAc 5:1); IR (film, cm-1) 3043, 2989, 2934, 2846, 1753, 1735, 1454, 1434, 1380, 1371, 1254, 1209, 1168, 1036, 885, 710 cm-1; 1H NMR (300 MHz, CDCl3) δ 6.12 (m, 2H), 6.04 (m, 1H), 5.84 (m, 1H), 4.99 (d, J = 4.1 Hz, 1H), 3.81 (s, 3H), 1.46 (s, 3H), 1.44 (s, 3H); 13C (CDCl3, 75 MHz) δ 172.18, 124.75, 124.52, 124.07, 124.01, 106.81, 79.42, 72.74, 52.95, 26.89, 25.16.60
8-tert-Butyl 3a-methyl (3aR,4R,7S,7aR)-2,2-dimethyl-7,7a-dihydro-4,7-(epoxyimino)-1,3-benzodioxole-3a,8(4H)-dicarboxylate (16a/b).
To a solution of diene ester 15 (2.72 g, 12.9 mmol) in a mixture of MeOH/H2O (4:1 v/v, 65 mL, 0.2 M) at 0 ˚C was added sodium periodate (3.60 g, 16.8 mmol, 1.3 equiv.) as a single portion. To this mixture was added a solution of t-butyl hydroxycarbamate (2.24 g, 16.8 mmol, 1.3 equiv.) in MeOH/H2O (4:1 v/v, 10 mL, 1.7 M) dropwise over several minutes. The reaction mixture became a thick slurry upon addition of the hydroxy carbamate and progress was monitored by TLC analysis (4:1 hexanes/EtOAc, CAM). Upon consumption of 15 (approximately 2.5 h) the reaction was diluted with EtOAc and the thick slurry was filtered. The filter cake was rinsed with EtOAc and the filtrate was collected and rinsed with aqueous NaHCO3 (saturated, 1x), H2O (1x), and then brine (1x). The organic phase was dried over Na2SO4, filtered and concentrated in vacuo to yield an orange oil. The crude material was chromatographed on silica gel (2:1 hexanes/EtOAc) to yield a mixture regioisomers 16a and 16b (4.32 g, 96%; 3:1 16a/16b ratio determined by NMR). The purity of the crude material was sufficient enough to use without further purification.
16a/16b: Rf = 0.34 (4:1 hexanes/EtOAc); IR (film, cm-1) ν 2984, 2954, 2940, 2253, 1745, 1709, 1458, 1437, 1382, 1372, 1263, 1213, 1160, 1110, 1088, 1061, 1017, 877, 732; 1H NMR (600 MHz, CDCl3) δ 6.53 (m, J = 4.9 Hz, 1H), 6.49 (dd, J = 2.0, 8.0 Hz, 1H), 5.17 (d, J = 4.4 Hz, 1H), 5.14 (dd, J = 1.4, 5.9 Hz, 1H), 5.09 (m, J = 2.9 Hz, 1H), 3.89 (s, 1H), 1.48 (s, 1H), 1.34 (s, 1H), 1.31 (s, 1H); 13C NMR (75 MHz, CDCl3) δ 170.9, 157.0, 131.0, 129.9, 128.7, 112.7, 82.7, 82.3, 74.4, 72.7, 53.5, 53.1, 28.1, 26.2, 26.1; MS (EI+) m/z (%) 341 (0.4), 159 (12), 158 (35), 151 (7), 143 (21), 124 (8), 109 (7), 105 (13), 93 (13), 83 (22), 73 (21), 65 (12), 61 (15), 59 (42), 58 (16), 57 (100), 56 (26), 55 (16), 45 (20), 44 (34), 43 (96), 42 (17), 41 (65); HRMS (+EI) calcd for C12H23N1O7: 341.1475. Found: 341.14759.
(3aR,7S,7aR)-Methyl-7-(tert-butoxycarbonylamino)-2,2-dimethyl-4-oxo-3a,4,7,7a-tetrahydrobenzo[d][1,3]dioxole-3a-carboxylate (17a).
To a solution of isoxazolidines 16a/b (773 mg, 2.26 mmol) in MeCN/H2O (16 mL, 0.15 M, 15:1 v/v) at 80 ˚C was added molybdenum hexacarbonyl (897 mg, 3.39 mmol, 1.5 equiv.) with stirring. The reaction was monitored by TLC analysis (CHCl3/MeOH 95:5) and deemed complete after 24 h. The reaction mixture was filtered through a plug of Celite and the filtrate concentrated to dryness in vacuo. The crude mixture of amino alcohol regioisomers (3:1 ratio established by NMR analysis) was subjected to oxidative conditions by dissolution in CH2Cl2 (23 mL, 0.1 M), chilling the solution to 0 ˚C, and a single portion addition of Dess-Martin periodinane (1.44 g, 3.39 mmol, 1.5 equiv.) with stirring. The reaction was allowed to slowly warm to ambient temperature and was monitored by TLC analysis (hexanes/EtOAc 1:1). After 23 h the reaction was quenched with aq. Na2S2O3 (sat’d, 2 mL) and diluted with CH2Cl2 (25 mL). Precipitation occurred and the solid was filtered. The organic filtrate was washed with aq. NaHCO3 (sat’d, 1 x 6 mL), brine (1 x 8 mL), and then dried over MgSO4 and filtered. The crude organic solution was concentrated in vacuo to a solid crude material (1.34 g) that was chromatographed on silica gel (two chromatographic processes) (CHCl3/MeOH/hexanes 78:4:1) to yield 17a (246 mg, 32% from 16a/b) as a yellow-orange oil and 17b (30 mg, 4% from 16a/b) as a yellow oil.
