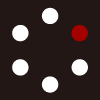
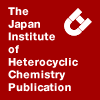
HETEROCYCLES
An International Journal for Reviews and Communications in Heterocyclic ChemistryWeb Edition ISSN: 1881-0942
Published online by The Japan Institute of Heterocyclic Chemistry
e-Journal
Full Text HTML
Received, 27th June, 2013, Accepted, 15th August, 2013, Published online, 22nd August, 2013.
DOI: 10.3987/COM-13-S(S)55
■ Synthesis of 2-Substituted 1,3-Cycloheptanedione via a Lewis Acid Mediated Ring Expansion Reaction
Kohei Inomata* and Yasuyuki Endo*
Faculty of Pharmaceutical Sciences, Tohoku Pharmaceutical University, 4-4-1 Komatsushima, Aoba-ku, Sendai 981-8558, Japan
Abstract
We have established a new route to provide 2-substituted 1,3-cycloheptanediones via a Lewis acid mediated ring expansion reaction of cyclobutanones as the key step. The ring expansion reactions were mediated by a series of Lewis acids. Among the used Lewis acids, ZnI2 was the most practical mediator. This route has succeeded in providing the title compounds even on a multi-gram scale. During the research, the Baeyer-Villiger oxidation of the cyclobutanones to obtain the new bicyclic lactones was also examined. The regioselective oxidation was observed in the case of chlorinated cyclobutanones.INTRODUCTION
We have recently established the chiral preparation of a Wieland-Miescher ketone analogue (1b) from 2-methyl-1,3-cycloheptanedione (2b).1 The several chiral amines bearing a heterocyclic moiety, such as pyrrolidine, tetrazole, or piperazine, mediated the asymmetric intramolecular aldol reaction of the trione (3) in the presence of trifluoroacetic acid (TFA) to afford 1b in an enantioselective fashion.1c Among the chiral amines, (S)-2-amino-1-phenyl-3-(pyrrolidin-1-yl)propane (4) was one of the most effective mediator to afford (R)-1b in high yield accompanied with over 80% ee. In connection with an ongoing synthetic project, we needed to prepare 2 bearing a variety of substituents (R1). However, there have been few reports regarding the preparation of 2-substituted 1,3-cycloheptanediones (2) except for only limited substituents (R1) such as methyl and ethyl groups (Figure 1). 2-4
For example, Hirsch et al. reported that the direct C-ethylation of 2a afforded 2c in low yield along with 3-ethoxy-2-cycloheptenone as a major product.4a The limited direct alkylation of 2a under basic conditions has also been reported by Swaminathan et al. They succeeded in preparation of 2b and 2c along with the recovery of the starting 2a.4b
Okamura and co-workers have reported the preparation of 2b from diethyl adipate (5) via an acyloin condensation, Simmos-Smith cyclopropanation, and subsequent oxidative ring expansion mediated by FeCl3 (Scheme 1).3c There have been some problems concerning the difficulties of the acyloin condensation on a large scale and of the effective preparation of 1,1-diiodoethane (7).5 Therefore, practical methods to prepare 2-substituted 1,3-cycloheptanedione (2) are still required.
We have been inspired by Ragan’s synthesis of 2a from 9 via the [2+2] cycloaddition and following zinc reduction of the chloride and the ring expansion of cyclobutanone.2g Although this process has been effective, the undesired 2-acetylcyclopentanone (11a) sometimes has been predominantly obtained under slightly different reaction conditions, especially on a large scale.2j Also, it has been difficult to handle the compound (10) due to its instability during the purification process such as column chromatography or distillation. In Ragan’s synthesis, we considered that the electron withdrawing dichloro substituents have promoted an undesired ring opening of the cyclobutanone in 10 and its instability against the purified process, and that the introduction of an electron donating group (EDG) on the cyclobutanone would be able to control the desired ring expansion to produce 2. We now report the preparation of bicyclic cyclobutanones (12) bearing a series of substituents (R1) and the regioselective ring expansion reaction of 12 mediated by Lewis acids to afford 2 (Scheme 2).
RESULTS AND DISCUSSION
First of all, we have started the [2+2] cycloaddition between 92j,6 and ketenes, which were prepared from a variety of acid halides (13), 7-9 in the presence of triethylamine (TEA).10,11 These results are summarized in Scheme 3 and Table 1. The chloroacetyl chloride (13a) and acetyl chloride (13d) hardly afforded 14a or 14d.11 On the other hand, 2-chloropropionyl chloride (13b) afforded the desired 14b as an inseparable mixture of two diastereomers regarding the methyl (R1) and chloro (X1) substituents. Based on these results, we considered that both the α-halo (X1) and α-alkyl (R1) substituents on 13 were needed to accelerate the effective [2+2] cycloaddition. The zinc reduction of 14b without a further purification of diastereomers and following solvolysis of the trimethysilyl group in aqueous 2-propanol (i-PrOH) smoothly proceeded to afford 12b as a single stereoisomer in 70% yield.
We next examined versatile acid halides (13) bearing alkyl, branched alkyl and benzyl substituents (R1) for these processes. Thus, the [2+2] cycloaddition using 13 and following zinc reduction afforded 12 as a single isomer (entries 3, 5-7). The α-bromo acid chloride (13f) and α-bromo acid bromide (13i) were also able to be used for the reaction. However, entry 9 showed that the zinc reduction of 14i bearing an α-bromo substituent revealed a lower yield than that of the corresponding chloride compound (14b). When 13h was used for the reaction, we could obtain 14h, but the zinc reduction of 14h afforded 11h as a mixture of its tautomers (15h and/or 16h) without the production of the desired 12h (entry 8).12 This result meant that an electron withdrawing substituent, such as a phenyl, at the α-position of the ketone (14) could not be used in this process. All of the compounds, (12) and (14), could be easily purified by silica gel column chromatography without any decomposition of the products. The reaction using 50 g of starting 9 also obtained almost the same results as entry 2 (entry 10).
