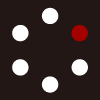
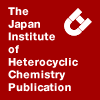
HETEROCYCLES
An International Journal for Reviews and Communications in Heterocyclic ChemistryWeb Edition ISSN: 1881-0942
Published online by The Japan Institute of Heterocyclic Chemistry
e-Journal
Full Text HTML
Received, 27th June, 2013, Accepted, 14th August, 2013, Published online, 21st August, 2013.
DOI: 10.3987/COM-13-S(S)54
■ Iodoetherification of Conformationally Restricted Dienyl Alcohols: Unexpected Formation of Oxocenes by 8-endo-mode Oxacyclizations
Kristen L. Stoltz, Andrea-Nekane R. Alba, Frank E. McDonald,* Marika B. Wieliczko, and John Bacsa
Department of Chemistry, Emory University, 1515 Dickey Drive, Atlanta, GA 30322, U.S.A.
Abstract
Iodine-promoted oxacyclizations of a family of conformationally restricted dienyl alcohols consistently afford oxocenes, arising from 8-endo-mode cyclizations.Fused polycyclic ether marine natural products exhibit a common trans,syn,trans-stereochemical motif with considerable variation in ring sizes from five- to nine-membered rings, as well as the number of cyclic ether rings within the structure.1 In addition to the hallmark structures of the brevetoxins, ciguatoxins, and maitotoxin, the pentacyclic natural product brevenal (1, Scheme 1) acts as a non-toxic competitive inhibitor of brevetoxins and ciguatoxin binding to sodium ion-channel receptors, counteracting the neurotoxic effects of exposure to the higher molecular weight polycyclic ethers.2 In contrast to our previous efforts to construct fused polycyclic ethers by endo-mode cascade oxacyclizations of polyepoxides,3-5 we have recently reported several cases of stereoselective six-membered ring ether (tetrahydropyran) formation from alkenyl diol cyclizations, with stereoinduction from an allylic oxygen substituent.6 The literature records several cases favoring 7-exo-mode oxacyclizations of alkenyl alcohol and alcohol derivatives (silyl ethers, isoxazolines) to form seven-membered ring ethers (oxepanes),7,8 but the effect of an allylic oxygen substituent on stereoinduction has not been described for this method of oxepane synthesis. Thus we sought to evaluate the oxacyclization behavior of alkenyl alcohol substrates corresponding to compound 2, with an acetal-protected diol corresponding to a model system for the B ring of brevenal (Scheme 1).
We envisioned that the erythro- stereochemistry of C14 and C15 in brevenal might arise from a carbohydrate starting material. Indeed a literature search revealed that compound 10 could be prepared from D-ribose (4, Scheme 2),9 and DIBAL-H reduction provided the dienyl alcohol 14 substrate. The disubstituted dienyl alcohols 15 and 16 were prepared by adapting the established synthetic route from the secondary alcohol 7.10 The incorporation of the internal cis-alkenes in dienyl alcohols 14 - 16 limited free rotation in the tethers relative to compound 17. For our purposes, the acetonide group not only isolated the primary alcohol in intermediate 5 for subsequent transformations, but also imparted additional conformational restriction in compounds 14 - 17 to favor cyclization. Although the methyl-substituted alkene diastereomers were inseparable as esters 11 or 13, pure samples of the dienyl alcohols 15 and 16 could be isolated by chromatography on silver nitrate-impregnated silica gel.11
Iodocyclization of the dienyl alcohol 14 gave one major product, which upon crystallographic characterization was revealed as the oxocene 18 arising from the 8-endo cyclization mode (Schemes 3, 4).12 In attempting to sterically block the 8-endo pathway, the cis,cis- and cis,trans-dienyl alcohols 15 and 16 were studied, but both compounds provided only the corresponding oxocenes 19 and 20, as single diastereomers and without any evidence of oxepane formation.13 The structural assignment for compound 19 was also confirmed by X-ray crystallography. Although compound 20 was not crystalline, the methyl doublets in the 1H NMR spectra for each deiodination product 23 and 24 were consistent with 8-membered ring formation.14 Moreover, deiodination of 19 and 20 produced different diastereomers, so we concluded by process of elimination that compound 20 must be the diastereomer of 19 at the position of the methyl substitution. To investigate if the cis-alkene in the tether was responsible for the unexpected regioselectivity, the hydroxyalkene 17 was studied (as a mixture of alkene isomers). However, iodocyclization also afforded the iodooxocane diastereomers 21, with additional structural confirmation upon deiodination, again showing methyl doublets for each diastereomer of oxocane 25.
