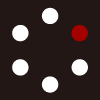
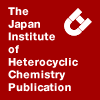
HETEROCYCLES
An International Journal for Reviews and Communications in Heterocyclic ChemistryWeb Edition ISSN: 1881-0942
Published online by The Japan Institute of Heterocyclic Chemistry
e-Journal
Full Text HTML
Received, 28th October, 2013, Accepted, 11th November, 2013, Published online, 15th November, 2013.
■ Asymmetric Alkylation of α-Cyanosulfones Catalyzed by Chiral 1,2,3-Triazolium Salts
Kohsuke Ohmatsu, Yusuke Hakamata, Ayano Goto, and Takashi Ooi*
Department of Applied Chemistry, Graduate School of Engineering, Nagoya University, Chikusa, Nagoya, Aichi 464-8601, Japan
Abstract
Chiral 1,2,3-triazolium salt-catalyzed asymmetric phase-transfer alkylation of α-cyanosulfones has been developed. This protocol is applicable to a variety of benzylic and allylic halides and cyanosulfones, affording chiral sulfones possessing tetrasubstituted carbons in excellent yields with good-to-high enantioselectivities.α-Chiral sulfonyl compounds are of increasing importance in medicinal chemistry primarily because of their intriguing biological activities; for example, dorzolamide,1 a carbonic anhydrase inhibitor, is a well-known antiglaucoma agent. Further, 4-substituted cyclohexyl sulfones show γ-secretase inhibitory activity and hence can be used to treat Alzheimer’s disease.2 In addition, sulfones having a tetrasubstituted chiral carbon at the α position have been recognized as promising candidates for biologically active sulfonyl compounds,3 and thus, establishment of a reliable method for their asymmetric synthesis is much sought after. However, there have been only a few successful examples of catalytic enantioselective reactions that fulfill this synthetic demand.4
Recently, we designed and synthesized optically pure 1,2,3-triazolium salts of type 1·X as a novel cationic chiral catalysts by exploiting the unique properties of the 1,2,3-triazole ring.5 These triazolium salts exhibited remarkable performance as chiral phase-transfer catalysts and were successfully applied to the development of asymmetric Mannich-type reactions of α-cyanosulfones under biphasic conditions.6 The reaction proceeded with excellent enantioselectivity but moderate diastereoselectivity, which was due to insufficient stereocontrol at the chiral center generated from the α-cyanosulfone component (Scheme 1), as revealed by single-crystal X-ray diffraction analyses of two diastereomers of a Mannich adduct. This observation prompted us to develop a system that enables the asymmetric construction of a tetrasubstituted stereogenic carbon center solely at the α position of the sulfonyl group. As an effective means of moving step forward in this direction, we here report the enantioselective phase-transfer alkylation7 of α-cyanosulfones catalyzed by chiral 1,2,3-triazolum salts.
We initially attempted to carry out the reaction of 2-(phenylsulfonyl)propanenitrile 2a with benzyl bromide in toluene and a saturated aqueous solution of KOH at 0 °C under the influence of triazolium bromide 1a·Br (Table 1, entry 1). Carbon–carbon bond formation was completed in 24 h and the desired alkylation product 3a was obtained in quantitative yield, albeit in racemic form. Subsequent trials with substrates bearing different substituents on the sulfonyl moiety (R1) under similar conditions revealed the influence of the structural feature of the sulfonyl substituent on the selectivity profile. While the benzylation of α-cyanosulfones with arylsulfonyl groups gave nearly racemic products regardless of the electronic properties of the aromatic functionalities (entries 2 and 3), the use of alkylsulfonyl-substituted cyanosulfones led to appreciable asymmetric induction. In particular, the reaction with 2-(isopropylsulfonyl)propanenitrile 2e afforded α-benzylated cyanosulfone 3e quantitatively
in 64.5:35.5 enantiomeric ratio (er) (entry 5).
