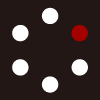
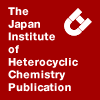
HETEROCYCLES
An International Journal for Reviews and Communications in Heterocyclic ChemistryWeb Edition ISSN: 1881-0942
Published online by The Japan Institute of Heterocyclic Chemistry
e-Journal
Full Text HTML
Received, 17th June, 2013, Accepted, 25th July, 2013, Published online, 31st July, 2013.
DOI: 10.3987/COM-13-S(S)35
■ Synthesis of Alkyl 3-Chloroazetidine-3-carboxylates via Regioselective Ring Transformation of Alkyl 2-(Bromomethyl)aziridine-2-carboxylates
Asta Žukauskaitė, Sven Mangelinckx, Algirdas Šačkus, and Norbert De Kimpe*
Department of Organic Chemistry, Faculty of Bioscience Engineering, Ghent University, Coupure Links 653, B-9000 Ghent, Belgium
Abstract
The synthesis of alkyl 3-chloroazetidine-3-carboxylates was developed by ring transformation of alkyl 2-(bromomethyl)aziridine-2-carboxylates utilizing ring opening with hydrochloric acid at the more sterically hindered carbon atom of the aziridine ring and subsequent base-promoted ring closure.2-(Chloromethyl)aziridines and 3-chloroazetidines are known in the literature since the 60’s when these heterocycles were introduced as the first 2-(halomethyl)aziridines and 3-haloazetidines, respectively.1 However, in comparison to the well explored and documented chemistry of 2-(bromomethyl)aziridines2 and 3-bromoazetidines,2h,2i,2l,3 the synthesis and application of their chlorinated analogues has received less attention in the literature.3a,4 More specifically, the synthesis of alkyl 2-(bromomethyl)aziridine¬2-carboxylates and alkyl 3-bromoazetidine-3-carboxylates was completed recently5 while their chlorinated analogues are still underdeveloped in the research field of the chemistry of aziridines and azetidines.
The synthesis of 2-(chloromethyl)aziridines has been accomplished through cyclization of O-mesylated 1-alkylamino-3-chloro-2-propanols,6 starting from 2-(bromomethyl)aziridines via a two-step sequence involving ring opening with hydrochloric acid and subsequent base-induced ring closure,1a direct aziridination,7 reductive cyclizations of β,γ-dichloroimines using NaBH4,4a or N-alkyl-α,α,β-trichloroimines with LiAlH4.8 On the other hand, 3-chloroazetidines were prepared from 3-(mesyloxy)azetidines by nucleophilic substitution with methanesulfonyl chloride,9 through reaction of 4-oxazolines with sulfur ylides,10 by reduction of 4-aryl-3-chloro-2-azetidinones,11 or by cyclization of β-chloro-γ-amino alcohol derivatives.12 Moreover, reaction of 1-azabicyclo[1.1.0]butanes with tosyl chloride,13 HCl,14 acid chlorides15 or chloroformate esters14a,16 afforded various 3-chloroazetidines by opening of the central bridging bond.
Even though these chlorinated substrates are less reactive towards nucleophilic substitution reactions,11b 2-(chloromethyl)aziridines have found application in ring opening reactions,4b while 3-chloroazetidines are valuable substrates for the synthesis of 2-azetines11a,17 and 1-azabicyclo[1.1.0]butanes.16a,c
Initially, in analogy with the aforementioned synthesis of alkyl 2-(bromomethyl)aziridine-2-carboxylates and alkyl 3-bromoazetidine-3-carboxylates,5 chlorination, instead of bromination, of alkyl 2-(aminomethyl)prop-2-enoates (1) was attempted in order to access alkyl 3-amino-2-chloro-2-(chloromethyl)propanoates (2), as convenient substrates for the synthesis of alkyl 2-(chloromethyl)aziridine-2-carboxylates (3) and alkyl 3-chloroazetidine-3-carboxylates (4).