Although this study did not provide the desired oxepanes (seven-membered ring ether), the regio- and stereoselectivity of this process is notable, and might be exploited in future synthetic applications for oxocene natural products.15 Current investigations towards achieving 7-exo-mode cyclizations are focused on changing the diol protective group, as the acetonide may be responsible for the 8-endo regioselectivity observed in this study.
EXPERIMENTAL
Representative Procedure for Iodine-promoted Oxacyclizations. The dienyl alcohol 15 (82 mg, 0.41 mmol) was dissolved in THF (4.1 mL), the solution was cooled to 0 ˚C, and solid NaHCO3 (265 mg, 3.2 mmol) was added, followed by I2 (624 mg, 2.5 mmol). The reaction mixture slowly warmed to room temperature over 19 h and was quenched with saturated aqueous Na2S2O3 (30 mL). The aqueous layer was extracted with EtOAc (3 x 15 mL), and the combined organic layers were dried over MgSO4, filtered, and concentrated under reduced pressure. The crude yellow material was purified by flash column chromatography (9:1 hexanes:EtOAc) to obtain iodooxocene 19 as a clear, colorless oil that solidified over time (77 mg, 58% yield).
(3aS,4S,9aS,Z)-4-Iodo-2,2-dimethyl-4,5,7,9a-tetrahydro-3aH-[1,3]dioxolo[4,5-d]oxocine (18): mp 65 - 67 ˚C. 1H NMR (CDCl3, 600 MHz) δ 5.64 - 5.58 (Ha, m, 1H), 5.39 - 5.34 (Hb, Hc, m, 2H), 4.52 - 4.47 (Hd, m, 1H), 4.45 (He, ddd, J1 = 5.0 Hz, J2 = 10.5 Hz, J3 = 12.5 Hz, 1H), 4.25 (Hf, dd, J1 = 5.8 Hz, J2 = 10.5 Hz, 1H), 4.03 (Hg, t, J = 12.5 Hz, 1H), 3.89 - 3.84 (Hh, m, 1H), 3.75 (Hi, dd, J1 = 5.0 Hz, J2 = 12.5 Hz, 1H), 1.50 (s, 3H), 1.41 (s, 3H). 13C NMR (CDCl3, 150 MHz) δ 129.9, 129.8, 107.4, 81.9, 76.9, 76.5, 71.9, 28.1, 26.7, 26.2. FT-IR (neat from a CH2Cl2 solution, the compound solidified upon standing, cm-1): 2986, 2918, 1380, 1368, 1262, 1248, 1222, 1164, 1106, 1081, 1055, 872, 738, 705 cm-1. HRMS Calcd for C10H16O3I1 (M+H)+: 311.01387, found 311.01466. [α]D25 +17.9 (c 0.6, CHCl3). Single crystals of compound 18 were obtained by slow evaporation of CDCl3. A suitable crystal was selected and mounted on an Apex2_Mo diffractometer. The crystal was kept at 173 K during data collection. Using Olex2, the structure was solved with the Superflip structure solution program using Charge Flipping and refined with the ShelXL refinement package using least squares minimization (SHELXL-97, Sheldrick, 2008).16 Crystal Data for C10H15IO3 (M = 310.12): monoclinic, space group P21, a = 7.9870(8) Å, b = 9.0616(9) Å, c = 8.3508(8) Å. Tables of structural parameters are provided in the supporting information. Deposition number CCDC-946585 for compound 18. Free copies of the data can be obtained via http://www.ccdc.cam.ac.uk/conts/retrieving.html (or from the Cambridge Crystallographic Data Centre, 12 Union Road, Cambridge, CB2 1EZ, UK; Fax: +44 1223 336033; e-mail: deposit@ccdc.cam.ac.uk).