17a: Rf = 0.60 (hexanes/EtOAc 1:1); [α]D20 64.912 (c 1.0, CHCl3); IR (film, cm-1) ν 3029, 2986, 1710, 1692, 1496, 1299, 1226, 1162, 1101, 770; 1H NMR (300 MHz, CDCl3) δ 6.87 (ddd, J = 1.6, 5.0, 9.9 Hz, 1H), 6.86 (d, J = 1.7 Hz, 1H), 6.16 (d, J = 10.2 Hz, 1H), 5.41 (d, J = 9.3 Hz, 1H), 4.84 (dd, J = 5.0, 7.4 Hz, 1H), 4.43 (s, 1H), 3.83 (s, 1H), 1.44 (s, 1H), 1.34 (s, 1H); 13C NMR (75 MHz, CDCl3) δ 191.30, 168.64, 154.73, 145.76, 127.45, 111.22, 81.98, 80.59, 79.44, 77.25, 53.56, 45.41, 28.30, 27.12, 25.59; MS (+EI) m/z (%) 285 (2), 270 (4), 248 (7), 228 (9), 159 (55), 143 (7), 127 (41), 96 (9), 83 (37), 73 (21), 59 (15), 57 (100), 43 (18), 41 (18); HRMS (+EI) calcd for C16H23O7N: 341.1475. Found: 341.1481.
(3aR,7S,7aR)-Methyl-7-(tert-butoxycarbonylamino)-5,6-dihydroxy-2,2-dimethyl-4-oxohexahydrobenzo[d][1,3]dioxole-3a-carboxylate (19).
Step 1. To a solution of sodium periodate (904 mg, 4.22 mmol, 1.5 equiv.) in H2O (0.85 mL, 3.3 M) at room temperature was added cerium (III) chloride heptahydrate (104 mg, 0.281 mmol, 10 mol%) and the white slurry was gently heated so that it became yellow in color. The yellow slurry was cooled in an ice-bath and a mixture of EtOAc/MeCN (1.2:1 v/v) was added. To this chilled mixture was added ruthenium (III) chloride hydrate (RuCl3·xH2O, 0.25 mol%) and the reaction mixture immediately turned to a dark brown color. A solution of enone 17a (962 mg, 2.81 mmol, 1.0 equiv.) in EtOAc (6.5 mL, 0.43 M) was added to the reaction mixture and the reaction was stirred for approximately 23 h with gradual warming to room temperature over the first 1.5 h. The reaction was monitored by TLC analysis (EtOAc/hexanes 2:1). Once the reaction was deemed complete by TLC small portions of sodium sulfite were added until no more active oxidant was detectable by KI/starch paper. The reaction mixture was filtered and the filtered solid washed with small portions of EtOAc. The filtrate was concentrated to yield 1.00 g of crude material as a light yellow foam-like solid. Silica gel chromatography (EtOAc/hexanes 2:1) provided 647 mg of 18 (quant.).
Step 2. To a solution of reasonably pure 18 (26 mg, 0.081 mmol, 1.0 equiv.) in CH2Cl2 (0.8 mL, 0.1 M) at room temperature was added acetic anhydride (30 µL, 0.327 mmol, 4.0 equiv.), triethylamine (45 µL, 0.327 mmol, 4.0 equiv.), and dimethylaminopyridine (DMAP, cat.) in succession. Upon addition of triethylamine the clear and colorless mixture became clear and yellow and following the addition of DMAP the mixture lightened in color. The reaction was monitored by TLC analysis (hexanes/EtOAc 1:1) and starting material was consumed after 10 min. The reaction was quenched with an aqueous solution of ammonium chloride (sat’d, 0.2 mL) and followed by the addition of H2O (0.4 mL). The phases were separated and the organic phase was washed with H2O (2x), brine (1x), and then dried over MgSO4. The organic phase was concentrated in vacuo to yield 28 mg of crude material as a white foam-like solid. The crude material was chromatographed on silica gel (hexanes/EtOAc 2:1) to yield 18 mg (52%) of acetate 19 as a white solid.
19: mp 103 – 105 ˚C (pentane); Rf = 0.54 (hexane/EtOAc 2:1); [α]D20 +64.888 (c 0.90, CHCl3); IR (film, cm-1) ν 3393, 2984, 1773, 1703, 1498, 1369, 1303, 1193, 1165, 1094, 1014, 875, 755; 1H NMR (300 MHz, CDCl3) δ 6.55 (d, J = 5.6 Hz, 1H), 5.48 (d, J = 9.6 Hz, 1H), 5.03 (dd, J = 9.1, 6.0 Hz, 1H), 4.46 (s, 1H), 3.89 (s, 3H), 2.25 (s, 3H), 1.53 – 1.46 (m, 12H), 1.45 (s, 3H); 13C NMR (75 MHz, CDCl3) δ 185.24, 168.05, 154.62, 143.94, 131.53, 111.93, 83.36, 80.74, 79.52, 77.44, 77.02, 76.59, 53.75, 44.90, 28.30, 26.97, 25.64, 22.32, 20.20, 14.04; HRMS (EI+ TOF) calcd for C18H25NO9: 399.1529. Found: 399.1539.
[(3aR,4R,7S,7aR)-2,2-Dimethyldihydro-4H-[1,3]dioxolo[4,5-d][1,2]oxazine-3a,4,7-triyl]tri(methylene) triacetate (22a).