The relative configuration of 12b was determined by NOE experiments as indicated in Scheme 4. From the NOE correlations, the stereochemistry between the methyl and hydroxyl substituents must be trans. The zinc reduction of the chloride in 14b produced the enol (17), and the following kinetic protonation proceeded from a convex face of the bicyclo[3.2.0]heptane skeleton in 17 to afford 12b as a single stereoisomer (Scheme 4).
As already described, 14b was obtained as a mixture of diastereomers. As a mixture of the both diastereomers afforded 12b as a single isomer, we next tried to separate them. Solvolysis of the TMS group in 14b rapidly proceeded to afford 18 and 19, which were readily separable by column chromatography. The NOE correlation between the methyl protons at C-7 and a methine proton at C-5 in 19 suggested the stereochemistry of 19 shown in Scheme 5. The zinc reduction of 18 and 19 respectively afforded 12b and we could not observe any diastereomers of 12b in both cases. These results support the kinetic protonation mechanism described above. However, the yields of 12b in the both cases were very low, because of the decomposition of the starting 18 or 19 during the reactions (Scheme 5).
At this stage, we examined the Baeyer-Villiger oxidation of the cyclobutanones to compare the electron donating character at C-5 and C-7 between 12b and 18 or 19. Thus, the treatment of 18 or 19 with m-chloroperbenzoic acid (mCPBA) in the presence of sodium hydrogen carbonate respectively afforded the lactone 20 or 21 as a single regioisomer. On the other hand, 12b afforded an inseparable mixture of 22 and 23 (22:23 = 2:3) in 74% yield under the same reaction conditions (Scheme 5). These results showed us that C-7 in 18 or 19 was the electron withdrawing character due to the chloro substituent, and that both C-5 and C-7 in 12b were almost the same electron donating character. Since the electron withdrawing chloro substituent in 18 or 19 would promote the undesired ring opening of the cyclobutanone moiety, 12b was selected as a substrate for continued ring expansion reactions.
Next, we examined the ring expansion reaction of 12b mediated by Lewis acidic or basic conditions.13,14 The results are summarized in Table 2. All the reactions were performed in the presence of 1.2 equivalents of the Lewis acidic or Lewis basic mediators to afford 2b accompanied by 11b, which were easily separable by silica gel column chromatography. The 1H-NMR of 11b in CDCl3 suggested that 11b was obtained as an inseparable 1:1 mixture of 11b and its tautomers (15b and/or 16b).15 First of all, according to Ragan’s method,2g 12b was exposed to acetic acid in aqueous 2-propanol. However, no reaction was observed. Basic conditions using potassium tert-butoxide (t-BuOK) in THF predominantly afforded the undesired 11b. Although most of the Lewis acidic conditions predominantly afforded 2b, the yields of 2b varied. When AlCl3 was used, the most selective reaction was observed. However, the yield of 2b slightly decreased and a longer reaction time was required. The stronger Lewis acid, such as ZrCl4 and GaCl3, also rapidly promoted the reaction, however, both the regioselectivity and yield of the desired 2b decreased. Since ZnI2 exhibited the highest yield of 2b among a variety of tested Lewis acids, we selected it as a mediator to develop further experiments.
We next examined the solvent effects of the ring expansion reaction of 12b mediated by a stoichiometric amount of ZnI2. The results are summarized in Table 3. Using nonprotic polar solvents, such as acetonitrile and THF, and a prolonged reaction time afforded the desired 2b in a lower yield than those in dichloromethane or 1,2-dichloroethane. Less polar solvents, such as toluene and hexane, decreased the yield of 2b. A catalytic reaction was also examined (entry 7). Thus, the reaction using 0.1 equivalent of ZnI2 prolonged the reaction time to completion and afforded 2b in a lower yield than the stoichiometric conditions. Therefore, we selected the stoichiometric ZnI2 in dichloromethane for further experiments.
Finally, the optimized conditions for the ring expansion reaction were used with 12 bearing versatile substituents (Table 4). Most of the substrates (12) predominantly afforded known or unknown cycloheptanediones (2) accompanied by a tautomeric mixture of 11, 15 and/or 16. The reaction using 27 g of 12b was also examined to observe the shorter reaction time and the slightly lower yield of 2 than the case on a small scale (entry 6). We have succeeded in preparing a 2-substituted 1,3-cycloheptanedione bearing an alkyl, branched alkyl and benzyl groups.
In conclusion, we have established a new route to provide 2-substituted 1,3-cycloheptanediones (2) via a Lewis acid mediated ring expansion reaction of cyclobutanones (12) as the key step. The ring expansion reactions were mediated by a series of Lewis acids. Among the used Lewis acids, ZnI2 was the most practical mediator. This route succeeded in providing the title compounds even on a multi-gram scale. During the research, the Baeyer-Villiger oxidation of the cyclobutanones to obtain the new bicyclic lactones was also examined. The regioselective oxidation was observed in the case of chlorinated cyclobutanones. The use of 2 to achieve the preparation of a new Wieland-Miescher ketone analogue (1) is currently in progress.
EXPERIMENTAL
Melting points are uncorrected. The 1H NMR spectra and 13C NMR spectra were recorded on a JEOL-AX-400 (1H 400 MHz, 13C 100 MHz) spectrometer and calibrated using trimethysilane as the internal standard. The mass spectra were recorded on a JEOL-DX-303 or JEOL JMS-MS700 spectrometer.
