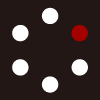
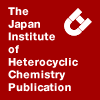
HETEROCYCLES
An International Journal for Reviews and Communications in Heterocyclic ChemistryWeb Edition ISSN: 1881-0942
Published online by The Japan Institute of Heterocyclic Chemistry
e-Journal
Full Text HTML
Received, 22nd June, 2013, Accepted, 12th July, 2013, Published online, 17th July, 2013.
DOI: 10.3987/COM-13-S(S)42
■ Synthesis and Molecular Structure of Cyclo[8](9,10-dihydro-9,10-anthraceno)pyrrole
Tetsuo Okujima,* Chie Ando, Shigeki Mori, Takahiro Nakae, Hiroko Yamada, and Hidemitsu Uno
Department of Chemistry and Biology, Graduate School or Science and Engineering, Ehime University, Bunkyo-cho 2-5, Matsuyama 790-8577, Japan
Abstract
Cyclo[8]pyrrole with eight 9,10-dihydroanthracene wings was synthesized by oxidative coupling of the corresponding 2,2'-bipyrrole in good yield. The molecular structure was determined by single crystal X-ray analysis. The three-dimensionally structured cyclo[8]pyrrole possessed a cavity created by a pair of slip-stacked cyclo[8]pyrroles.INTRODUCTION
Cyclo[n]pyrroles (n = 6, 7 and 8) belong to a family of ring-expanded porphyrins with no meso-bridges which was first reported as β-alkyl cyclo[8]pyrroles by Sessler and co-workers in 2002.1 The photophysical, anion–binding and liquid crystal properties have been studied in-depth, along with their electronic structures.2 Recently, some preparative examples of cyclo[8]pyrrole have been reported for derivatization at β-pyrrolic positions. For example, introduction of fused aromatic-rings resulted in the dramatic shift of the absorption bands, while the intense band was observed at around 1000 nm. The longest wavelength absorptions of cyclo[8]isoindole,3 cyclo[4]naphthobipyrrole,4 and cyclo[8]acenaphthopyrrole5 appeared at 1078, 1334 and 1515 nm with large absorption coefficient, ε, respectively. On the other hand, Sessler has reported the liquid crystalline cyclo[8]pyrroles self-organized by donor–acceptor interaction with trinitrobenzenes or trinitrofluorenone in 2007.2 We report herein the synthesis of biconcave cyclo[8]pyrrole, which possesses two wide cavities created by eight 9,10-dihydro-9,10-anthraceno moieties above and below porphyrin plane, for the purpose of the construction of the host–guest system by multi-pyrrolic macrocycles.
RESULTS AND DISCUSSION
The preparation of cyclo[8]pyrrole 6 is summarized in Scheme 1. Pyrrole 1 was prepared according to the literature procedure.6 After iodination of 1 by treating with benzyltrimethylammonium dichloroiodate (BTMA·ICl2), the protection of pyrrolic NH with di-t-butyl dicarbonate ((Boc)2O) gave pyrrole 3. Pyrrole 3 was dimerized by the Ullmann coupling reaction followed by deprotection with 1 M HCl and deesterification by heating with NaOH at 170 °C to give 2,2'-bipyrrole 5. Oxidative coupling of 5 afforded cyclo[8]pyrrole 6. Reaction conditions similar to those reported by Sessler1 were adopted with a 1 M H2SO4 solution of FeCl3 used as the oxidant. After purification by silica gel column chromatography and recrystallization, 6 was obtained in 61% yield as a sulfate similar to the reported cyclo[8]pyrroles (Table 1).1–5 The MALDI–TOF mass spectrum displays molecular ion peaks at m/z 2026. Cyclo[8]pyrrole 6 was characterized by physical and spectral methods including the X-ray crystallographic analysis. The nitrosonium ion generated from NaNO2 and acid was used as a mild oxidant to give 6 in 59% yield. When the reaction was carried out using Ce(SO4)2 as an oxidant together with H2SO4, Na2SO4, tetra(n-butyl)ammonium bisulfate (TBAHSO4),3 the best yield of 65% was obtained.