Recently, a successful example of the use of chlorine gas for the functionalization of the double bond of allylamine was reported.4a However, due to the impracticability and toxicity of this chlorinating agent, an alternative and more convenient approach to access alkyl 3-amino-2-chloro-2-(chloromethyl)propanoates (2) was used. Thus, the amino group of alkyl 2-[(alkylamino)methyl]prop-2-enoates (1),5 was protected by treatment with hydrogen chloride in diethyl ether to provide the corresponding hydrochloride salts (Scheme 1). Subsequently, adopting a procedure for the chlorination of 3-chloro-2-methylpropene with sulfuryl chloride,18 crude hydrochloride salts were treated with one equivalent of sulfuryl chloride and the temperature of the reaction mixture was raised from room temperature to 70 °C within one hour. The temperature was maintained at 70 °C for an additional 30 minutes to obtain compounds (2a-d) in 81-99% yield after neutralisation and purification via column chromatography on silica gel.
As mentioned before, the synthesis of 2-(halomethyl)aziridines can be carried out through intramolecular ring closure of β,γ-dihaloamines. However, as compared to the reactivity of alkyl 3-amino2-bromo-2-(bromomethyl)propanoates,5 it can be expected that in order to achieve ring closure of alkyl 3-amino-2-chloro-2-(chloromethyl)propanoates (2), more harsh reaction conditions, such as prolonged reaction times or higher temperatures, might be required due to the weaker leaving group character of chloride as compared to bromide. Therefore, with alkyl 3-amino-2-chloro-2-(chloromethyl)propanoates (2) in hand, their ring closure towards alkyl 2-(chloromethyl)aziridine-2-carboxylates (3) and alkyl 3-chloroazetidine-3-carboxylates (4) was studied (Table 1). When ethyl 3-(tert-butylamino)-2-chloro-2-(chloromethyl)propanoate (2a) was heated with 1.5 equivalents of K2CO3 in acetonitrile at 60 °C for 16 hours, upon analysis of the 1H NMR spectrum of the crude reaction mixture, the corresponding azetidine (4a), as well as unreacted dichloropropanoate (2a) were observed in a ratio 2a/4a = 10/1 (Table 1, entry 1). An increased amount of the base, higher temperature or prolonged reaction times did not lead to full conversion and instead the formation of side products was noticed (Table 1, entries 2-3). Moreover, when 4 equivalents of K2CO3 were used and the reaction mixture was stirred in acetonitrile at 70 °C for 65 hours, the ratio between starting material (2a) and azetidine (4a) became worse, due to the observed formation of unidentified side products (Table 1, entry 4). When ethyl 3-(tert-pentylamino)-2-chloro-2-(chloromethyl)propanoate (2b) was heated with 30 equivalents of pyridine at 100 °C for 16 hours, analysis of the 1H NMR spectrum of the crude reaction mixture showed that only 13% of the substrate was converted into the corresponding azetidine (4b) (Table 1, entry 5). All other attempts with different bases (Table 1, entries 6-9) were unsuccessful due to poor conversions. The formation of the aziridines (3) was not observed in any of the attempts.
As the initial attempted synthesis of alkyl 2-(chloromethyl)aziridine-2-carboxylates (3) and/or alkyl 3-chloroazetidine-3-carboxylates (4) could not be accomplished due to the weak reactivity of dichloropropanoates (2), a different synthetic strategy was developed. As mentioned above, 2-(chloromethyl)aziridines can be obtained from 2-(bromomethyl)aziridines,1a which tend to ring open at the less sterically hindered aziridine carbon atom upon treatment with hydrochloric acid and can be subsequently ring closed by intramolecular nucleophilic displacement of the bromo substituent under basic conditions. On the other hand, if ring opening would occur at the more sterically hindered carbon atom, the synthesis of 3-chloroazetidines would be feasible. Thus, with several alkyl 2-(bromomethyl)aziridine-2-carboxylates (5a-d) available,5 their reactivity towards treatment with hydrochloric acid was studied (Scheme 2). When alkyl 2-(bromomethyl)aziridine-2-carboxylates (5a-d) were treated with 5-7 equivalents of 4M hydrochloric acid and stirred at 50 °C for 5 to 24 hours, 1H NMR analysis of the crude reaction mixtures, after neutralisation with NaOH, revealed that the expected attack at the unsubstituted aziridine carbon atom, which would have resulted in alkyl 2-amino-3-bromo-2-(chloromethyl)propanoates (6a-d) en route to alkyl 2-(chloromethyl)aziridine-2-carboxylates (3), did not occur. Instead, ring opening at the sterically hindered C-2 carbon atom took place giving rise to alkyl 3-amino-2-(bromomethyl)-2-chloropropanoates (7a-d) making alkyl 3-chloroazetidine-3-carboxylates (4a-d) accessible. The crude amines (7a-d) were obtained in nearly quantitative yields (purity > 90%) and could be used in the next step without further purification. Upon treatment of these substrates (7a-d) with 1.5 equivalents of K2CO3 and stirring in acetonitrile at 60 °C for 17-24 hours successfully afforded alkyl 3-chloroazetidine-3-carboxylates (4a-d) which were obtained in moderate overall yield (25-33%) after purification by preparative TLC. The moderate yields most probably result from the limited stability of compounds (4a-d) on silica gel.