To a clear and colorless solution of isoxazolidine 16a/b (104 mg, 0.295 mmol, ~6:1 mixture of regioisomers) in CH2Cl2 (6 mL) at -70 °C was bubbled ozone until a blue color persisted (ca. 35 min) which corresponded to the consumption of 16a/b by TLC analysis (hexanes/EtOAc 2:1). Oxygen was used to purge the reaction mixture and devoid it of excess ozone and its blue color. Sodium borohydride (21 mg) was added directly to the reaction mixture at -54 °C and the reaction was slowly warmed to room temperature and allowed to stir for a total of 23 h at room temperature. To the hazy, off-white reaction mixture was added H2O (3 drops) and stirring was allowed for several minutes prior to concentration in vacuo. The crude mixture was then reconstituted in CH2Cl2 (3 mL) and acetic anhydride (140 μL, 1.47 mmol), triethylamine (205 μL, 1.47 mmol), and 4-dimethylaminopyridine (DMAP, cat.) was added in sequence and allowed to stir overnight at room temperature. After 17 h the reaction was quenched with aq. NH4Cl (sat’d, 1 mL) and the phases were separated. The organic phase was washed with H2O (2 x 1 mL) and brine (1 x 1 mL), dried over anhydrous MgSO4, and concentrated in vacuo to provide an orange oil (119 mg). The crude material was chromatographed on silica gel (hexanes/EtOAc 2:1) to yield 22a (31 mg, 22%) as a colorless oil.
22a: Rf = 0.41 (hexanes/EtOAc 2:1); [α]D20 +37.47° (c 0.7, CHCl3); IR (film, cm-1) υ 2984, 2936, 1746, 1706, 1456, 1370, 1337, 1233, 1165, 1132, 1043, 930, 885, 854, 759; 1H NMR (300 MHz, DMSO) δ 4.55 (d, J = 4.0 Hz, 1H), 4.38 – 4.23 (m, 3H), 4.20 (s, 2H), 4.14 (dd, J = 8.9, 6.6 Hz, 1H), 4.01 (dd, J = 13.0, 8.4 Hz, 1H), 3.81 (dd, J = 8.3, 2.1 Hz, 1H), 2.04 (s, 3H), 2.03 (s, 3H), 2.02 (s, 3H), 1.45 (s, 9H), 1.40 (s, 3H), 1.35 (s, 3H); 13C NMR (75 MHz, DMSO) δ 170.7, 170.5, 170.1, 155.9, 109.6, 81.9, 81.8, 78.3, 73.5, 64.8, 62.2, 62.0, 52.8, 28.3, 27.2, 26.0, 21.2, 21.1, 20.9; MS (+EI) m/z (%) 283 (M+-CH3, 1), 375 (11), 223 (21), 167 (20), 149 (100), 101 (15), 57 (87); HRMS (+EI) calcd for C21H33O11N: 475.2054. Found 475.2054.
(3aR,4R,7S,7aR)-tert-Butyl 3a,4,7-tris(hydroxymethyl)-2,2-dimethyldihydro-3aH-[1,3]dioxolo[4,5-d][1,2]oxazine-6(4H)-carboxylate (23).
To a solution of protected isoxazolidine 22a (39 mg, 0.082 mmol) in MeOH (2 mL) at room temperature was added an aqueous solution of potassium carbonate (10%, 1.3 mL) with stirring. The consumption of 22a was observed by TLC analysis (EtOAc) after 1.2 h and the reaction mixture was neutralized with aqueous HCl (1 M). The mixture was extracted with EtOAc (3 x 1.5 mL), the combined organic phases were dried over anhydrous sodium sulfate and concentrated in vacuo to yield 23 (31 mg, quant.) as a colorless oil. An analytical sample was obtained by silica gel chromatography (EtOAc).
23: Rf = 0.37 (EtOAc); [α]D20 +56.68° (c 0.7, CHCl3); IR (film, cm-1) υ 3379, 2983, 2935, 1700, 1456, 1393, 1370, 1337, 1251, 1217, 1150, 1117, 1050, 1010, 904, 866, 844, 826, 756; 1H NMR (600 MHz, DMSO) δ 5.18 (t, J = 5.2 Hz, 1H), 4.85 (t, J = 5.5 Hz, 1H), 4.52 (d, J = 3.6 Hz, 1H), 4.48 (t, J = 5.7 Hz, 1H), 3.79 (dt, J = 9.8, 4.2 Hz, 1H), 3.68 – 3.63 (m, J = 9.5, 4.7 Hz, 1H), 3.63 – 3.53 (m, 4H), 3.45 – 3.38 (m, 2H), 1.42 (s, 9H), 1.34 (s, 3H), 1.29 (s, 3H); 13C NMR (150 MHz, DMSO) δ 157.4, 108.3, 85.1, 81.1, 80.1, 74.5, 63.8, 60.4, 59.1, 57.5, 28.4, 27.5, 26.6; MS (FAB+) m/z (%) 350 (3), 294 (13), 250 (10), 192 (14), 136 (12), 109 (11), 107 (12), 97 (14), 95 (17), 57 (100), 43 (58), 41 (50), 39 (18), 29 (23); HRMS (FAB+) calcd for C15H28O8N: 350.1815 [M++1]. Found 350.1791.
tert-Butyl (S)-1-((4R,5S)-5-((R)-1,2-dihydroxyethyl)-5-(hydroxymethyl)-2,2-dimethyl-1,3-dioxolan-4-yl)-2-hydroxyethylcarbamate (25).
To a clear, light yellow and degassed solution of isoxazolidine 23 (152 mg, 0.435 mmol, 1.0 equiv.) in MeCN/H2O (4.4 mL, 0.1 M, 15:1 v/v) at room temperature was added molybdenum hexacarbonyl (sublimed, 252 mg, 0.957 mmol, 2.2 equiv.) with stirring and under a blanket of argon. The reaction mixture slowly changed from a light yellow heterogeneous mixture of Mo(CO)6 crystals and dissolved substrate to a darker homogeneous mixture and an eventual black mixture upon heating to reflux. The reaction was monitored by TLC analysis (4:1 EtOAc/MeOH, CAM) and stirred for ca. 20 h prior to cooling to room temperature and spiking the reaction mixture with solid NaHCO3 (two small spatula tips) and several drops of an aqueous NaHCO3 (sat’d) solution and exposing to air for > 8 h. Celite (small scoop) was added to the reaction mixture with stirring and then passed over a short pad of Celite/SiO2 (1:1 w/w) and eluted with EtOAc. Three fractions were collected and the main fraction that contained 25 was concentrated in vacuo to yield 133 mg (87%) of 25 as a slightly pink crude material that was used without further purification.