A single crystal of 6 suitable for X-ray structure determination was obtained after recrystallization from CHCl3/methanol. The crystal structure is shown in Figures 1 and 2, and the crystallographic data7–10 are summarized in experimental section. Cyclo[8]pyrrole 6 crystallized in a monoclinic cell, space group P21/c with Z = 4. The resulting structure was similar to those of bicyclo[2.2.2]octadiene-fused cyclo[8]pyrrole reported by our group3 and β-alkyl substituted ones by Sessler's group.1 Alternating pyrrole moieties tilt above and below the plane formed by the 16 α carbons with a mean deviation of 0.2133 Å. In contrast, displacements for bicyclo[2.2.2]octadiene-fused3 and β-alkyl substituted1 cyclo[8]pyrroles are 0.1891 and 0.4603 Å, respectively. Fused bicyclic-rings at β-pyrrolic positions resulted in a very flat conformation due to relatively small steric-hindrance between the neighboring bicyclo[2.2.2]octadiene moieties. Dihedral angles of 21.6–28.6° are observed between adjacent pyrrole moieties, while the inner SO42− ion is bound by hydrogen-bonding interactions with NH···O distances ranging from 1.921 to 2.426 Å. Distances between the opposite anthracene wings are ca. 14.6 and 16.8 Å (Figure 2b). In the molecular packing, there is a cavity between the slip-stacked cyclo[8]pyrrole planes. The distance between these planes is ca. 8.3 Å as shown in Figure 2c. Although solvent molecules were caged in the similar cavity of tetrakis(6,13-dihydro-6,13-pentaceno)porphyrins,11 no caged-solvent molecules were observed in this crystal.
The absorption spectrum of 6 is shown in Figure 3. The Soret and Q bands were observed at 467 nm (ε = 170,700 M−1 cm−1) and 1087 nm (ε = 330,800 M−1 cm−1). The Soret band was slightly blue-shifted whereas the Q band was red-shifted by ca. 50 nm compared to those of bicyclo[2.2.2]octadiene-fused cyclo[8]pyrrole. Inclusion behavior of 6 with π-systems in solution was examined by the measurement of the absorption spectra. When a solution of octaethylporphyrin (OEP) in CHCl3 was added dropwise to this yellow solution of 6, absorption spectra of OEP and 6 were observed independently. Novel absorptions arising from the formation of an adduct of 6 with OEP were not observed. Recrystallization from a 1 : 1 mixture in CHCl3/methanol afforded only crystals of 6. Crystals of 6 including OEP in their cavity were not obtained under these conditions.
In summary, we succeeded in synthesis and characterization of three-dimensionally structured cyclo[8]pyrrole 6, which exhibited the Soret band at visible region and the intense Q band at NIR region. X-Ray crystallographic analysis revealed that alternating pyrrole moieties tilted above and below the mean cyclo[8]pyrrole plane with the small deviation similar to the flat conformation of bicyclo[2.2.2]octadiene-fused cyclo[8]pyrrole.3 9,10-Dihydro-9,10-anthraceno moieties at β-pyrrolic positions created the cavities with a diameter of ca. 15 Å. A pair of slip-stacked cyclo[8]pyrroles was observed in the molecular packing. The further investigations for the formation of adduct of 6 with other π-systems are under way.
EXPERIMENTAL
General. Melting points were determined on a Yanaco micro melting point apparatus MP500D and are uncorrected. DI–EI and FAB mass spectra were measured on a JEOL JMS-700. MALDI–TOF mass spectra were measured on an Applied Biosystems Voyager-DE Pro. IR spectra were measured on a Horiba FT-720 infrared spectrophotometer, and UV–vis–NIR absorption spectrum was measured on a JASCO V-570 spectrophotometer. 1H NMR spectra (13C NMR spectra) were recorded on a JEOL AL-400 at 400 MHz (100 MHz). Elemental analyses were performed at the Integrated Center for Sciences, Ehime University.
Crystallographic analysis. X-Ray diffraction data were recorded at 100 K on a Rigaku CCD detector (Saturn 724) mounted on a Rigaku rotating anode X-ray generator (MicrMax-007HF) using Mo–Kα radiation from the corresponding set of confocal optics. Crystallographic data of 6: C144H88N8O4S, FW 2026.39, monoclinic, P21/c, Z = 2, a = 21.816(5) Å, b = 32.473(6) Å, c = 22.067(5) Å, α = 90.00º, β = 104.147(4)º, γ = 90.00º, V = 15159(5) Å3, µ (MoΚα) = 0.067 mm−1, unique 34678, Rint 0.1195, obs. 19084, param. 1415, R1 (I > 2σ(I)) 0.0958, wR2 (all data) 0.1848, GOF 1.013, CCDC No. 946058.