Typical procedure for [2+2] cycloaddition of 9 with 13 and subsequent zinc reduction of 14.
To a stirred solution of 9 (5 g, 32.1 mmol) and triethylamine (TEA, 8.0 mL, 57.7 mmol) in hexane (80 mL) was added 2-chlorobutyryl chloride (13c) (7.23 g, 51.3 mmol) in hexane (10 mL) in one portion at 0 oC. After stirring the mixture at rt for 10 min, the mixture was heated to reflux for 17 h. After cooling, the mixture was filtered to remove the TEA hydrochloride and the filtrate was washed with saturated aqueous NaHCO3 and brine. After drying (Na2SO4), the solvent was removed under reduced pressure. The residue was chromatographed (1% Et2O-hexane to 2% Et2O-hexane) to afford 14c (4.76 g, 56%) as a 85:15 diastereomeric mixture and a pale yellow oil. The compound (14c) was used for the next reaction without further purification. To a stirred solution of 14c (2.0 g, 7.68 mmol) in 2-propanol (20 mL) and H2O (10 mL) was added zinc powder (2.51 g, 38.4 mmol) in one portion at rt. The mixture was stirred at the same temperature for 51 h. The mixture was filtered through a Celite pad and the filtrate was evaporated under reduced pressure. The residue was dissolved in AcOEt and the mixture was washed with saturated aqueous NaHCO3 and brine. After drying (Na2SO4), the solvent was removed under reduced pressure. The residue was chromatographed (15% AcOEt-hexane) to afford 12c (643 mg, 54%) as a single stereoisomer and a colorless oil.
(1S*, 5R*, 7S*)-7-Ethyl-1-hydroxybicyclo[3.2.0]heptan-6-one (12c)
1H-NMR (CDCl3) δ 1.03 (t, J = 7.2 Hz, 3H), 1.33-1.49 (m, 2H), 1.58-1.83 (m, 3H), 1.85-1.98 (m, 2H), 2.08-2.14 (brs, 1H, D2O exchangeable), 2.22 (dd, J = 6.8 Hz, 13.2 Hz, 1H), 3.26-3.34 (m, 2H); 13C-NMR (CDCl3) δ 12.1, 16.7, 26.6, 27.8, 35.1, 68.5, 70.2, 79.8, 215.6; EIMS (m/z) 154 (M+), 126, 97 (100%), 84, 55; HRMS calcd for C9H14O2 154.0994. Found. 154.0995.
(1S*, 5R*, 7S*)-1-Hydroxy-7-methylbicyclo[3.2.0]heptan-6-one (12b)
Yiled: 70% (colorless oil); 1H-NMR (CDCl3) δ 1.06 (d, J = 7.2 Hz, 3H), 1.30-1.45 (m, 1H), 1.65-1.83 (m, 2H), 1.88 (dd, J = 5.8 Hz, 12.6 Hz, 1H), 1.95 (dd, J = 6.3 Hz, 12.6 Hz, 1H), 2.10-2.16 (brs, 1H, D2O exchangeable), 2.21 (dd, J = 6.3 Hz, 13.0 Hz, 1H), 3.35 (dd, J = 4.8 Hz, 8.2 Hz, 1H), 3.46-3.54 (m, 1H); 13C-NMR (CDCl3) δ 6.87, 26.3, 28.0, 34.8, 63.3, 68.6, 79.7, 216.4; EIMS (m/z) 140 (M+), 122, 112, 84 (100%), 83; HRMS calcd for C8H12O2 140.0837. Found. 140.0831.
(1R*, 5R*, 7S*)-7-Benzyl-1-hydroxybicyclo[3.2.0]heptan-6-one (12e)
Yiled: 41% (colorless oil); 1H-NMR (CDCl3) δ 1.43-1.60 (m, 1H), 1.68-1.86 (m, 2H), 1.88-2.03 (m, 2H), 2.03-2.14 (brs, 1H, D2O exchangeable), 2.28 (dd, J = 5.3 Hz, 13.5 Hz, 1H), 2.73 (dd, J = 9.2 Hz, 15.5 Hz, 1H), 3.02 (dd, J = 5.8 Hz, 15.5 Hz, 1H), 3.39 (dd, J = 4.3 Hz, 8.2 Hz, 1H), 3.81 (quint, J = 4.8 Hz, 1H), 7.19-7.24 (m, 1H), 7.25-7.34 (m, 4H); 13C-NMR (CDCl3) δ 26.8, 28.0, 29.3, 35.7, 68.80, 68.84, 80.2, 126.3, 128.4, 128.6, 128.9, 138.8, 213.5; EIMS (m/z) 216 (M+), 188, 97 (100%), 91, 84; HRMS calcd for C14H16O2 216.1150. Found. 216.1152.
(1R*, 5R*, 7S*)-1-Hydroxy-7-isopropylbicyclo[3.2.0]heptan-6-one (12f)
Yield: 47% (pale yellow oil); 1H-NMR (CDCl3) δ 0.97 (d, J = 6.8 Hz, 3H), 1.12 (d, J = 6.3 Hz, 3H), 1.37-1.51 (m, 1H), 1.70-1.96 (m, 5H), 2.00 (brs, 1H, D2O exchangeable), 2.27 (dd, J = 7.2 Hz, 12.6 Hz, 1H), 3.03 (dd, J = 4.3 Hz, 11.1 Hz, 1H), 3.25 (dd, J = 4.3 Hz, 7.7 Hz, 1H); 13C-NMR (CDCl3) δ 20.8, 20.9, 24.9, 26.8, 27.6, 35.7, 68.3, 75.1, 80.0, 214.0; EIMS (m/z) 168 (M+), 140, 97 (100%), 84; HRMS calcd for C10H16O2 168.1150. Found. 168.1149.