(3aS,4S,5R,9aS,Z)-4-Iodo-2,2,5-trimethyl-4,5,7,9a-tetrahydro-3aH-[1,3]dioxolo[4,5-d]oxocine (19): mp 82 - 84 oC; 1H NMR (600 MHz, CDCl3) δ 5.54 (ddd, J = 12.0, 5.4, 4.2 Hz, 1H), 5.36 (ddd, J = 12.0, 6.0, 4.8 Hz, 1H), 5.28 (ddd, J = 6.0, 5.4, 2.4 Hz, 1H), 4.55 (dd, J = 11.1, 4.5 Hz, 1H), 4.46 (ddd, J = 18.6, 4.8, 4.2 Hz, 1H), 4.29 (dd, J = 11.1, 5.2 Hz, 1H), 3.91 – 3.85 (m, 2H), 1.53 (d, J = 6.5 Hz, 3H), 1.48 (s, 3H), 1.39 (s, 3H); 1H NMR (600 MHz, C6D6) δ 5.39 (m, 2H), 4.97 (ddd, J = 13.2, 6.0, 2.4 Hz, 1H), 4.48 (dd, J = 11.1, 4.8 Hz, 1H), 4.36 (dd, J = 11.1, 6.0 Hz, 1H), 3.93 (ddd, J = 18.6, 4.8, 2.4 Hz, 1H), 3.53 (qd, J = 6.6, 4.6 Hz, 1H), 3.04 (ddd, 18.6, 4.8, 2.4 Hz, 1H), 1.45 (s, 3H), 1.41 (d, J = 6.6 Hz, 3H), 1.33 (s, 3H). 13C NMR (101 MHz, CDCl3) δ 129.9 (2 carbons), 107.1, 80.5, 77.9, 76.1, 71.2, 29.3, 28.3, 26.6, 18.5. HRMS (APCI): m/z calcd. C11H18O3I (M+H+) 325.02951, found 325.02930. [α]D25 +24.0 (c 1.00, CHCl3). FT-IR (neat, cm-1): 2990, 2932, 2889, 1454, 1434, 1368, 1268, 1246, 1222, 1158, 1101, 1077, 1059, 1028, 935, 879, 711, 652. Single crystals of compound 19 were obtained by slow evaporation of CH2Cl2. Crystallography was conducted as described above, except that the crystal was kept at 109.1 K during data collection. Crystal Data for C11H17IO3 (M = 324.14): orthorhombic, space group P212121, a = 7.4901(6) Å, b = 10.2210(8) Å, c = 15.9521(12) Å. Tables of structural parameters are provided in the supporting information. Deposition number CCDC-946586 for compound 19.
(3aS,4S,5S,9aS,Z)-4-Iodo-2,2,5-trimethyl-4,5,7,9a-tetrahydro-3aH-[1,3]dioxolo[4,5-d]oxocine (20): 1H NMR (600 MHz, CDCl3) δ 5.61 (ddd, J = 11.8, 4.2, 2.7 Hz, 1H), 5.41 (ddd, J = 11.8, 2.6, 1.6 Hz, 1H), 5.33 (ddd, J = 6.3, 4.8, 2.4 Hz, 1H), 4.44 – 4.32 (m, 1H), 4.25 – 4.20 (m, 2H), 4.18 (ddd, J = 18.0, 3.4, 1.9 Hz, 1H), 4.12 (ddd, J = 18.1, 4.8, 2.6 Hz, 1H), 1.59 (d, J = 4.9 Hz, 3H), 1.48 (s, 3H), 1.40 (s, 3H); 1H NMR (600 MHz, C6D6) δ 5.47 – 5.36 (m, 2H), 5.04 (ddd, J = 13.2, 3.6, 2.4 Hz, 1H), 4.32 (dd, J = 10.2, 2.0 Hz, 1H), 4.14 – 4.05 (m, 2H), 3.68 (ddd, J = 18.0, 4.8, 2.4 Hz, 1H), 3.54 (ddd, J = 18.0, 4.8, 1.8 Hz, 1H), 1.46 (s, 3H), 1.32 (s, 3H), 1.26 (d, J = 6.1 Hz, 3H). 13C NMR (101 MHz, CDCl3) δ 131.4, 130.0, 107.3, 83.2, 77.5, 76.7, 62.0, 35.4, 28.3, 26.2, 17.7. FT-IR (neat, cm-1): 2984, 283, 1376, 1247, 1218, 1165, 1080, 1048, 910, 866, 732, 694, 650. [α]D25 +14.8 (c 1.94, CHCl3).