Ethyl 5-iodo-3,4-(9,10-dihydro-9,10-anthraceno)-1H-pyrrole-2-carboxylate 2
A solution of 1 (3.75 g, 11.9 mmol), BTMA·ICl2 (4.14 g) and CaCO3 (2.37 g) in CH2Cl2 (120 mL) and MeOH (60 mL) was refluxed for 3 h. After the reaction mixture was filtered through a Celite pad, the filtrate was washed successively with sat. aqueous Na2SO3, water and brine, dried over Na2SO4, and concentrated under reduced pressure. The residue was purified by column chromatography on silica gel with CH2Cl2 and washing with MeOH to give 2 (4.85 g, 92%).
White powder; mp 224.4–225.8 °C; 1H NMR (400 MHz, CDCl3) δ 8.21 (brs, 1H, NH), 7.36 (m, 4H, H-1',4',5',8'), 6.99 (m, 4H, H-2',3',6',7'), 5.76 (s, 1H, H-9'), 5.07 (s, 1H, H-10'), 4.35 (q, 2H, J = 7.1 Hz, CO2Et), 1.42 (t, 3H, J = 7.1 Hz, CO2Et); 13C NMR (100 MHz, CDCl3) δ 160.0, 145.9, 145.5, 139.8, 138.8, 125.2, 125.2, 123.9, 123.6, 120.1, 62.7, 60.5, 47.9, 47.8, 14.6; IR (KBr disk) νmax 3396, 3255, 1697, 1683 cm−1; MS (EI) m/z (rel intensity) 441 (M+, 100), 315 (6); Anal. Calcd for C21H16INO2: C, 57.16; H, 3.65; N, 3.17. Found: C, 57.43; H, 3.77; N, 3.13.
1-t-Butyl 2-ethyl 5-iodo-3,4-(9,10-dihydro-9,10-anthraceno)-1H-pyrrole-1,2-carboxylate 3
To a solution of 2 (1.50 g, 3.40 mmol) and DMAP (20 mg) in dry CH2Cl2 (20 mL) was added (Boc)2O (1.0 mL) at room temperature under an Ar atmosphere. The mixture was stirred at same temperature for 21 h, then washed successively with water and brine, dried over Na2SO4, and concentrated under reduced pressure. The residue was purified by column chromatography on silica gel with CHCl3 followed by recrystallization from CHCl3/hexane to give 3 (1.36 g, 74%).
White powder; mp 163.5–166.5 °C; 1H NMR (400 MHz, CDCl3) δ 7.36 (m, 4H, H-1',4',5',8'), 7.02 (m, 4H, H-2',3',6',7'), 5.67 (s, 1H, H-9'), 5.10 (s, 1H, H-10'), 4.37 (q, 2H, J = 7.1 Hz, CO2Et), 1.54 (s, 9H, CO2t-Bu), 1.44 (t, 3H, J = 7.1 Hz, CO2Et); 13C NMR (100 MHz, CDCl3) δ 159.3, 148.6, 144.8, 144.4, 141.3, 140.5, 125.6, 125.5, 124.1, 123.7, 121.5, 85.4, 66.6, 60.7, 47.9, 47.8, 27.6, 14.6; IR (KBr disk) νmax 1751, 1718 cm−1; MS (EI) m/z (rel intensity) 541 (M+, 11), 441 (100); Anal. Calcd for C26H24INO4: C, 57.68; H, 4.47; N, 2.59. Found: C, 57.69; H, 4.19; N, 2.54.
Diethyl 1H,1'H-2,2'-bis[3,4-(9,10-dihydro-9,10-anthraceno)pyrrole]-1,1'-dicarboxylate 4
A mixture of 3 (1.34 g, 2.48 mmol) and Cu powder (1.23 g) in dry DMF (20 mL) was heated at 110 °C for 18 h. The reaction mixture was filtered through a Celite pad to remove the copper and washed with CH2Cl2. The filtrate was extracted with CH2Cl2, washed successively with 1 M HCl, water and brine, dried over Na2SO4, and concentrated under reduced pressure. The residue was purified by column chromatography on silica gel with 30% EtOAc/CH2Cl2 followed by recrystallization from CHCl3/hexane to give 4 (697 mg, 90%).