25: mp 61 – 63 ˚C; Rf = 0.58 (EtOAc/MeOH 4:1); [α]D20 +2.1 (c 1.5, CHCl3); IR (film, cm-1) ν 3418, 2982, 2936, 1691, 1507, 1456, 1384, 1252, 1218, 1168, 1053, 894, 865, 615; 1H NMR (300 MHz, MeOD) δ 4.38 (d, J = 4.6 Hz, 1H), 4.11 (dd, J = 11.0, 5.3 Hz, 1H), 3.88 (dd, J = 7.3, 3.5 Hz, 1H), 3.81 – 3.72 (m, 1H), 3.72 – 3.57 (m, 5H), 1.51 (s, 3H), 1.47 (s, 8H), 1.40 (s, 3H); 13C NMR (75 MHz, MeOD) δ 156.7, 107.5, 84.2, 79.0, 78.4, 71.6, 63.6, 62.3, 62.2, 51.0, 27.3, 25.8, 25.1; MS (FAB+) m/z (%) 353 (12), 352 (67.5), 296 (17), 253 (12), 252 (99), 238 (15), 214 (21), 194 (33), 176 (10), 149 (29), 113 (12), 57 (100), 55 (13), 43 (28), 41 (24), 29 (12); HRMS (FAB+) calcd for C15H30NO8: 352.1971 [M+1]. Found 352.1976; Anal. Calcd for C15H29O8N: C, 51.27; H, 8.32. Found C, 51.37; H, 8.16.
((4S,5R)-5-((S)-2-Acetoxy-1-(tert-butoxycarbonylamino)ethyl)-4-((R)-2-acetoxy-1-hydroxyethyl)-2,2-dimethyl-1,3-dioxolan-4-yl)methyl acetate (26).
To a clear and colorless solution of isoxazolidine triol 23 (52 mg, 0.148 mmol) in a mixture of MeCN/H2O (1.5 mL, 15:1 v/v) at room temperature was added molybdenum hexacarbonyl (58 mg, 0.223 mmol) with stirring. The reaction was heated to reflux for ca. 24 h prior to an additional portion of molybdenum hexacarbonyl (39 mg, 0.148 mmol) was added. The reaction was stirred at reflux for another 12 h prior to cooling to room temperature and filtration through a pad of Celite. The filtrate was concentrated in vacuo to yield a crude black mass that was dissolved in CH2Cl2 (2.5 mL) and acetic anhydride (140 μL, 1.48 mmol), Et3N (206 μL, 1.48 mmol), and 4-dimethylaminopyridine (cat.) was added sequentially at room temperature and with stirring. The reaction was stirred for 20 h and quenched with aq. NH4Cl (sat’d, 0.5 mL). The phases were separated and the organic phase was washed with H2O (2x) and brine (1x). The organic phase was dried over MgSO4 and concentrated to provide crude material (25 mg) that was chromatographed on silica gel (hexanes/EtOAc 1:1) to yield 26 (6 mg, 8%) as a white foam.
26: Rf = 0.53 (hexanes/EtOAc 1:1); IR (film, cm-1) υ 3368, 2981, 2930, 1747, 1715, 1497, 1454, 1369, 1226, 1165, 1046, 870, 603; 1H NMR (300 MHz, CDCl3) δ 5.39 (dd, J = 7.2, 3.4 Hz, 1H), 4.96 (d, J = 9.0 Hz, 1H), 4.34 – 4.23 (m, 2H), 4.21 (d, J = 4.2 Hz, 2H), 4.18 – 4.05 (m, 3H), 2.13 (s, 3H), 2.09 (s, 3H), 2.05 (s, 3H), 1.99 (s, 3H), 1.50 (s, 3H), 1.46 (s, 8H), 1.36 (s, 3H); MS (EI+) m/z (%) 448 (2), 404 (3), 346 (10), 287 (14), 259 (15), 149 (11), 102 (36), 57 (64), 43 (100); HRMS (EI+) calcd for C22H34O12N: 504.2081 [M+-CH3]. Found 504.2084.
((3aR,7S,7aR)-4-Acetoxy-7-(tert-butoxycarbonylamino)-2,2-dimethyltetrahydro-3aH-[1,3]dioxolo[4,5-c]pyran-3a-yl)methyl acetate (27).
To a clear and red solution of tetrol 25 (2.37 g, 6.74 mmol; 3.70 g 25 with 64.1% w/w purity) in CH2Cl2 (70 mL, 0.1 M) at 0 ˚C was added a freshly prepared quantity of silica gel support NaIO4 (15% w/w; 13.5 g, 9.43 mmol, 1.4 equiv.)76 over 2 min; the color of the slurry immediately changed from red to light yellow and a small exotherm was detected. The reaction was monitored by TLC analysis (EtOAc, CAM) and 25 was consumed after 0.5 h. The reaction mixture was filtered and the solid support was rinsed thoroughly with CH2Cl2 and EtOAc until no chemical species was detectable by TLC [Rf = 0.61 (EtOAc)]. The clear and yellow filtrate was dried over Na2SO4 and quickly concentrated in vacuo prior to reconstitution in CH2Cl2 (70 mL, 0.1 M) and addition of acetic anhydride (4.5 mL, 47.1 mmol, 7 equiv.), triethylamine (6.6 mL, 47.1 mmol, 7 equiv.), and a catalytic amount of dimethylaminopyridine with stirring. The acylation was monitored by TLC analysis (EtOAc, CAM) and the intermediate lactol (Rf = 0.61) was consumed within 1 h. An aqueous solution of NH4Cl (half sat’d, 25 mL) was added to the chilled (ice bath) reaction mixture. After warming to ambient temperature the phases were separated and the organic phase was washed with H2O (1x), brine (1x), dried over Na2SO4, filtered and then concentrated in vacuo to an orange oil (4.03 g). The crude material was chromatographed on silica gel (3:1 → 2:1 hexanes/EtOAc) to yield 1.53 g (56%) of acetal 27 as a hygroscopic white foam.