(1R*, 5R*, 7S*)-1-Hydroxy-7-isobutylbicyclo[3.2.0]heptan-6-one (12g)
Yield: 61% (pale yellow oil); 1H-NMR (CDCl3) δ 0.93 (d, J = 7.2 Hz, 6H), 1.25-1.53 (m, 3H), 1.66-1.82 (m, 3H), 1.84-1.97 (m, 2H), 2.05 (brs, 1H, D2O exchangeable), 2.21 (dd, J = 6.8 Hz, 13.5 Hz, 1H), 3.31 (dd, J = 4.3 Hz, 8.2 Hz, 1H), 3.45 (dd, J = 5.8 Hz, 14.0 Hz, 1H); 13C-NMR (CDCl3) δ 22.3, 22.5, 26.4, 26.5, 27.9, 32.2, 35.5, 67.1, 68.6, 80.1, 215.5; EIMS (m/z) 182 (M+), 154, 111, 84 (100%), 55; HRMS calcd for C11H18O2 182.1307. Found. 182.1301.
2-(2-Phenylacetyl)cyclopentanone (11h)
Yield: 56% (pale yellow oil) as an equilibrium mixture of 11h and its tautomers (15h and/or 16h); 1H-NMR (CDCl3) δ 1.75-2.09 (m, 2H), 2.18-2.32 (m, 1H), 2.41 (t, J = 7.7 Hz, 2H), 2.58 (t, J = 7.2 Hz, 1H), 3.48 (t, J = 8.2 Hz, 0.5H), 3.56 (s, 1H), 3.95 (d, J = 15.9 Hz, 0.5H), 4.01 (d, J = 15.5 Hz, 0.5H), 7.20-7.36 (m, 5H), 13.4-13.7 (brs, 0.5H, D2O exchangeable); 13C-NMR (CDCl3) δ 20.2, 20.5, 25.2, 25.6, 36.7, 38.8, 41.0, 49.7, 60.5, 190.7, 126.8, 127.0, 128.5, 128.6, 128.9, 129.6, 133.6, 135.2, 176.3, 202.0, 205.4, 212.8; EIMS (m/z) 202 (M+), 111 (100%), 91, 83; HRMS calcd for C13H14O2 202.0994. Found. 202.0991.
Solvolysis of 14b
A solution of 14b (1.16 g, 4.71 mmol) in MeOH (10 mL) was a stirred at rt for 1 h. The solvent was removed under reduced pressure and the residue was chromatographed (silica gel: spherical, 10% to 15% AcOEt-hexane) to afford 18 (414 mg, 51%) and 19 (276 mg, 34%).
(1R*, 5R*, 7R*)-7-Chloro-1-hydroxy-7-methylbicyclo[3.2.0]heptan-6-one (18)
Colorless oil; 1H-NMR (CDCl3) δ 1.34-1.48 (m, 1H), 1.57 (s, 3H), 1.82-2.00 (m, 2H), 2.01- 2.11 (m, 2H), 2.17 (dd, J = 6.8 Hz, 13.5 Hz, 1H), 3.08 (s, 1H, D2O exchangeable), 3.63 (d, J = 7.7 Hz, 1H); 13C-NMR (CDCl3) δ 16.9, 25.9, 28.3, 34.8, 69.2, 81.3, 83.0, 208.8; EIMS (m/z) 176 (M++2), 174 (M+), 138, 111 (100%), 84; HRMS calcd for C8H1135ClO2 174.0448. Found. 174.0453; HRMS calcd for C8H1137ClO2 176.0418. Found. 176.0417.
(1R*, 5R*, 7S*)-7-Chloro-1-hydroxy-7-methylbicyclo[3.2.0]heptan-6-one (19)
Colorless oil; 1H-NMR (CDCl3) δ 1.56-1.68 (m, 1H), 1.75 (s, 3H), 1.79-1.97 (m, 3H), 2.07 (dd, J = 6.3 Hz, 11.1 Hz, 1H), 2.16 (s, 1H, D2O exchangeable), 2.59 (dd, J = 6.8 Hz, 12.6 Hz, 1H), 3.47 (d, J = 8.7 Hz, 1H); 13C-NMR (CDCl3) δ 21.0, 26.2, 29.5, 38.6, 66.0, 78.4, 83.9, 209.2; EIMS (m/z) 176 (M++2), 174 (M+), 138, 111 (100%), 84; HRMS calcd for C8H1135ClO2 174.0448. Found. 174.0413; HRMS calcd for C8H1137ClO2 176.0418. Found. 176.0413.
Typical procedure for zinc reduction of 18 and 19
To a stirred solution of 18 (100 mg, 0.575 mmol) in a mixture of 2-propanol (3 mL) and H2O (3 mL) was added zinc powder (188 mg, 2.87 mmol) in one portion at rt. The mixture was stirred at rt for 49 h. The mixture was then filtered through a Celite pad and the filtrate was evaporated under reduced pressure. The residue was dissolved in AcOEt and was washed with saturated aqueous NaHCO3 and brine. After drying (Na2SO4), the solvent was removed under reduced pressure. The residue was chromatographed (15% AcOEt-hexane) to afford 12b (18 mg, 22%) as a single stereoisomer and colorless oil. All of the spectroscopic data were identical to 12b as already described.
Typical procedure for the Baeyer-Villiger oxidation of the cyclobutanones.