In conclusion, the synthesis of alkyl 3-chloroazetidine-3-carboxylates was developed by ring opening of alkyl 2-(bromomethyl)aziridine-2-carboxylates with aqueous hydrochloric acid at the more sterically hindered carbon atom of the aziridine ring followed by base-promoted ring closure. Attempts to synthesize alkyl 2-(chloromethyl)aziridine-2-carboxylates and alkyl 3-chloroazetidine-3-carboxylates starting from alkyl 3-amino-2-chloro-2-(chloromethyl)propanoates were unsuccessful due to the weak leaving group character of chloride. This ring transformation of aziridines to azetidines adds to the rare examples of such heterocyclic conversions.2h,2i,2l,5,19
EXPERIMENTAL
Flame-dried glassware was used for non-aqueous reactions. Commercially available solvents and reagents were purchased from common chemical suppliers and used without further purification. The purification of reaction mixtures was performed through flash chromatography which was carried out using a glass column filled with silica gel (Acros, particle size 0.035-0.070 mm, pore diameter ca. 6 nm) or by preparative thin layer chromatography on silica gel (silica gel GF preparative layer with UV 254, 2 mm). The course of the reactions was monitored by initial TLC analysis on glass-backed silica plates (Merck Kieselgel, 60 F254, precoated 0.25 mm) which were developed using standard visualization techniques or agents: UV fluorescence (254 nm and 366 nm) and coloring with permanganate solution and heating. The migration rate of the compounds is described by the retention factor Rf (petroleum ether, hexane, EtOAc, Et2O). Compounds with boiling points lower than 150 °C were removed by rotary evaporation (20-30 mmHg) while for removal of high boiling compounds a high vacuum pump was used (0.01-2 mmHg). For NMR analysis, the compounds were diluted in CDCl3, quoted in parts per million (ppm) and referenced to tetramethylsilane (TMS, δ = 0) or the residual solvent peak. 1H NMR (300 MHz) and 13C NMR (75 MHz) spectra were recorded with a Jeol Eclipse FT 300 NMR spectrometer. Peak assignments were elucidated via HSQC and DEPT-135 techniques when required. Infrared spectra from samples in neat form were obtained from a Perkin Elmer Spectrum BX spectrometer with an ATR (Attenuated Total Reflectance) accessory. Only selected absorbences (υmax/cm-1) are reported. LC-MS was performed with Agilent 1100 Series VL (ES, 4000V) equipment or Agilent 1100 Series SL (ES, 4000V) equipment, performing electron-spray ionization at 4 kV (positive mode) or 3.5 kV (negative mode) and fragmentation at 70 eV, with only molecular ions ([MH]+) and major peaks being reported with intensities quoted as percentage of the base peak. Mass spectra were recorded on an Agilent 1100 series mass spectrometer using either a direct inlet system (electron spray, 4000V) or LC-MS coupling (UV detector). HRMS analysis was performed using an Agilent 1100 series HPLC coupled to an Agilent 6220 TOF-Mass Spectrometer equipped with an ESI/APCI-multimode source.