White powder; mp >300 °C; 1H NMR (400 MHz, CDCl3) δ 8.31 (brs, 2H, NH), 7.44 (m, 8H, H-1',4',5',8'), 7.04 (m, 8H, H-2',3',6',7'), 5.84 (s, 2H, H-9'), 5.54 (s, 2H, H-10'), 4.33 (q, 4H, J = 7.1 Hz, CO2Et), 1.40 (t, 6H, J = 7.1 Hz, CO2Et); 13C NMR (100 MHz, CDCl3) δ 161.2, 146.0, 145.8, 139.0, 131.7, 125.2, 125.1, 124.0, 123.3, 119.4, 115.5, 60.4, 47.8, 47.1, 14.4; IR (KBr disk) νmax 3308, 1684 cm−1; MS (FAB) m/z 628 [M]+; Anal. Calcd for C42H32N2O4·1/4H2O: C, 79.66; H, 5.17; N, 4.42. Found: C, 79.88; H, 5.20; N, 4.39.
1H,1'H-2,2'-Bis[3,4-(9,10-dihydro-9,10-anthraceno)pyrrole] 5
A mixture of 4 (320 mg, 0.509 mmol) and NaOH (0.26 g) in ethylene glycol (10 mL) was heated at 170 °C for 2 h under an Ar atmosphere. The reaction mixture was poured into water and extracted with CHCl3. The organic layer was washed successively with water and brine, dried over Na2SO4, and concentrated under reduced pressure. The residue was rinsed with hexane, MeOH and ether to give 5 (182 mg, 74%).
White powder; mp 224.4–225.8 °C; 1H NMR (400 MHz, CDCl3) δ 7.51–7.35 (m, 8H, H-1',4',5',8'), 7.01–6.99 (m, 8H, H-2',3',6',7'), 6.60 (d, 2H, J = 3.4 Hz, H-5), 5.37 (s, 1H, H-9'), 5.33 (s, 1H, H-10'); IR (KBr disk) νmax 3412 cm−1; MS (EI) m/z (rel intensity) 484 (M+, 55); Anal. Calcd for C36H24N2·1/4CHCl3: C, 84.63; H, 4.75; N, 5.45. Found: C, 84.97; H, 4.77; N, 5.36.
Cyclo[8]pyrrole 6
Entry 1: 1 M H2SO4 (10 mL) and a solution of FeCl3·6H2O (0.29 g) in H2O (10 mL) were added dropwise slowly to a stirred solution of 5 (48 mg, 0.099 mmol) in CHCl3 (100 mL) at 0 °C. The mixture was stirred at room temperature for 24 h. After an addition of water, the organic layer was washed successively with water and brine, dried over Na2SO4, and concentrated under reduced pressure. The residue was purified by column chromatography on silica gel with CHCl3 followed by recrystallization from CHCl3/MeOH and rinsing with hexane, water and EtOH to give 6 (31 mg, 61%).
Entry 2: Conc. H2SO4 (0.1 mL) and a solution of NaNO2 (14 mg) in H2O (2 mL) were added dropwise to a stirred solution of 5 (97 mg, 0.20 mmol) in CHCl3 (200 mL) at 0 °C. The mixture was stirred at room temperature for 24 h. After concentration under reduced pressure, the residue was purified by column chromatography on silica gel with CHCl3 followed by recrystallization from CHCl3/MeOH to give 6 (60 mg, 59%).
Entry 3: Conc. H2SO4 (0.05 mL) and a solution of Ce(SO4)2 (60 mg) in H2O (1 mL) were added dropwise to a stirred mixture of 5 (49 mg, 0.10 mmol), TBAHSO4 (0.6 mg) and Na2SO4 (133 mg) in CHCl3 (100 mL) at 0 °C. The mixture was stirred at room temperature for 24 h. After an addition of water, the organic layer was washed successively with water and brine, dried over Na2SO4, and concentrated under reduced pressure. The residue was purified by column chromatography on silica gel with CHCl3 followed by recrystallization from CHCl3/MeOH and rinsing with hexane to give 6 (33 mg, 65%).