27: mp (pentane) 53 – 56 ˚C; Rf = 0.39 (2:1 hexanes/EtOAc); IR (film, cm-1) υ 3362, 2983, 2934, 1764, 1747, 1711, 1511, 1454, 1382, 1366, 1305, 1240, 1218, 1171, 1097, 1053, 1006, 899, 872, 757, 735; 1H NMR (300 MHz, CDCl3) δ 5.69 (s, 1H), 5.27 (d, J = 7.8 Hz, 1H), 4.48 – 4.31 (m, J = 4.0, 2.0 Hz, 3H), 4.09 (d, J = 12.1 Hz, 1H), 4.03 (s, 1H), 3.86 (d, J = 12.2 Hz, 1H), 2.15 (s, 3H), 2.13 (s, 3H), 1.53 (s, 3H), 1.46 (s, 8H), 1.41 (s, 3H); 13C NMR (75 MHz, CDCl3) δ 170.1, 169.0, 154.9, 109.7, 95.5, 80.05, 66.6, 61.5, 46.8, 28.3, 27.8, 26.3, 20.9, 20.8; MS (EI+) m/z (%) 403 (< 1), 272 (19), 227 (20), 184 (18), 113 (45), 101 (12), 88 (29), 57 (76), 43 (100); HRMS (EI+) calcd for C18H29O9N: 403.1842 [M+]. Found 403.1842. Anal. Calcd for C18H29O9N: C, 53.59; H, 7.25. Found C, 53.68; H, 7.26.
((3aR,7S,7aR)-4-Acetoxy-7-amino-2,2-dimethyltetrahydro-3aH-[1,3]dioxolo[4,5-c]pyran-3a-yl)-methyl acetate (28).
To a flamed dried flask a solution of acetal 27 (33 mg, 0.081 mmol) in CH2Cl2 (2 mL) at 0 ˚C was added trifluoroacetic acid (0.5 mL) as a single portion with stirring and under a blanket of argon. The reaction was allowed to warm to room temperature and monitored by TLC analysis (EtOAc/MeOH 4:1 (or) hexanes/EtOAc 2:1). The reaction was deemed complete after 50 min and concentrated in vacuo to provide crude material (31 mg) that was passed through a plug of deactivated (20% wt/wt H2O) silica gel and eluted with EtOAc. The mother liquor was concentrated to yield 15 mg (62%) of amine salt 28 as a white crystalline solid.
28: mp 163 – 165 ˚C (Et2O); Rf = 0.13 (ethyl acetate); [α]D20 -17.890 (c 0.60, CHCl3); IR (KBr, cm-1) ν 3369, 2991, 2939, 1769, 1746, 1678, 1376, 1212, 1137, 1063, 907, 836, 799, 721, 665; 1H NMR (300 MHz, CDCl3) δ 5.85 (bs, 2H), 5.68 (s, 1H), 4.68 (d, J = 12.8 Hz, 1H), 4.44 (s, 1H), 4.36 (d, J = 12.8 Hz, 1H), 4.20 – 3.90 (m, 2H), 3.60 (s, 1H), 2.15 (s, 2H), 2.14 (s, 2H), 1.54 (s, 3H), 1.42 (s, 3H); 13C NMR (75 MHz, CDCl3) δ 171.18, 168.91, 110.07, 110.07, 95.45, 95.45, 77.82, 77.82, 77.43, 77.01, 76.59, 75.59, 65.56, 60.79, 47.59, 47.59, 27.75, 27.75, 26.08, 26.08, 20.77, 20.77, 20.70, 20.70; Anal. Calcd for C15H22F3NO9: C, 43.17; H, 5.31. Found C, 42.92; H, 5.34.
((3aR,6S,6aR)-2,2-Dimethyl-6,6a-dihydro-3aH-[1,3]dioxolo[4,5-c]pyrrole-3a,6-diyl)dimethanol (29). To a flamed dried vessel that contained vacuum dried solid K2CO3 (31 mg, 0.229 mmol, 4.0 equiv.) was added a solution of trifluoroacetate salt 28 (23 mg, 0.057 mmol, 1.0 equiv.) in anhydrous MeOH-d4 (0.50 mL, 0.11 M) with stirring. The reaction was stirred for 5.5 h at room temperature and was monitored by 1H NMR [Note: Rf is identical to that of starting material 28, Rf = 0.52 (4:1 EtOAc/MeOH)]. The reaction was filtered through a pad of Celite and washed with small volumes of MeOH (2 x 0.5 mL). The filtrate was concentrated in vacuo to provide an oily white crystalline material (32 mg) that was contaminated with KOAc and CF3CO2K. The crude material could be purified by trituration with acetone several times to yield 9 mg of 29 (81%).
29: Rf = 0.52 (4:1 EtOAc/MeOH); [α]20D -24.408 (c 0.45, MeOH); IR (KBr, cm-1) ν 3306, 2987, 2930, 2846, 1626, 1572, 1454, 1373, 1240, 1215, 1182, 1090, 1049, 900, 863; 1H NMR (600 MHz, MeOH-d4) δ 4.62 (d, J = 4.1 Hz, 1H), 4.07 – 3.99 (m, 1H), 3.93 – 3.84 (m, 2H), 3.84 – 3.77 (m, 2H), 1.39 (s, 3H), 1.35 (s, 3H); 13C NMR (MeOH-d4, 150 MHz) δ 169.2, 111.9, 97.7, 80.0, 75.6, 61.4, 59.9, 26.2, 25.5; HRMS (EI+) calcd for C9H16NO4 (M+H): 202.1079. Found: 202.1071.