(3aS,4S,9aS)-4-Iodo-2,2,5-trimethylhexahydro-3aH-[1,3]dioxolo[4,5-d]oxocine (21), mixture of diastereomers at C5: 1H NMR (600 MHz, CDCl3) δ 4.61 (dd, J = 11.4, 4.8 Hz, 1H), 4.35 – 4.22 (m, 6H), 4.04 (ddd, J = 13.8, 7.2, 6.6 Hz, 1H), 3.93 – 3.84 (m, 2H), 3.56 (ddd, J = 11.4, 4.5, 2.7 Hz, 1H), 3.32 (td, J = 11.6, 2.4 Hz, 1H), 3.26 (t, J = 12.2 Hz, 1H), 1.93 – 1.82 (m, 1H), 1.80 – 1.69 (m, 3H), 1.69 – 1.61 (m, 4H), 1.60 (d, J = 6.5 Hz, 3H), 1.52 (d, J = 6.2 Hz, 3H), 1.43 (s, 6H), 1.36 (s, 3H), 1.36 (s, 3H). 13C NMR (150 MHz, C6D6) δ 105.83, 105.71, 83.02, 80.18, 79.67, 77.71, 77.45, 76.01, 68.48, 62.14, 34.85, 31.57, 30.58, 29.43, 29.17, 28.74, 26.52, 26.26 (2), 19.27, 18.63. HRMS (NSI): m/z calcd. C11H20IO3 (M+H+) 325.02951, found 325.02928. FT-IR (neat, cm-1): 2984, 2932, 2877, 1451, 1369, 1220, 1168, 1080, 1037, 961, 939, 866, 826, 753, 706. [α]D25 +10.8 (c 0.80, CHCl3).
ACKNOWLEDGEMENTS
This material is based upon work supported by the National Science Foundation under grant CHE-1151304. A.N.R.A. thanks MICINN for supporting her research fellowship at Emory University.
References
1. T. Yasumoto and M. Murata, Chem. Rev., 1993, 93, 1897; CrossRef T. Yasumoto, Chem. Rec., 2001, 1, 228; CrossRef T. Nakata, Chem. Rev., 2005, 105, 4314; CrossRef M. Inoue, Chem. Rev., 2005, 105, 4379; CrossRef M. Sasaki and H. Fuwa, Nat. Prod. Rep., 2008, 25, 401; CrossRef K. C. Nicolaou, M. O. Frederick, and R. J. Aversa, Angew. Chem. Int. Ed., 2008, 47, 7182. CrossRef
2. A. J. Bourdelais, H. M. Jacocks, J. L. C. Wright, P. M. Bigwarfe, and D. G. Baden, J. Nat. Prod., 2005, 68, 2; CrossRef A. J. Bourdelais, W. M. Abraham, S. K. Campbell, H. M. Jacocks, J. Naar, J. L. C. Wright, and D. G. Baden, Cell. Mol. Neurobiol., 2004, 24, 553; CrossRef T. D. Nguyen-Huu, C. Mattei, P. J. Wen, A. J. Bourdelais, R. J. Lewis, E. Benoit, D. G. Baden, J. Molgo, and F. A. Meunier, Toxicon, 2010, 56, 792; CrossRef H. Fuwa, M. Ebine, A. J. Bourdelais, D. G. Baden, and M. Sasaki, J. Am. Chem. Soc., 2006, 128, 16989; CrossRef H. Takamura, S. Kikuchi, Y. Nakamura, Y. Yamagami, T. Kishi, I. Kadota, and Y. Yamamoto, Org. Lett., 2009, 11, 2531; CrossRef Y. A. Zhang, J. Rohanna, J. Zhou, K. Iyer, and J. D. Rainier, J. Am. Chem. Soc., 2011, 133, 3208; CrossRef M. Ebine, H. Fuwa, and M. Sasaki, Chem. Eur. J., 2011, 17, 13754. CrossRef
3. J. C. Valentine, F. E. McDonald, W. A. Neiwert, and K. I. Hardcastle, J. Am. Chem. Soc., 2005, 127, 4586; CrossRef J. C. Valentine and F. E. McDonald, Synlett, 2006, 1816; CrossRef F. E. McDonald, R. B. Tong, J. C. Valentine, and F. Bravo, Pure Appl. Chem., 2007, 79, 281; CrossRef M. A. Boone, R. B. Tong, F. E. McDonald, S. Lense, R. Cao, and K. I. Hardcastle, J. Am. Chem. Soc., 2010, 132, 5300. CrossRef
4. For other approaches to endo-selective polyepoxide cyclizations, see: I. Vilotijevic and T. F. Jamison, Science, 2007, 317, 1189; CrossRef C. J. Morten, J. A. Byers, and T. Jamison, J. Am. Chem. Soc., 2011, 133, 1902; CrossRef D. J. Clausen, S. Wan, and P. E. Floreancig, Angew. Chem. Int. Ed., 2011, 50, 5178. CrossRef
5. For an example of the limitations of polyepoxide cyclization methodology, see: K. C. Nicolaou, J. H. Seo, T. Nakamura, and R. J. Aversa, J. Am. Chem. Soc., 2011, 133, 214. CrossRef