Yellow powder; mp >300 °C; 1H NMR (400 MHz, CDCl3) δ 8.25 (m, 32H, H-1',4',5',8'), 8.18 (s, 16H, H-9',10'), 7.28 (m, 32H, H-2',3',6',7'), −2.45 (brs, 8H, NH); UV–vis–NIR (CHCl3) λmax, nm (log ε) 467 (5.23), 1087 (5.52); MS (MALDI–TOF) m/z 2026 [M+H]+; Anal. Calcd for C144H88N8SO4·H2O: C, 84.60; H, 4.44; N, 5.48. Found: C, 84.39; H, 4.56; N, 5.35.
ACKNOWLEDGEMENTS
This work was partially supported by JSPS KAKENHI Grant Number 24750041 (TO) and MEXT KAKENHI Grant Number 23350020 (HU). We appreciate the gift of the ethyl isocyanoacetate, which was used in preparation of the starting pyrroles, by the Nippon Synthetic Chem. Ind. (Osaka Japan). We thank the Venture Business Laboratory, Ehime University, for their assistance in obtaining MALDI-TOF mass spectra.
References
1. D. Seidel, V. Lynch, and J. L. Sessler, Angew. Chem. Int. Ed., 2002, 41, 1422. CrossRef
2. J. L. Sessler, E. Karnas, S. K. Kim, Z. Ou, M. Zhanf, K. M. Kadish, K. Ohkubo, and S. Fukuzumi, J. Am. Chem. Soc., 2008, 130, 15256; CrossRef L. R. Eller, M. Stępień, C. J. Fowler, J. T. Lee, J. L. Sessler, and B. A. Moyer, J. Am. Chem. Soc., 2007, 129, 11020; CrossRef M. Stępień, B. Donnio, and J. L. Sessler, Angew. Chem. Int. Ed., 2007, 46, 1431; CrossRef A. Gorski, T. Köhler, D. Seidel, J. T. Lee, G. Orzanowska, J. L. Sessler, and J. Waluk, Chem. Eur. J., 2005, 11, 4179. CrossRef
3. T. Okujima, G. Jin, N. Matsumoto, J. Mack, S. Mori, K. Ohara, D. Kuzuhara, C. Ando, N. Ono, H. Yamada, H. Uno, and N. Kobayashi, Angew. Chem. Int. Ed., 2011, 50, 5699. CrossRef
4. V. V. Roznyatovskiy, J. M. Lim, V. M. Lynch, B. S. Lee, D. Kim, and J. L. Sessler, Org. Lett., 2011, 13, 5620; CrossRef T. Sarma and P. K. Panda, Chem. Eur. J., 2011, 17, 13987. CrossRef
5. T. Okujima, C. Ando, J. Mack, S. Mori, I. Hisaki, T. Nakae, H. Yamada, K. Ohara, N. Kobayashi, and H. Uno, Chem. Eur. J., 2013, in press.
6. S. Ito, T. Murashima, and N. Ono, J. Chem. Soc., Perkin Trans. 1, 1997, 3161. CrossRef
7. The diffraction data were corrected for Lorentz, polarization, and absorption effects. The structures were solved with SIR20048 and refined with SHELXL-97.9 All calculations were performed by using the Crystal Structure crystallographic software package.10 CCDC-946058 contains the supplementary crystallographic data for this paper. These data can be obtained free of charge from the Cambridge Crystallographic Data center via www.ccdc.cam.ac.uk/data_request/cif.
8. SIR2004: an improved tool for crystal structure determination and refinement, M. C. Burla, R. Caliandro, M. Camalli, B. Carrozzini, G. L. Cascarano, L. De Caro, C. Giacovazzo, G. Polidori, and R. Spagna, J. Appl. Cryst., 2005, 38, 381. CrossRef
9. SHELXL-97: Program for solution and refinement of crystal structures from diffraction data, University of Göttingen, Göttingen, Germany; "A short history of SHELX". G. M. Sheldrick, Acta Cryst., 2008, A64, 112.
10. Rigaku (2010) Crystal Structure. Version 3.8.2 or 4.0.1. Rigaku Corporation, Tokyo, Japan.
11. H. Uno, H. Watanabe, Y. Yamashita, and N. Ono, Org. Biomol. Chem., 2005, 3, 448. CrossRef