Starting materials. Alkyl 2-(aminomethyl)prop-2-enoates (1a-d) and alkyl 2-(bromomethyl)aziridine-2-carboxylates (5a-d) were prepared by previously reported procedures.5
Synthesis of alkyl 3-amino-2-chloro-2-(chloromethyl)propanoates (2a-d)
The synthesis of ethyl 3-(tert-butylamino)-2-chloro-2-(chloromethyl)propanoate (2a) is representative. Ethyl 2-[(tert-butylamino)methyl]prop-2-enoate (1a) (300 mg, 1.62 mmol) was dissolved in dry Et2O, cooled down to 0 °C and treated with HCl gas for 30 min. Evaporation of the solvent under reduced pressure afforded the corresponding crude hydrochloride salt in nearly quantitative yield. The hydrochloride salt was used in the next step without purification. To neat stirred ethyl 2-[(tert-butylamino)methyl]prop-2-enoate hydrochloride salt, sulfuryl chloride (219 mg, 0.13 mL, 1.62 mmol) was added dropwise at room temperature. The reaction mixture was gradually heated to 70 °C over a period of 1 h and maintained for 30 min. Subsequently, the reaction mixture was quenched with ice and saturated aq. NaHCO3 (5 mL) and extracted with EtOAc (3 × 15 mL). Drying of the combined organic extracts with MgSO4, filtration, evaporation of the solvent under reduced pressure and purification of the residue by flash chromatography on silica gel (petroleum ether / EtOAc 20/1) afforded pure compound (2a).
Ethyl 3-(tert-butylamino)-2-chloro-2-(chloromethyl)propanoate (2a). Light yellow oil, yield 81%, Rf = 0.48 (petroleum ether / EtOAc 9/1). 1H NMR (300 MHz, CDCl3): δ 1.08 (9H, s, C(CH3)3), 1.30 (1H, br s, NH), 1.32 (3H, t, J = 7.2 Hz, OCH2CH3), 3.14 (1H, d, J = 13.4 Hz, CH(H)NH), 3.19 (1H, d, J = 13.4 Hz, CH(H)NH), 4.02 (1H, d, J = 11.0 Hz, CH(H)Cl), 4.13 (1H, d, J = 11.0 Hz, CH(H)Cl), 4.27 (1H, dq, J = 11.0, 7.2 Hz, OCH(H)CH3), 4.29 (1H, dq, J = 11.0, 7.2 Hz, OCH(H)CH3). 13C NMR (75 MHz, CDCl3): δ 14.1, 29.4, 46.5, 46.6, 50.5, 62.6, 70.7, 168.3. IR (neat, cm-1): υNH = 3330 (weak), υC=O = 1741. MS (ES, pos. mode): m/z (%): 256/258/260 (M+H+, 100). HRMS Calcd for C10H19Cl2NO2: 256.0866 [M+H+]. Found: 256.0874/258.0844/260.0816 [M+H+].
Ethyl 3-(tert-amylamino)-2-chloro-2-(chloromethyl)propanoate (2b). Light yellow oil, yield 99%, Rf = 0.40 (petroleum ether / EtOAc 9/1). 1H NMR (300 MHz, CDCl3): δ 0.84 (3H, t, J = 7.5 Hz, CCH2CH3), 1.02 (6H, s, C(CH3)2), 1.03 (1H, br s, NH), 1.33 (3H, t, J = 7.2 Hz, OCH2CH3), 1.37 (2H, q, J = 7.5 Hz, CCH2CH3), 3.10 (1H, d, J = 12.9 Hz, CH(H)NH), 3.14 (1H, d, J = 12.9 Hz, CH(H)NH), 4.04 (1H, d, J = 11.0 Hz, CH(H)Cl), 4.14 (1H, d, J = 11.0 Hz, CH(H)Cl), 4.28 (1H, dq, J = 10.9, 7.1 Hz, OCH(H)CH3), 4.29 (1H, dq, J = 10.9, 7.1 Hz, OCH(H)CH3). 13C NMR (75 MHz, CDCl3): δ 8.3, 14.1, 26.8, 26.9, 33.6, 46.2, 46.7, 52.6, 62.6, 70.8, 168.4. IR (neat, cm-1): υNH = 3340 (weak), υC=O = 1741. MS (ES, pos. mode): m/z (%): 270/272/274 (M+H+, 100). HRMS Calcd for C11H21Cl2NO2: 270.1022 [M+H+]. Found: 270.1028/272.0998/274.0970 [M+H+].