To a stirred suspension of 18 (100 mg, 0.575 mmol) and NaHCO3 (241 mg, 2.87 mmol) in CH2Cl2 (2 mL) was added mCPBA (149 mg, 0.862 mmol) in an ice bath. After stirring at the same temperature for 10 min, the mixture was further stirred at rt for 1.5 h. The mixture was filtered through a Celite pad and the filtrate was washed with 10 (w/v) % aqueous Na2SO3, saturated aqueous NaHCO3 and brine. After drying (Na2SO4), the solvent was removed under reduced pressure. The residue was chromatographed (20% AcOEt-hexane) to afford 20 (84 mg, 77%) as a single regioisomer and a colorless oil.
(3R*,3aR*,6aR*)-3-Chloro-3a-hydroxy-3-methylhexahydro-2H-cyclopenta[b]furan-2-one (20)
1H-NMR (CDCl3) δ 1.66-1.74 (m, 1H), 1.73 (s, 3H), 1.87-1.96 (m, 2H), 1.97-2.14 (m, 2H), 2.17-2.28 (m, 1H), 2.57 (s, 1H, D2O exchangeable), 4.59 (d, J = 5.3 Hz, 1H); 13C-NMR (CDCl3) δ 19.5, 23.8, 28.8, 33.0, 69.8, 87.5, 88.2, 172.0; EIMS (m/z) 192 (M++2), 190 (M+), 155, 111 (100%), 90, 83; HRMS calcd for C8H1135ClO3 190.0396. Found. 190.0388; HRMS calcd for C8H1137ClO3 192.0367. Found. 192.0358.
(3S*,3aR*,6aR*)-3-Chloro-3a-hydroxy-3-methylhexahydro-2H-cyclopenta[b]furan-2-one (21)
Yield: 65% (colorless needles); mp 83-84 oC (from Et2O-hexane); 1H-NMR (CDCl3) δ 1.56-1.72 (brs, 1H, D2O exchangeable), 1.80 (s, 3H), 1.82-2.11 (m, 4H), 2.16-2.36 (m, 2H), 4.56 (d, J = 5.3 Hz, 1H); 13C-NMR (CDCl3) δ 23.1, 23.8, 30.9, 37.8, 71.0, 86.8, 88.9, 174.1; EIMS (m/z) 192 (M++2), 190 (M+), 155, 111 (100%), 90, 83; HRMS calcd for C8H1135ClO3 190.0396. Found. 190.0403; HRMS calcd for C8H1137ClO3 192.0367. Found. 192.0370.
(3S*,3aS*,6aR*)-3a-hydroxy-3-methylhexahydro-2H-cyclopenta[b]furan-2-one (22) and (3S*,3aR*, 6aR*)-3a-hydroxy-3-methylhexahydro-1H-cyclopenta[c]furan-1-one (23)
Yield: 74% (colorless oil) as an inseparable mixture of 22 and 23; 1H-NMR (CDCl3) δ 1.26 (d, J = 7.2 Hz, 1.2H), 1.40 (d, J = 6.3 Hz, 1.8H), 1.50-2.80 (m, 6H, 1H: D2O exchangeable), 2.87 (q, J = 7.2 Hz, 0.4H), 2.93 (ddd, J = 1.6 Hz, 3.9 Hz, 7.7 Hz, 0.6H), 4.52 (q, J = 6.3 Hz, 0.6H), 4.54 (d, J = 6.3 Hz, 0.4H); 13C-NMR (CDCl3) δ 9.2, 14.2, 23.5, 25.0, 27.6, 30.8, 33.9, 34.3, 45.6, 53.1, 81.8, 86.8, 86.9, 88.9, 178.0, 179.0; EIMS (m/z) 156 (M+), 128, 100, 84 (100%), 72; HRMS calcd for C8H12O3 156.0786. Found 156.0780.
Typical procedure for a ring expansion reaction of 12.
To a stirred solution of 12b (100 mg, 0.714 mmol) in CH2Cl2 (3 mL) was added ZnI2 (274 mg, 0.857 mmol) at 0 oC. After stirring the mixture for 30 min, the mixture was further stirred at rt for 60 h. After adding saturated aqueous NaHCO3 at 0 oC, the mixture was filtered through a Celite pad. The filtrate was extracted with CH2Cl2. The combined organic layer was washed with brine and dried (Na2SO4). The solvent was removed under reduced pressure. The residue was chromatographed (10% Et2O-hexane to 15% Et2O-hexane) to afford 11b (14 mg, 14%) as a colorless oil and 2b (61 mg, 61%) as a colorless oil. The 1H-NMR spectrum of 11b in CDCl3 was observed as a 1:1 mixture of 11b and its tautomers (15b and/or 16b).
2-Methyl-1,3-cycloheptanedione (2b)3
1H-NMR (CDCl3) δ 1.23 (d, J = 6.8 Hz, 3H), 1.78-1.97 (m, 2H), 1.98-2.13 (m, 2H), 2.46-2.65 (m, 4H), 3.75 (q, J = 6.8 Hz, 1H); 13C-NMR (CDCl3) δ 11.1, 25.7, 43.3, 60.8, 208.0; EIMS (m/z) 140 (M+), 112, 97 (100%); HRMS calcd for C8H12O2 140.0837. Found. 140.0832.