((3aR,6S,6aR)-2,2-Dimethyltetrahydro-3aH-[1,3]dioxolo[4,5-c]pyrrole-3a,6-diyl)dimethanol (30).
To a round bottom flask that contained crude pyrroline 29 (22 mg, 0.109 mmol, 1.0 equiv.; contaminated with KOAc/CF3CO2K) was added HOAc (1.0 mL; aqueous, 3 M) followed by 10% Pd/C (2 mg, 10 wt%) and the reaction vessel was purged with H2 (3x) and set to stir at room temperature for approximately 15 h under 1 atmosphere of hydrogen. The reaction mixture was filtered over a pad of Celite and rinsed with small amounts of H2O. The filtrate was concentrated in vacuo to yield crude material that was crystalline in nature and was triturated with acetone (precipitating any KOAc/CF3CO2K). The mother liquor was concentrated in vacuo to yield a sticky white foam, 8 mg, 30 (quantative over 2 steps from TFA salt).
30: Rf = 0.30 (4:1 EtOAc/MeOH); [α]20D +17.312 (c 0.40, MeOH); IR (KBr, cm-1) ν 3416, 2937, 1681, 1563, 1414, 1384, 1206, 1139, 1051, 803, 723; 1H NMR (600 MHz, MeOH-d4) δ 4.60 (d, J = 3.0 Hz, 1H), 3.92 (dd, J = 11.4, 5.7 Hz, 1H), 3.80 (dd, J = 11.4, 7.6 Hz, 1H), 3.77 – 3.72 (m, 2H), 3.15 (dd, J = 12.8 Hz, 2H), 1.52 (s, 3H), 1.39 (s, 3H), signal (1H) under solvent peak; 13C NMR (151 MHz, MeOH-d4) δ 111.8, 91.4, 81.8, 65.0, 63.5, 58.3, 53.3, 48.0, 47.9, 47.7, 47.6, 47.5, 47.3, 47.2, 25.8, 24.8; HRMS (ESI) calcd for C9H17NO4 (M+H): 204.1228. Found: 204.1236.
(2S,3R,4R)-3,4-Dihydroxy-2,4-bis(hydroxymethyl)pyrrolidinium chloride (14b).
To a light yellow solution of protected pyrrolidine 30 (165 mg, 0.811 mmol) in MeOH (4 mL, 0.2 M) at room temperature was added conc. HCl (0.7 mL) with stirring and under a blanket of argon. The reaction was heated to 40 ˚C and monitored by NMR. Starting acetonide 30 was consumed after approximately 5 h and the reaction mixture was concentrated in vacuo to dryness. The reaction was reconstituted in a minimal amount of MeOH and precipitated with the addition of Et2O while stirring. The slightly off-white precipitate was dried under high vacuum to yield 121 mg (75%) of pyrrolidine tetrol 14b as the hydrochloride salt. An analytical sample was obtained by recrystallization from boiling MeOH to give white crystalline solid.
14b: mp 141 – 143 ˚C (MeOH); Rf = 0.0 (EtOAc/MeOH 4:1); [α]D20 -29.881 (c 0.95, MeOH); IR (film, cm-1) ν 3214, 1607, 1415, 1322, 1251, 1196, 1119, 1031, 696, 636, 524; 1H NMR (600 MHz, MeOD) δ 4.26 (d, J = 6.7 Hz, 1H), 3.97 – 3.87 (m, 2H), 3.81 (dd, J = 12.7, 6.6 Hz, 1H), 3.61 (d, J = 11.6 Hz, 1H), 3.59 (d, J = 12.6 Hz, 1H); 13C NMR (150 MHz, MeOD) δ 78.92, 70.65, 63.37, 63.32, 58.16, 49.91;MS (FAB+) m/z (%) 223 (18), 164 (56), 131 (100), 75 (11), 61 (14), 57 (23), 43 (12), 39 (45), 29 (13); HRMS (FAB+) calcd for C12H14O4N: 164.0917. Found: 164.09301.
References
1. N. Asano, R. J. Nash, R. J. Molyneux, and G. W. J. Fleet, Tetrahedron: Asymmetry, 2000, 11, 1645. CrossRef
2. B. Andersen, N. Westergaard, and K. Lundgren, Biochem. J., 1999, 342, 545. CrossRef
3. D. Durantel, N. Branza-Nichita, S. Carrouée-Durantel, T. D. Butters, R. A. Dwek, and N. Zitzmann, J. Virol., 2001, 75, 8987. CrossRef
4. P. E. Goss, M. A. Baker, J. P. Carver, and J. W. Dennis, Clin. Cancer Res., 1995, 1, 935.
5. P. L. Kotian, A. Ghosh, T. H. Lin, M. Wu, V. Satish Kumar, Y. S. Babu, and P. Chand, Tetrahedron Lett., 2011, 52, 365. CrossRef
6. S. S. C. Olsen, Infect. Disord.: Drug Targets, 2006, 6, 17. CrossRef
7. G. J. Zylstra and D. T. Gibson, J. Biol. Chem., 1989, 264, 14940.
8. (a) R. A. Johnson, Organic Reactions, Vol. 63; John Wiley and Sons, Inc.: New York, 2004, pp. 117–264; (b) T. Hudlicky, D. Gonzalez, and D. T. Gibson, Aldrichimica Acta, 1999, 32, 35.