Methyl 3-(tert-butylamino)-2-chloro-2-(chloromethyl)propanoate (2c). Light yellow oil, yield 95%, Rf = 0.50 (petroleum ether / EtOAc 9/1). 1H NMR (300 MHz, CDCl3): δ 1.08 (9H, s, C(CH3)3), 1.25 (1H, br s, NH), 3.17 (2H, s, CH2NH), 3.83 (3H, s, OCH3), 4.03 (1H, d, J = 11.0 Hz, CH(H)Cl), 4.14 (1H, d, J = 11.0 Hz, CH(H)Cl). 13C NMR (75 MHz, CDCl3): δ 29.3, 46.5, 46.7, 50.6, 53.4, 70.6, 168.9. IR (neat, cm-1): υNH = 3345 (weak), υC=O = 1744. MS (ES, pos. mode): m/z (%): 242/244/246 (M+H+, 100). HRMS Calcd for C9H17Cl2NO2: 242.0709 [M+H+]. Found: 242.0718/244.0688/246.0659 [M+H+].
Methyl 3-(tert-amylamino)-2-chloro-2-(chloromethyl)propanoate (2d). Light yellow oil, yield 95%, Rf = 0.55 (petroleum ether / EtOAc 9/1). 1H NMR (300 MHz, CDCl3): δ 0.84 (3H, t, J = 7.5 Hz, CH2CH3), 1.02 (6H, s, C(CH3)2), 1.21 (1H, br s, NH), 1.38 (2H, q, J = 7.5 Hz, CH2CH3), 3.11 (1H, d, J = 13.5 Hz, CH(H)NH), 3.15 (1H, d, J = 13.5 Hz, CH(H)NH), 3.83 (3H, s, OCH3), 4.04 (1H, d, J = 11.1 Hz, CH(H)Cl), 4.15 (1H, d, J = 11.1 Hz, CH(H)Cl). 13C NMR (75 MHz, CDCl3): δ 8.3, 26.8, 26.9, 33.6, 46.3, 46.6, 52.7, 53.4, 70.8, 168.9. IR (neat, cm-1): υNH = 3472 (weak), υC=O = 1745. MS (ES, pos. mode): m/z (%): 256/258/260 (M+H+, 100). HRMS Calcd for C10H19Cl2NO2: 256.0866 [M+H+]. Found: 256.0876/258.0845/260.0817 [M+H+].
Synthesis of alkyl 3-chloroazetidine-3-carboxylates (4a-d)
The synthesis of ethyl 1-tert-butyl-3-chloroazetidine-3-carboxylate (4a) is representative. Ethyl 2-(bromomethyl)-1-tert-butylaziridine-2-carboxylate (5a) (200 mg, 0.76 mmol) was stirred in 4M hydrochloric acid (0.95 mL, 3.78 mmol) at 50 °C for 16 h. After cooling, NaOH (212 mg, 5.3 mmol) was added, stirring was continued for an additional 30 min and the resulting reaction mixture was extracted with Et2O (3 × 15 mL). Drying of the combined organic extracts with MgSO4, filtration and evaporation of the solvent under reduced pressure afforded crude ethyl 2-(bromomethyl)-3-(tert-butylamino)-2- chloropropanoate (7a). The obtained crude ethyl 2-(bromomethyl)-3-(tert-butylamino)-2- chloropropanoate (7a) and K2CO3 (157 mg, 1.14 mmol) were heated in MeCN (5 mL) at 60 °C for 24 h. Then the solvent was removed under reduced pressure and Et2O (30 mL) was added. The resulting solution was filtered and the filter cake was washed with small portions of Et2O. Evaporation of the solvent under reduced pressure and purification of the residue by preparative TLC on silica gel (petroleum ether / Et2O 7/3) afforded pure compound (4a).