6. F. E. McDonald, K. Ishida, and J. A. Hurtak, Tetrahedron, 2013, 69, 7746. CrossRef
7. G. Lassalle and R. Grée, C. R. Acad. Sci. Paris, 1990, 310, Série II, 907; M. J. Kurth, M. J. Rodriguez, and M. M. Olmstead, J. Org. Chem., 1990, 55, 283; CrossRef Y. Brunel and G. Rousseau, J. Org. Chem., 1996, 61, 5793; CrossRef D. A. Ockey, D. R. Lane, J. A. Seeley, and N. E. Schore, Tetrahedron, 2000, 56, 711; CrossRef M. Pérez, P. Canoa, G. Gómez, C. Terán, and Y. Fall, Tetrahedron Lett., 2004, 45, 5207; CrossRef M. Pérez, P. Canoa, G. Gómez, M. Teijeira, and Y. Fall, Synthesis, 2005, 411; CrossRef D. S. Hamilton and D. A. Nicewicz, J. Am. Chem. Soc., 2012, 134, 18577. CrossRef
8. For examples in which 8-endo-mode cyclization competes with 7-exo-cyclization, see: (a) K. Fujiwara, H. Mishima, A. Amano, T. Tokiwano, and A. Murai, Tetrahedron Lett., 1998, 39, 393; CrossRef (b) T. Saitoh, T. Suzuki, N. Onodera, H. Sekiguchi, H. Hagiwara, and T. Hoshi, Tetrahedron Lett., 2003, 44, 2709; CrossRef (c) S. A. Snyder, D. S. Treitler, A. P. Brucks, and W. Sattler, J. Am. Chem. Soc., 2011, 133, 15898; CrossRef (d) S. A. Snyder, A. P. Brucks, D. S. Treitler, and I. Moga, J. Am. Chem. Soc., 2012, 134, 17714; CrossRef Radical processes generally favor 8-exo- over 7-exo-cyclizations: (e) E. Lee, C. H. Yoon, T. H. Lee, S. Y. Kim, T. J. Ha, Y.-S. Sung, S.-H. Park, and S. Lee, J. Am. Chem. Soc., 1998, 120, 7469; CrossRef (f) X. Fang, K. Liu, and C. Li, J. Am. Chem. Soc., 2010, 132, 2274. CrossRef
9. L. J. Baird, M. S. M. Timmer, P. H. Teesdale-Spittle, and J. E. Harvey, J. Org. Chem., 2009, 74, 2271. CrossRef
10. L. Beigelman, L. Blatt, and G. Wang, US Patent 2010/0249068 A1.
11. T.-S. Li, J.-T. Li, and H.-Z. Li, J. Chromatogr. A, 1995, 715, 372. CrossRef
12. The corresponding selenium-promoted cyclization of 14 (PhSeNPhth, CSA, CH2Cl2) provided the phenylselenium analog of 18 in low yield, which provided the oxocene 22 upon radical deselenylation.
13. The iodocyclization processes described herein required a considerable excess of iodine to maximize conversion of 14 - 17 to the corresponding cyclic ethers. The yields obtained for compounds 19 - 21 were obtained only when the molar amount of sodium bicarbonate exceeded the amount of iodine used.
14. We did not find any products from transannular cyclization onto the cyclic alkene in the radical deiodinations. The deiodination transformations were conducted primarily to confirm the ring sizes for the iodocyclization products, thus we did not attempt to optimize the yields for compounds 23 and 24. The yield of the compound 25 (from deiodination of the saturated cyclic ether 21) was notably higher, but a lower boiling solvent (benzene instead of toluene) was used for this transformation.
15. T. Irie, M. Suzuki, and T. Masamune, Tetrahedron Lett., 1965, 6, 1091; CrossRef A. F. Cameron, K. K. Cheung, G. Ferguson, and J. M. Robertson, Chem. Commun., 1965, 638; CrossRef T. Irie, M. Suzuki, and T. Masamune, Tetrahedron, 1968, 24, 4193. CrossRef
16. O. V. Dolomanov, L. J. Bourhis, R. J. Gildea, J. A. K. Howard, and H. Puschmann, J. Appl. Cryst., 2009, 42, 339. CrossRef
17. Supporting information is available on the Heterocycles website, http://www.heterocycles.jp/.