For further optimization, we focused on structural modification of chiral 1,2,3-triazolium ion 1 (Table 2). In our previous studies, we recognized that the stereocontrolling ability of the triazolium ion was strongly affected by the substituent attached to the stereogenic carbon of the amino acid origin (R’). Thus, we screened several α-amino acid-derived catalysts and found that triazolium ion 1d prepared from L-cyclohexylalanine could promote the alkylation with good enantioselectivity (entries 1-3). Additional structural tuning by introducing an electron-withdrawing 4-chlorophenyl group as Ar’ delivered a notable increase in the product er, and triazolium ion 1e was identified to be the optimal catalyst for this alkylation (entry 4). Furthermore, we evaluated the effect of solvent and reaction temperature on the reactivity and selectivity, and found that changing the solvent to mesitylene and lowering the temperature to –20 °C resulted in the formation of 3e with a satisfactory level of enantiomeric purity without diminishing the catalytic efficiency (entry 7).
With the optimized catalyst structure and reaction conditions in hand, the generality of the 1e·Br-catalyzed asymmetric alkylation of α-cyanosulfones was explored; the representative results are summarized in Table 3. With benzylic bromides, the present system tolerated the incorporation of both electron-withdrawing and electron-donating substituents (entries 1-4). Asymmetric methallylation of 2e also proceeded smoothly, affording the corresponding product 3j quantitatively with a similar degree of enantioselectivity (entry 5).
Next, we examined the feasibility of extending this system to the alkylation of α-aryl-substituted α-cyanosulfones. Because of the high acidity of the α-proton in this substrate class, the alkylation was expected to proceed under the milder reaction conditions. Indeed, the reactions of 2-(isopropylsulfonyl)-2-phenylacetonitrile 2f with various benzylic or allylic bromides in the presence of 1e·Br could be carried out using a saturated aqueous solution of K2CO3 as a base at –30 °C to furnish the desired alkylated sulfones almost quantitatively with good-to-high stereoselectivities (entries 6-11). This asymmetric benzylation protocol could be extended to a range of α-cyanosulfones possessing electronically and sterically diverse aromatic α-substituents, including a fused aromatic unit (entries 12-17). However, incorporation of a heteroaryl appendage into the cyanosulfone component caused a decrease in the enantioselectivity (entries 18 and 19).
In conclusion, we developed an asymmetric phase-transfer alkylation of α-cyanosulfones utilizing chiral 1,2,3-triazolium salts as the requisite catalyst. This catalytic system was applicable to a variety of α-substituted α-cyanosulfones and reactive alkyl bromides such as benzylic bromides, in which good to high enantioselectivities were uniformly obtained. We believe that the present study expands the versatility of sulfonyl nucleophiles for preparing synthetically valuable α-chiral sulfonyl compounds.
EXPERIMENTAL
General procedure for 1e·Br-catalyzed asymmetric alkylation of α-cyanosulfones
Triazolium salt 1e·Br (2.93 mg, 0.003 mmol) and α-cyanosulfone 2e (16.1 mg, 0.1 mmol) were placed in a test tube and dissolved into 500 μL of mesitylene. Then, benzyl bromide (24 μL, 0.2 mmol) was introduced, and the resulting solution was cooled to –20 °C. To this solution was added saturated aqueous solution of KOH (6 μL, 0.12 mmol) and the whole reaction mixture was stirred for 24 h at the same temperature. The reaction was quenched by the addition of saturated aqueous solution of NH4Cl and the extractive workup was performed with EtOAc. After drying over Na2SO4, filtration, and removal of solvents, the resulting crude residue was purified by column chromatography (H/EtOAc = 5:1 as eluent) to afford 3e (24.9 mg, 0.099 mmol, 99% yield) as a colorless liquid. 3e: 1H NMR (400 MHz, CDCl3) δ 7.43-7.31 (5H, m), 3.67 (1H, sept, J = 6.9 Hz), 3.50 (1H, d, J = 13.7 Hz), 3.12 (1H, d, J = 13.7 Hz), 1.62 (3H, s), 1.59 (3H, d, J = 6.9 Hz), 1.58 (3H, d, J = 6.9 Hz); HPLC OD3, H/IPA = 10:1, flow rate = 1.0 mL/min, λ = 210 nm, 13.8 min (minor), 15.8 min (major).