Ethyl 1-tert-butyl-3-chloroazetidine-3-carboxylate (4a). Light yellow oil, yield 33%, Rf = 0.39 (hexane / EtOAc 9/1). 1H NMR (300 MHz, CDCl3): δ 0.97 (9H, s, C(CH3)3), 1.33 (3H, t, J = 7.1 Hz, CH2CH3), 3.50 (2H, d, J = 8.5 Hz, CH(H)NCH(H)), 3.89 (2H, d, J = 8.5 Hz, CH(H)NCH(H)), 4.29 (2H, q, J = 7.1 Hz, CH2CH3). 13C NMR (75 MHz, CDCl3): δ 14.0, 24.1, 52.4, 56.5, 59.0, 62.5, 169.7. IR (neat, cm-1): υC=O = 1740. MS (ES, pos. mode): m/z (%): 220/222 (M+H+, 100).
Ethyl 1-tert-amyl-3-chloroazetidine-3-carboxylate (4b). Light yellow oil, yield 33%, Rf = 0.14 (petroleum ether / Et2O 9/1). 1H NMR (300 MHz, CDCl3): δ 0.83 (3H, t, J = 7.6 Hz, CCH2CH3), 0.89 (6H, s, C(CH3)2), 1.26 (2H, q, J = 7.6 Hz, CCH2CH3), 1.33 (3H, t, J = 7.1 Hz, OCH2CH3), 3.47 (2H, d, J = 9.1 Hz, CH(H)NCH(H)), 3.85 (2H, d, J = 9.1 Hz, CH(H)NCH(H)), 4.28 (2H, q, J = 7.1 Hz, OCH2CH3). 13C NMR (75 MHz, CDCl3): δ 8.6, 14.0, 20.1, 31.6, 54.7, 56.9, 58.8, 62.4, 169.9. IR (neat, cm-1): υC=O = 1741. MS (ES, pos. mode): m/z (%): 234/236 (M+H+, 100). HRMS Calcd for C11H20ClNO2: 234.1255 [M+H+]. Found: 234.1260/236.1231 [M+H+].
Methyl 1-tert-butyl-3-chloroazetidine-3-carboxylate (4c). Light yellow oil, yield 32%, Rf = 0.12 (petroleum ether / EtOAc 9/1). 1H NMR (300 MHz, CDCl3): δ 0.97 (9H, s, C(CH3)3), 3.50 (2H, d, J = 8.8 Hz, CH(H)NCH(H)), 3.84 (3H, s, OCH3), 3.88 (2H, d, J = 8.8 Hz, CH(H)NCH(H)). 13C NMR (75 MHz, CDCl3): δ 24.1, 52.3, 53.4, 56.4, 59.1, 170.3. IR (neat, cm-1): υC=O = 1745. MS (ES, pos. mode): m/z (%): 206/208 (M+H+, 100). HRMS Calcd for C9H16ClNO2: 206.0942 [M+H+]. Found: 206.0946/208.0918 [M+H+].
Methyl 1-tert-amyl-3-chloroazetidine-3-carboxylate (4d). Light yellow oil, yield 25%, Rf = 0.22 (hexane / EtOAc 9/1). 1H NMR (300 MHz, CDCl3): δ 0.84 (3H, t, J = 7.5 Hz, CCH2CH3), 0.89 (6H, s, C(CH3)2), 1.26 (2H, q, J = 7.5 Hz, CCH2CH3), 3.48 (2H, d, J = 8.6 Hz, CH(H)NCH(H)), 3.84 (3H, s, OCH3), 3.86 (2H, d, J = 8.6 Hz, CH(H)NCH(H)). 13C NMR (75 MHz, CDCl3): δ 8.6, 20.1, 31.5, 53.4, 54.7, 56.7, 58.9, 170.4. IR (neat, cm-1): υC=O = 1746. MS (ES, pos. mode): m/z (%): 220/222 (M+H+, 100). HRMS Calcd for C10H18ClNO2: 220.1099 [M+H+]. Found: 220.1103/222.1074 [M+H+].
ACKNOWLEDGEMENTS
The authors are grateful to the Research Foundation-Flanders (FWO-Flanders), Ghent University (BOF) and Kaunas University of Technology for financial support.