9. (a) T. Hudlicky and J. W. Reed, Synlett, 2009, 685; CrossRef (b) T. Hudlicky and J. W. Reed, Chem. Soc. Rev., 2009, 38, 3117. CrossRef
10. For reviews see: (a) J. Duchek, D. R. Adams, and T. Hudlicky, Chem. Rev., 2011, 111, 4223; CrossRef (b) T. Hudlicky, D. A. Entwistle, K. K. Pitzer, and A. J. Thorpe, Chem. Rev., 1996, 96, 1195; CrossRef (c) T. Hudlicky, J. W. Reed, In Advances in Asymmetric Synthesis; ed. by A. Hassner, JAI Press: London, 1995; Vol. 1, p. 271; CrossRef (d) T. Hudlicky, M. Mandel, J. Rouden, R. S. Lee, B. Bachmann, T. Dudding, K. Yost, and J. S. Merola, J. Chem. Soc., Perkin Trans. 1, 1994, 1553. CrossRef
11. T. Hudlicky, H. F. Olivo, and B. McKibben, J. Am. Chem. Soc., 1994, 116, 5108. CrossRef
12. T. Hudlicky, J. Rouden, H. Luna, and S. Allen, J. Am. Chem. Soc., 1994, 116, 5099. CrossRef
13. (a) F. Fabris, J. Collins, B. Sullivan, H. Leisch, and T. Hudlicky, Org. Biomol. Chem., 2009, 7, 2619; CrossRef (b) D. A. Entwistle and T. Hudlicky, Tetrahedron Lett., 1995, 36, 2591. CrossRef
14. (a) F. Yan, B. V. Nguyen, C. York, and T. Hudlicky, Tetrahedron, 1997, 53, 11541; CrossRef (b) B. V. Nguyen, C. York, and T. Hudlicky, Tetrahedron, 1997, 53, 8807. CrossRef
15. T. Hudlicky, K. K. Pitzer, M. R. Stabile, A. J. Thorpe, and G. M. Whited, J. Org. Chem., 1996, 61, 4151. CrossRef
16. (a) U. Rinner and T. Hudlicky, Top. Curr. Chem., 2012, 309, 33; CrossRef (b) J. Zezula and T. Hudlicky, Synlett, 2005, 388. CrossRef
17. (a) A. Kornienko and A. Evidente, Chem. Rev., 2008, 108, 1982; CrossRef (b) U. Rinner and T. Hudlicky, Synlett, 2005, 365. CrossRef
18. T. Hudlicky, J. Rouden, and H. Luna, J. Org. Chem., 1993, 58, 985. CrossRef
19. J. Rouden and T. Hudlicky, J. Chem. Soc., Perkin Trans. 1, 1993, 1095. CrossRef
20. (a) T. Hudlicky and J. D. Price, Synlett, 1990, 159; CrossRef (b) T. Hudlicky, H. Luna, J. D. Price, and F. Rulin, Tetrahedron Lett., 1989, 30, 4053. CrossRef
21. D. R. Adams, C. Aichinger, J. Collins, U. Rinner, and T. Hudlicky, Synlett, 2011, 725. CrossRef
22. D. R. Boyd, N. D. Sharma, and C. C. R. Allen, Curr. Opin. Biotechnol., 2001, 12, 564. CrossRef
23. T. Hudlicky, D. Gonzalez, and D. T. Gibson, Aldrichimica Acta, 1999, 32, 35.
24. D. R. Boyd and G. N. Sheldrake, Nat. Prod. Rep., 1998, 309. CrossRef
25. H. A. J. Carless, Tetrahedron: Asymmetry, 1992, 3, 795. CrossRef
26. D. A. Widdowson, D. W. Ribbons, and S. D. Thomas, Janssen Chim. Acta, 1990, 8, 3.
27. T. Hudlicky, R. Fan, H. Luna, H. Olivo, and J. Price, Pure Appl. Chem., 1992, 64, 1109. CrossRef
28. T. Hudlicky, R. Fan, H. Luna, H. Olivo, and J. Price, Indian J. Chem., 1993, 32B, 154.
29. T. Hudlicky, In Enzymes in Organic Synthesis; NATO ASI Series C; ed. by S. Servi, Kluwer Academic: Boston, 1992; Vol. 381, p. 123.
30. S. M. Brown and T. Hudlicky, In Organic Synthesis: Theory and Applications; ed. by T. Hudlicky, JAI Press: London, 1993; Vol. 2, p. 113.