ACKNOWLEDGEMENTS
This work was financially supported by CREST from JST, NEXT program, Program for Leading Graduate Schools “Integrative Graduate Education and Research Program in Green Natural Sciences” in Nagoya University, and the Daiko Foundation.
References
1. J. A. Balfour and M. I. Wilde, Drugs Aging, 1997, 10, 384; CrossRef M. F. Sugrue, A. Harris, and I. Adamsons, Drugs Today, 1997, 33, 283.
2. M. Teall, P. Oakley, T. Harrison, D. Shaw, E. Kay, J. Elliott, U. Gerhard, J. L. Castro, M. Shearman, R. G. Ball, and N. N. Tsou, Bioorg. Med. Chem. Lett., 2005, 15, 2685; CrossRef I. Churcher, D. Beher, J. D. Best, J. L. Castro, E. E. Clarke, A. Gentry, T. Harrison, L. Hitzel, E. Kay, S. Kerrad, H. D. Lewis, P. Morentin-Gutierrez, R. Mortishire-Smith, P. J. Oakley, M. Reilly, D. E. Shaw, M. S. Shearman, M. R. Teall, S. Williams, and J. D. J. Wrigley, Bioorg. Med. Chem. Lett., 2006, 16, 280; CrossRef J. P. Scott, S. F. Oliver, K. M. J. Brands, S. E. Brewer, A. J. Davies, A. D. Gibb, D. Hands, S. P. Keen, F. J. Sheen, R. A. Reamer, R. D. Wilson, and U. Dolling, J. Org. Chem., 2006, 71, 3086; CrossRef J. P. Scott, D. R. Lieberman, O. M. Beureux, K. M. J. Brands, A. J. Davies, A. W. Gibson, D. C. Hammond, C. J. McWilliams, G. W. Stewart, R. D. Wilson, and U. Dolling, J. Org. Chem., 2007, 72, 4149. CrossRef
3. V. Aranapakam, G. T. Grosu, J. M. Davis, B. Hu, J. Ellingboe, J. L. Baker, J. S. Skotnicki, A. Zask, J. F. DiJoseph, A. Sung, M. A. Sharr, L. M. Killar, T. Walter, G. Jin, and R. Cowling, J. Med. Chem., 2003, 46, 2361; CrossRef M. Fernández and J. Caballero, Bioorg. Med. Chem., 2007, 15, 6298. CrossRef
4. Y. Fukuda, K. Kondo, and T. Aoyama, Tetrahedron Lett., 2007, 48, 3389; CrossRef M. Belén Cid, J. López-Cantarero, S. Duce, and J. L. García Ruano, J. Org. Chem., 2009, 74, 431. CrossRef
5. K. Ohmatsu, M. Kiyokawa, and T. Ooi, J. Am. Chem. Soc., 2011, 133, 1307; CrossRef K. Ohmatsu, Y. Hamajima, and T. Ooi, J. Am. Chem. Soc., 2012, 134, 8794. CrossRef
6. K. Ohmatsu, A. Goto, and T. Ooi, Chem. Commun., 2012, 48, 7913. CrossRef
7. T. Ooi and K. Maruoka, Angew. Chem. Int. Ed., 2007, 46, 4222; CrossRef T. Hashimoto and K. Maruoka, Chem. Rev., 2007, 107, 5656; CrossRef S. Shirakawa and K. Maruoka, Angew. Chem. Int. Ed., 2013, 52, 4312. CrossRef