2-Propanoylcyclopentanone (11b)15,16
1H-NMR (CDCl3) δ 1.06 (t, J = 7.2 Hz, 1.5H), 1.15 (t, J = 7.7 Hz, 1.5H), 1.79-1.96 (m, 1.5H), 2.01-2.13 (m, 1H), 2.27 (q, J = 7.7 Hz, 2H), 2.38-2.58 (m, 3H), 2.82-2.94 (m, 0.5H), 3.38 (t, J = 7.7 Hz, 0.5H), 13.4-13.8 (brs, 0.5H, D2O exchangeable); 13C-NMR (CDCl3) δ 7.2, 9.3, 20.2, 20.7, 25.3, 25.5, 27.9, 36.3, 36.5, 38.7, 61.6, 108.8, 181.4, 203.4, 205.1, 213.2; EIMS (m/z) 140 (M+), 111 (100%), 84; HRMS calcd for C8H12O2 140.0837. Found. 140.0839.
2-Ethyl-1,3-cycloheptanedione (2c)4
Yield: 62% (pale yellow oil); 1H-NMR (CDCl3) δ 0.87 (t, J = 7.2 Hz, 3H), 1.80-1.94 (m, 4H), 1.99-2.11 (m, 2H), 2.45-2.60 (m, 4H), 3.57 (t, J = 7.2 Hz, 1H); 13C-NMR (CDCl3) δ 11.5, 19.6, 25.7, 43.7, 68.3, 207.4; EIMS (m/z) 154 (M+), 126, 97 (100%), 55; HRMS calcd for C9H14O2 154.0994. Found. 154.0999.
2-n-Butyrylcyclopentanone (11c)17
Yield: 21% (pale pink oil) as an equilibrium mixture of 11c and its tautomers (15c and/or 16c); 1H-NMR
(CDCl3) δ 0.92 (t, J = 7.2 Hz, 1.5H), 0.97 (t, J = 7.7 Hz, 1.5H), 1.54-1.71 (m, 2H), 1.80-1.96 (m, 1.5H), 2.01-2.12 (m, 1H), 2.18-2.30 (m, 1.5H), 2.38 (m, 3.5H), 2.38-2.58 (m, 3.5H), 2.80 (td, J = 7.7 Hz, 17.4 Hz, 0.5H), 3.37 (t, J = 7.7 Hz, 0.5H), 13.5-13.8 (brs, 0.5H, D2O exchangeable); 13C-NMR (CDCl3) δ 13.5, 13.7, 16.6, 18.9, 20.2, 20.7, 25.2, 25.5, 36.2, 36.8, 38.6, 44.9, 61.7, 109.4, 178.9, 204.6, 205.1, 213.0; EIMS (m/z) 154 (M+), 126, 111 (100%), 84; HRMS calcd for C9H14O2 154.0994. Found. 154.0994.
2-Benzyl-1,3-cycloheptanedione (2e)18
Yield: 48% (colorless oil); 1H-NMR (CDCl3) δ 1.78-1.90 (m, 2H), 2.03-2.15 (m, 2H), 2.47 (ddd, J = 3.9 Hz, 8.7 Hz, 14.5 Hz, 2H), 2.55 (ddd, J = 4.3 Hz, 8.7 Hz, 14.5 Hz, 2H), 3.15 (d, J = 6.8 Hz, 2H), 4.22 (t, J = 6.8 Hz, 1H), 7.12-7.20 (m, 3H), 7.21-7.28 (m, 2H); 13C-NMR (CDCl3) δ 25.0, 31.8, 44.3, 68.3, 126.3, 128.4, 128.9, 139.1, 205.4; EIMS (m/z) 216 (M+, 100%), 188, 159, 131, 91; HRMS calcd for C14H16O2 216.1150. Found. 216.1149.
2-(3-Phenylpropanoyl)cyclopentanone (11e)19
Yield: 36% (pale yellow oil) as an equilibrium mixture of 11e and its tautomers (15e and/or 16e); 1H-NMR (CDCl3) δ 1.79-1.91 (m, 2H), 1.99-2.10 (m, 1H), 2.15-2.30 (m, 1H), 2.34-2.46 (m, 2.5H), 2.24 (t, J = 3.0 Hz, 1H), 2.75-2.95 (m, 2H), 3.20 (ddd, J = 4.8 Hz, 7.7 Hz, 16.4 Hz, 0.5H), 3.34 (t, J = 7.7 Hz, 0.5H), 7.14-7.32 (m, 5H), 13.30-13.80 (brs, 0.5H, D2O exchangeable); 13C-NMR (CDCl3) δ 20.2, 20.7, 25.2, 25.5, 29.3, 31.5, 36.4, 36.8, 38.7, 44.4, 62.0, 109.9, 126.0, 126.2, 128.3, 128.4, 140.7, 140.8, 177.8, 203.6, 204.8, 212.9; EIMS (m/z) 216 (M+), 198, 111, 91 (100%); HRMS calcd for C14H16O2 216.1150. Found. 216.1145.
2-Isopropyl-1,3-cycloheptanedione (2f)
Yield: 69% (colorless needles); mp 47.5-48 oC (from hexane); 1H-NMR (CDCl3) δ 0.92 (d, J = 6.8 Hz, 6H), 1.82-1.93 (m, 2H), 1.99-2.10 (m, 2H), 2.42-2.57 (m, 5H), 3.53 (d, J = 7.7 Hz, 1H); 13C-NMR (CDCl3) δ 20.1, 25.6, 26.3, 44.4, 73.4, 207.2; EIMS (m/z) 168 (M+), 150, 125, 97 (100%), 84, 69; HRMS calcd for C10H16O2 168.1150. Found. 168.1156.