References
1. (a) W. J. Gensler, B. A. Brooks, and W. R. Koehler, J. Org. Chem., 1965, 30, 4365; CrossRef (b) V. R. Gaertner, Tetrahedron Lett., 1968, 9, 5919. CrossRef
2. (a) N. De Kimpe, D. De Smaele, and P. Bogaert, Synlett, 1994, 287; CrossRef (b) N. De Kimpe, R. Jolie, and D. De Smaele, J. Chem. Soc., Chem. Commun., 1994, 1221; CrossRef (c) D. De Smaele, P. Bogaert, and N. De Kimpe, Tetrahedron Lett., 1998, 39, 9797; CrossRef (d) H. Goossens, K. Vervisch, S. Catak, S. Stanković, M. D’hooghe, F. De Proft, P. Geerlings, N. De Kimpe, M. Waroquier, and V. Van Speybroeck, J. Org. Chem., 2011, 76, 8698; CrossRef (e) M. D'hooghe and N. De Kimpe, Chem. Commun., 2007, 1275; CrossRef (f) M. D’hooghe, W. Van Brabandt, and N. De Kimpe, Tetrahedron, 2003, 59, 5383; CrossRef (g) M. D’hooghe, M. Rottiers, I. Kerkaert, and N. De Kimpe, Tetrahedron, 2005, 61, 8746; CrossRef (h) S. Stanković, H. Goossens, S. Catak, M. Tezcan, M. Waroquier, V. Van Speybroeck, M. D’hooghe, and N. De Kimpe, J. Org. Chem., 2012, 77, 3181; CrossRef (i) S. Stanković, S. Catak, M. D’hooghe, H. Goossens, K. Abbaspour Tehrani, P. Bogaert, M. Waroquier, V. Van Speybroeck, and N. De Kimpe, J. Org. Chem., 2011, 76, 2157; CrossRef (j) M. D’hooghe, S. Kenis, K. Vervisch, C. Lategan, P. J. Smith, K. Chibale, and N. De Kimpe, Eur. J. Med. Chem., 2011, 46, 579; CrossRef (k) S. Stanković, M. D’hooghe, and N. De Kimpe, Org. Biomol. Chem., 2010, 8, 4266; CrossRef (l) S. Stanković, M. D’hooghe, K. Abbaspour Tehrani, and N. De Kimpe, Tetrahedron Lett., 2012, 53, 107. CrossRef
3. (a) For a recent review on the chemistry of 3-haloazetidines, see: W. Van Brabandt, S. Mangelinckx, M. D’hooghe, B. Van Driessche, and N. De Kimpe, Curr. Org. Chem., 2009, 13, 827; (b) Y. Ikee, K. Hashimoto, M. Kamino, M. Nakashima, K. Hayashi, S. Sano, M. Shiro, and Y. Nagao, Chem. Pharm. Bull., 2008, 56, 346; CrossRef (c) R. Bartnik, R. Faure, and K. Gebicki, J. Chem. Crystallogr., 1998, 28, 119. CrossRef
4. (a) S. Stanković, M. D’hooghe, J. Dewulf, P. Bogaert, R. Jolie, and N. De Kimpe, Tetrahedron Lett., 2011, 52, 4529; CrossRef (b) S. K. Kim and E. N. Jacobsen, Angew. Chem. Int. Ed., 2004, 43, 3952; CrossRef (c) T. Martinu and W. P. Dailey, J. Org. Chem., 2000, 65, 6784; CrossRef (d) J. A. Deyrup, C. L. Moyer, and P. S. Dreifus, J. Org. Chem., 1970, 35, 3428. CrossRef
5. (a) S. Mangelinckx, A. Žukauskaitė, V. Buinauskaitė, A. Šačkus, and N. De Kimpe, Tetrahedron Lett., 2008, 49, 6896; CrossRef (b) A. Žukauskaitė, S. Mangelinckx, V. Buinauskaitė, A. Šačkus, and N. De Kimpe, Amino Acids, 2011, 41, 541. CrossRef
6. V. R. Gaertner, J. Org. Chem., 1970, 35, 3952. CrossRef