31. T. Hudlicky, Pure Appl. Chem., 1994, 66, 2067. CrossRef
32. T. Hudlicky, D. A. Entwistle, K. K. Pitzer, and A. J. Thorpe, Chem. Rev., 1996, 96, 1195. CrossRef
33. V. de la Sovera, A. Bellomo, and D. Gonzalez, Tetrahedron Lett., 2011, 52, 430. CrossRef
34. A. Bellomo, J. B. Bonilla, J. Lopez-Prados, M. Martin-Lomas, and D. Gonzalez, Tetrahedron: Asymmetry, 2009, 20, 2061. CrossRef
35. A. Bellomo, S. Camarano, C. Rossini, and D. Gonzalez, Carbohydr. Res., 2009, 344, 44. CrossRef
36. A. Bellomo, D. Gonzalez, and H. A. Stefani, J. Organomet. Chem., 2008, 693, 1136. CrossRef
37. A. Bellomo, C. Giacomini, B. Brena, G. Seoane, and D. Gonzalez, Synth. Commun., 2007, 37, 3509. CrossRef
38. T. Hudlicky, U. Rinner, D. Gonzalez, H. Akgun, S. Schilling, P. Siengalewicz, T. A. Martinot, and G. R. Pettit, J. Org. Chem., 2002, 67, 8726. CrossRef
39. J. C. Ramos, M. Brovetto, and G. A. Seoane, Org. Lett., 2013, 15, 1982. CrossRef
40. G. Fonseca and G. A. Seoane, Tetrahedron: Asymmetry, 2005, 16, 1393. CrossRef
41. I. Carrera, M. C. Brovetto, and G. Seoane, Tetrahedron, 2007, 63, 4095. CrossRef
42. J. C. Ramos, P. Bracco, M. Mazzini, J. R. Fernandez, D. Gamenara, and G. A. Seoane, Tetrahedron: Asymmetry, 2010, 21, 969. CrossRef
43. J. C. Ramos, M. Brovetto, and G. A. Seoane, Org. Lett., 2013, 15, 1982. CrossRef
44. M. G. Banwell, X. H. Ma, N. Asano, I. B. Kyoko, and J. N. Lambert, Org. Biomol. Chem., 2003, 1, 2035. CrossRef
45. M. G. Banwell and D. T. J. Loong, Org. Biomol. Chem., 2004, 2, 2050. CrossRef
46. K. A. B. Austin, M. G. Banwell, D. T. J. Loong, A. D. Rae, and A. C. Willis, Org. Biomol. Chem., 2005, 3, 1081. CrossRef
47. K. A. B. Austin, M. G. Banwell, G. J. Harfoot, and A. C. Willis, Tetrahedron Lett., 2006, 47, 7381. CrossRef
48. M. G. Banwell, K. A. B. Austin, and A. C. Willis, Tetrahedron, 2007, 63, 6388. CrossRef
49. M. G. Banwell, O. J. Kokas, and A. C. Willis, Org. Lett., 2007, 9, 3503. CrossRef
50. M. Matveenko, O. J. Kokas, M. G. Banwell, and A. C. Willis, Org. Lett., 2007, 9, 3683. CrossRef
51. O. J. Kokas, M. G. Banwell, and A. C. Willis, Tetrahedron, 2008, 64, 6444. CrossRef
52. M. Matveenko, M. G. Banwell, and A. C. Willis, Tetrahedron, 2008, 64, 4817. CrossRef
53. D. M. Pinkerton, M. G. Banwell, and A. C. Willis, Org. Lett., 2009, 11, 4290. CrossRef
54. M. G. Banwell, X. Ma, O. P. Karunaratne, and A. C. Willis, Aust. J. Chem., 2010, 63, 1437. CrossRef
55. L. V. White, B. D. Schwartz, M. G. Banwell, and A. C. Willis, J. Org. Chem., 2011, 76, 6250. CrossRef
56. D. J. Y. D. Bon, B. Lee, M. G. Banwell, and I. A. Cade, Chimica Oggi-Chemistry Today, 2012, 30, 22.
57. B. D. Schwartz, E. Matousova, R. White, M. G. Banwell, and A. C. Willis, Org. Lett., 2013, 15, 1934. CrossRef
58. T. Hudlicky, In Enzymes in Action; ed. by B. Zwanenburg; NATO ASI Series; Kluwer Academic, Dordrecht, 2000; p. 311.
59. A. M. Reiner and G. D. Hegeman, Biochemistry, 1971, 10, 2530. CrossRef
60. A. G. Myers, D. R. Siegel, D. J. Buzard, and M. G. Charest, Org. Lett., 2001, 3, 2923. CrossRef
61. M. D. Mihovilovic, H. G. Leisch, and K. Mereiter, Tetrahedron Lett., 2004, 45, 7087. CrossRef
62. M. A. Khan, J. P. Lowe, A. L. Johnson, A. J. W. Stewart, and S. E. Lewis, Chem. Commun., 2011, 47, 215. CrossRef
63. T. C. M. Fischer, H. G. Leisch, and M. D. Mihovilovic, Monatsh. Chem., 2010, 141, 699.
64. S. E. Lewis, S. Pilgrim, G. Kociok-Kohn, and M. D. Lloyd, Chem. Commun., 2011, 47, 4799. CrossRef
65. M. Ali Khan, M. F. Mahon, J. P. Lowe, A. J. W. Stewart, and S. E. Lewis, Chem. Eur. J., 2012, 18, 13480. CrossRef
66. M. J. Palframan, G. Kociok-Köhn, and S. E. Lewis, Chem. Eur. J., 2012, 18, 4766. CrossRef
67. D. R. Adams, C. Aichinger, J. Collins, U. Rinner, and T. Hudlicky, Synlett, 2011, 725. CrossRef
68. S. Danishefsky, T. Kitahara, P. F. Schuda, and S. J. Ethredge, J. Am. Chem. Soc., 1976, 98, 3028. CrossRef
69. (a) R. Hirschman, N. G. Steinberg, and R. Walker, J. Am. Chem. Soc., 1962, 84, 1270; CrossRef (b) E. Baer, J. Am. Chem. Soc., 1940, 62, 1597. CrossRef
70. S. Cicchi, A. Goti, A. Brandi, A. Guarna, and F. De Sarlo, Tetrahedron Lett., 1990, 31, 3351. CrossRef
71. V. VanRheenen, R. C. Kelly, and D. Y. Cha, Tetrahedron Lett., 1976, 17, 1973. CrossRef
72. B. Plietker and M. Niggemann, J. Org. Chem., 2005, 70, 2402. CrossRef
73. M. Nitta and T. Kobayashi, J. Chem. Soc., Perkin Trans. 1, 1985, 1401. CrossRef
74. S. D. Nielsen, G. Smith, M. Begtrup, and J. L. Kristensen, Eur. J. Org. Chem., 2010, 2010, 3704.
75. G. N. Jenkins, D. W. Ribbons, D. A. Widdowson, A. M. Z. Slawin, and D. J. Williams, J. Chem. Soc., Perkin Trans. 1, 1995, 2647. CrossRef
76. Y. L. Zhong and T. K. M. Shing, J. Org. Chem., 1997, 62, 2622. CrossRef