2-(3-Methyl-n-butanoyl)cyclopentanone (11f)
Yield: 19% (colorless oil) as an equilibrium mixture of 11f and its tautomers (15f and/or 16f); 1H-NMR (CDCl3) δ 0.88-0.99 (m, 6H), 1.86-1.96 (m, 1.75H), 2.00-2.30 (m, 2.25H), 2.55 (t, J = 7.2 Hz, 1H), 2.61-2.69 (m, 0.25H), 3.36 (t, J = 7.7 Hz, 0.25H), 13.56-13.76 (brs, 0.75H, D2O exchangeable); 13C-NMR (CDCl3) δ 20.2, 20.6, 22.1, 22.2, 22.4, 23.1, 23.6, 23.8, 25.2, 26.2, 30.5, 34.3, 35.7, 36.8, 37.0, 38.6, 42.8, 42.9, 46.2, 46.5, 51.8, 61.9, 85.7. 110.0, 117.2, 204.3, 206.4, 213.0, 215.0; EIMS (m/z) 168 (M+), 153, 126, 111 (100%), 85, 57; HRMS calcd for C10H16O2 168.1150. Found. 168.1144.
2-Isobutyl-1,3-cycloheptanedione (2g)
Yield: 52% (colorless oil); 1H-NMR (CDCl3) δ 0.87 (d, J = 6.8 Hz, 6H), 1.49 (sept, J = 6.8 Hz, 1H), 1.72 (t, J = 7.2 Hz, 2H), 1.82-1.93 (m, 2H), 2.01-2.11 (m, 2H), 2.46-2.61 (m, 4H), 3.74 (t, J = 7.2 Hz, 1H); 13C-NMR (CDCl3) δ 22.4, 25.7, 25.9, 35.0, 43.6, 65.0, 207.7; EIMS (m/z) 182 (M+), 127 (100%), 111, 55; HRMS calcd for C11H18O2182.1307. Found. 182.1300.
2-(4-Methyl-n-pentanoyl)cyclopentanone (11g)
Yield: 34% (colorless oil) as an equilibrium mixture of 11g and its tautomers (15g and/or 16g); 1H-NMR (CDCl3) δ 0.89, 0.91 (pair of d, J = 6.3 Hz, 6.3 Hz, 6H), 1.43-1.65 (m, 3H), 1.81-1.96 (m, 1.5H), 2.00-2.12 (m, 1H), 2.19-2.30 (m, 2H), 2.38-2.58 (m, 3H), 2.77-2.87 (m, 0.5H), 3.39 (t, J = 8.2 Hz, 0.5H), 13.50-13.80 (brs, 0.5H, D2O exchangeable); 13C-NMR (CDCl3) δ 20.3, 20.7, 22.2, 22.3, 22.4, 25.4, 25.6, 27.5, 27.8, 31.9, 32.4, 34.3, 36.8, 38.7, 41.1, 61.8, 109.2, 179.8, 204.83, 204.86, 213.2; EIMS (m/z) 182 (M+), 139, 126, 111 (100%); HRMS calcd for C11H18O2182.1307. Found. 182.1304.
ACKNOWLEDGEMENTS
This work was supported by a Grant-in-Aid for Scientific Research from the Ministry of Education, Culture, Sports, Science and Technology of the Japanese Government.
References
1. (a) T. Nagamine, K. Inomata, Y. Endo, and L. A. Paquette, J. Org. Chem., 2007, 72, 123; CrossRef (b) T. Nagamine, K. Inomata, and Y. Endo, Chem. Pharm. Bull., 2007, 55, 1710; CrossRef (c) T. Nagamine, K. Inomata, and Y. Endo, Heterocycles, 2008, 76, 1191. CrossRef
2. Preparation of 2a: (a) B. Eistert, F. Haupter, and K. Schank, Liebigs Ann., 1963, 665, 55; CrossRef (b) I. Maclean and R. P. A. Sneeden, Tetrahedron, 1965, 21, 31; CrossRef (c) M. Suzuki, A. Watanabe, and R. Noyori, J. Am. Chem. Soc., 1980, 102, 2095; CrossRef (d) I. Nishiguchi and T. Hirashima, Chem. Lett., 1981, 551; CrossRef (e) Y. D. Vankar, N. C. Chaudhuri, and C. T. Rao, Tetrahedron Lett., 1987, 28, 551; CrossRef (f) Y. D. Vankar, M. V. Reddy, and N. C. Chaudhuri, Tetrahedron, 1994, 50, 11057; CrossRef (g) J. A. Ragan, T. W. Makowski, D. J. Ende, P. J. Clifford, G. R. Young, A. K. Conrad, and S. A. Eisenbeis, Org. Process Res. Dev., 1998, 2, 379; CrossRef (h) M. Kirihara, M. Ichinose, S. Takizawa, and T. Momose, Chem. Commun., 1998, 1691; CrossRef (i) M. Kirihara, H. Kakuda, M. Ichinose, Y. Ochiai, S. Takizawa, A. Mokuya, K. Okubo, A. Hatano, and M. Shiro, Tetrahedron, 2005, 61, 4831; CrossRef (j) J. Ragan, N. Do, R. McDermott, and A. Martius, Org. Synth., 2008, 85, 138.