7. (a) R. S. Atkinson, C. M. Darrah, and B. J. Kelly, Tetrahedron Lett., 1987, 28, 1711; CrossRef (b) R. S. Atkinson, J. Fawcett, I. S. T. Lochrie, S. Ulukanli, and T. A. Claxton, J. Chem. Soc., Perkin Trans. 2, 2002, 2, 819. CrossRef
8. M. D’hooghe, B. De Meulenaer, and N. De Kimpe, Synlett, 2008, 2437. CrossRef
9. E. Bacque, J.-M. Paris, and S. Le Bitoux, Synth. Commun., 1995, 25, 803. CrossRef
10. M. Vaultier, R. Danion-Bougot, D. Danion, J. Hamelin, and R. Carrié, J. Org. Chem., 1975, 40, 2990. CrossRef
11. (a) Y. Dejaegher, S. Mangelinckx, and N. De Kimpe, J. Org. Chem., 2002, 67, 2075; CrossRef (b) B. Van Driessche, W. Van Brabandt, M. D’hooghe, Y. Dejaegher, and N. De Kimpe, Tetrahedron, 2006, 62, 6882. CrossRef
12. (a) F. Colpaert, S. Mangelinckx, S. De Brabandere, and N. De Kimpe, J. Org. Chem., 2011, 76, 2204; CrossRef (b) S. Mangelinckx, B. De Sterck, F. Colpaert, S. Catak, J. Jacobs, S. Rooryck, M. Waroquier, V. Van Speybroeck, and N. De Kimpe, J. Org. Chem., 2012, 77, 3415. CrossRef
13. (a) W. Funke, Chem. Ber., 1969, 102, 3148; CrossRef (b) K. Hayashi, C. Sato, S. Hiki, T. Kumagai, S. Tamai, T. Abe, and Y. Nagao, Tetrahedron Lett., 1999, 40, 3761; CrossRef (c) G. Mlostoń, M. Woźnicka, J. Drabowicz, A. Linden, and H. Heimgartner, Helv. Chim. Acta, 2008, 91, 1419; CrossRef (d) K. Hayashi, Y. Ikee, S. Goto, M. Shiro, and Y. Nagao, Chem. Pharm. Bull., 2004, 52, 89. CrossRef
14. (a) K. Hayashi, S. Hiki, T. Kumagai, and Y. Nagao, Heterocycles, 2002, 56, 433; CrossRef (b) A. G. Hortmann and D. A. Robertson, J. Am. Chem. Soc., 1972, 94, 2758. CrossRef
15. A. P. Marchand and A. Devasagayaraj, Synth. Commun., 1999, 29, 885. CrossRef
16. (a) P. R. Dave, J. Org. Chem., 1996, 61, 5453; CrossRef (b) A. P. Marchand, G. V. M. Sharma, D. Rajagopal, R. Shukla, G. Mloston, and R. Bartnik, J. Heterocycl. Chem., 1996, 33, 837; CrossRef (c) R. Bartnik and A. P. Marchand, Synlett, 1997, 1029; CrossRef (d) M. Woznicka, K. Urbaniak, G. Mloston, and H. Heimgartner, Heterocycles, 2006, 69, 351; CrossRef (e) A. P. Marchand and A. Devasagayaraj, J. Org. Chem., 1997, 62, 4434; CrossRef (f) R. Bartnik, S. Leśniak, G. Mlostoń, and J. Romański, Polish J. Chem., 1994, 68, 1347.
17. S. Mangelinckx, V. Van Speybroeck, P. Vansteenkiste, M. Waroquier, and N. De Kimpe, J. Org. Chem., 2008, 73, 5481. CrossRef
18. A. Mooradian and J. B. Cloke, J. Am. Chem. Soc., 1946, 68, 785. CrossRef
19. (a) M. Karikomi and N. De Kimpe, Tetrahedron Lett., 2000, 41, 10295; CrossRef (b) N. De Kimpe, D. De Smaele, and Z. Szakonyi, J. Org. Chem., 1997, 62, 2448; CrossRef (c) A. Žukauskaitė, S. Mangelinckx, G. Callebaut, C. Wybon, A. Šačkus, and N. De Kimpe, Tetrahedron, 2013, 69, 3437. CrossRef