3. Preparation of 2b: (a) A. C. Cope, P. Scheiner, and M. J. Youngquist, J. Org. Chem., 1963, 28, 518; CrossRef (b) R. Selvarajan, J. P. John, K. V. Narayakan, and S. Swaminathan, Tetrahedron, 1966, 22, 949; CrossRef (c) S. Lewicka-Piekut and W. H. Okamura, Synth. Commun., 1980, 10, 415. CrossRef
4. Preparation of 2c: (a) G. S. Thompson and J. A. Hirsch, J. Org. Chem., 1998, 63, 1098; CrossRef (b) D. Rajagopal, R. Narayanan, and S. Swaminathan, Proc. Indian Acad. Sci. 2001, 113, 197. CrossRef
5. R. L. Letsinger and C. W. Kammeyer, J. Am. Chem. Soc., 1951, 73, 4476. CrossRef
6. H. House, L. J. Czuba, M. Gall, and H. D. Olmstead, J. Org. Chem., 1969, 34, 2324. CrossRef
7. (a) D. N. Harpp, L. Q. Bao, C. J. Black, R. A. Smith, and J. G. Gleason, Tetrahedron Lett., 1974, 3235; CrossRef (b) D. N. Harpp, L. Q. Bao, C. J. Black, J. G. Gleason, and R. A. Smith, J. Org. Chem., 1975, 40, 3420. CrossRef
8. The acid halides (13) were commercially available or were easily prepared from the corresponding carboxylic acids by Harpp’s methods described in ref. 7 or by an acid-chlorination of the corresponding α-halocarboxylic acids with thionyl chloride. See also ref. 9. All of acid halides (13) were purified by distillation before use.
9. Some selected preparation of 13: (a) M. Furukawa, T. Okawara, and Y. Terawaki, Chem. Pharm. Bull., 1977, 25, 181; CrossRef (b) T. Tsuchiya and T. Takamoto, Jpn Kokai Tokkyo Koho, JP1996-183761 A; (c) R. Amoroso, G. Bettoni, B. De Filippis, and M. L. Tricca, Chirality, 1999, 11, 483; CrossRef (d) A. Ammazzalorso, R. Amoroso, G. Bettoni, and B. De Filippis, Chirality, 2001, 13, 102; CrossRef (e) F. Boschi, P. Camps, M. Comes-Franchini, D. Muñoz-Torrero, A. Ricci, and L. Sánchez, Tetrahedron: Asymmetry, 2005, 16, 3739. CrossRef
10. Some selected reviews of ketene cycloadditions: (a) J. A. Hyatt and P. W. Raynolds, Org. React., 1994, 45, 159; b) R. K. Orr and M. A. Calter, Tetrahedron, 2003, 59, 3545; CrossRef (c) A. D. Allen and T. T. Tidwell, Eur. J. Org. Chem., 2012, 1081. CrossRef
11. The [2+2] cycloaddition reactions of 9 with a variety of ketenes have been reported: (a) A. Hassner, H. W. Pinnick, and J. M. Ansell, J. Org. Chem., 1978, 43, 1774; CrossRef (b) L. R. Krepski and A. Hassner, J. Org. Chem., 1978, 43, 3173; CrossRef (c) W. T. Brady and R. M. Lloyd, J. Org. Chem., 1979, 44, 2560; CrossRef (d) W. T. Brady and R. M. Lloyd, J. Org. Chem., 1980, 45, 2025. CrossRef
12. Compound 11h was isolated as a single spot by thin layer chromatography (TLC). However, the 13C-NMR spectra of 11h in CDCl3 indicated that there were three carbonyl carbon signals at δ 202.0, 205.4, and 212.8 and two olefinic carbon signals at δ 109.7 and 176.3 due to the α,β-unsaturated carbon. Also, we observed a broad singlet proton at δ 13.4-13.7, which was suggested as a hydrogen-bonded enol proton in the 1H-NMR spectrum of 11h. These results showed that 11h was obtained along with its tautomers 15h and/or 16h. Since the two enolic forms 15h and 16h are in a rapid dynamic equilibrium, the peaks in the NMR spectrum for 15h and 16h cannot be distinguished. The same tendency was observed for all of the compounds 11 which were obtained by a ring expansion reaction. See also refs. 15 and 20.
13. M. Hesse, ‘Ring Enlargement in Organic Chemistry’, VCH, Weinheim, 1991.
14. Some selected recent reviews: (a) E. Leemans, M. D’hooghe, and N. De Kimpe, Chem. Rev., 2011, 111, 3268; CrossRef (b) T. Seiser, T. Saget, D. N. Tran, and N. Cramer, Angew. Chem. Int. Ed., 2011, 50, 7740. CrossRef
15. Change in the ratio (11b:15b and/or 16b) in the 1H-NMR spectrum in different deuterated solvents was observed as follows: 11b:15b and/or 16b = 1:1 (in C6D5CD3) and 2:1 (in DMSO-d6 or CD3CN). These results showed that 11b was obtained as an equilibrium mixture with its tautomer 15b and/or 16b.
16. (a) S. Hünig and W. Lendle, Chem. Ber., 1960, 93, 909; CrossRef (b) J. B. Paine, III, J. R. Brough, K. K. Buller, and E. E. Erikson, J. Org. Chem., 1987, 52, 3986. CrossRef
17. (a) R. M. Manyik, F. C. Frostick, Jr., J. J. Sanderson, and C. R. Hauser, J. Am. Chem. Soc., 1953, 75, 503; CrossRef (b) B. Kreidler, A. Baro, W. Frey, and J. Christoffers, Chem. Eur. J., 2005, 11, 2660. CrossRef
18. K. P. Cusack, T. D. Gordon, D. C. Ihle, M. E. Hayes, E. C. Breinlinger, A. M. Ericsson, B. Li, L. Wang, G. Y. Martinez, A. Burchat, A. D. Hobson, K. D. Mullen, M. Friedman, and M. J. Morytko, PCT Int. Appl., WO 2012/125797 A1.
19. T. M. Harris and C. R. Hauser, J. Am. Chem. Soc., 1959, 75, 1160. CrossRef
20. (a) S. Bolvig and P. E. Hansen, Magn. Reson. Chem., 1996, 34, 467; CrossRef (b) S. Moon and Y. Kwon, Magn. Reson. Chem., 2001, 39, 89. CrossRef