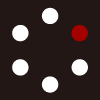
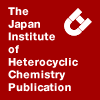
HETEROCYCLES
An International Journal for Reviews and Communications in Heterocyclic ChemistryWeb Edition ISSN: 1881-0942
Published online by The Japan Institute of Heterocyclic Chemistry
e-Journal
Full Text HTML
Received, 30th June, 2013, Accepted, 24th July, 2013, Published online, 1st August, 2013.
DOI: 10.3987/COM-13-S(S)72
■ Preparation and Electrochemical Property of Octaethylphthalocyanine Fused with Four Diselenadithiafulvalene Units
Takeshi Kimura*
Center for Instrumental Analysis, Iwate University, 4-3-5 Ueda, Morioka, Iwate 020-8551, Japan
Abstract
3,6-Diethylphthalonitrile (3) fused with diselenadithiafulvalene (DSDTF) was prepared from 5,6-dibromo-4,7-diethylbenzotriselenole (1) and treated with lithium alkoxide to produce octaethylphthalocyanine (6) with four DSDTF units. The structure of the product was determined by 1H NMR and MALDI-TOF-MS. Electrochemical and optical properties of 6 and its nickel complex (6-Ni) were examined by cyclic voltammetry and UV-vis spectroscopy.Besides actual use for dyes, pigments, and catalysts, phthalocyanines (Pcs) are expected to be applied to functional dyes in fields of solar cells, nonlinear optical materials, photodynamic therapy for cancer, and so on.1 In these applications, it is one of the most significant subjects that Pcs possess strong absorption (Q-band) at the near infrared region. The Q-band absorption can be modulated by the substituent effect; for example, Kobayashi and Cook reported that Pcs with the electron donating groups at the α-positions can show the absorption at the lower energy field compared with unsubstituted Pcs and β-substituted ones.2 On the other hand, there are many reports of hybrid molecules consisting of Pcs and tetrathiafulvalene (TTF),3 and Pcs, directly fused with four TTF scaffolds, were first reported by Decurtins.4 As related studies, we recently reported Pcs with long alkyl groups at the α-positions and four TTF units at the β-positions [Pc(TTF)4] and their unsymmetric derivatives.5,6 Diselenadithiafulvalene (DSDTF) is an analogous molecule of TTF and a strong electron donor similar to TTF and tetraselenafulvalene (TSF).7-9 Although DSDTF is known to be able to produce electron-transfer complexes, there is no report about hybrid molecules between DSDTF and Pcs. To construct a molecule consisted of Pc and four DSDTF units, phthalonitrile (3) fused with the DSDTF unit at the 4,5-positions was prepared as a precursor. In addition, eight ethyl groups at the α-positions of Pc and eight butylthio groups on the DSDTF units were introduced in order to shift the Q-band absorption to near infrared region and solubilize the product in common organic solvents. This paper reports the preparation, structure determination, and optical and electrochemical properties of octaethyl- phthalocyanine bearing the four DSDTF units.
4,5-(o-Xylylenediseleno)-3,6-diethylphthalonitrile (3) was prepared from 5,6-dibromo-4,7-diethylbenzotriselenole (1)6 via 4,5-(o-xylylenediseleno)-3,6-diethyl-1,2-dibromobenzene (2) (Scheme 1). In this process, the cyanation reaction of 2 was unfortunately low yield, which may depend on the lower bond energy of the Se-C bond compared with that of the S-C bond.5 Compound (3) was reacted with toluene in the presence of AlCl3 under argon for 2 h to eliminate the o-xylylene group, and then carbonyldiimidazole was added to the reaction mixture. The solution was stirred at 100 °C for 3 h to produce 3,6-diethylphthalonitrile (4) fused with a 1,3-diselenol-2-one ring in 67% yield. The o-xylylene group could be used as a protecting group for o-benzenediselenol. Thus, the deprotection of the o-xylylene group via the Se-C bond cleavage and the generation of o-benzenediselenol were conducted by this reaction. Compound (4) was reacted with 4,5-bis(butylthio)-1,3-dithiol-2-thione in triethylphosphite at 120 °C for 3 h to give 3,6-diethylphthalonitrile (5) with the DSDTF unit in 38% yield after silica gel column chromatography and recrystallization.10,11 For the tetramerization of phthalonitrile, lithium alkoxide prepared from lithium (31.7 mg, 4.6 mmol) in n-hexanol (1.5 ml) was added to 5 (200 mg, 0.3 mmol) and the reaction mixture was stirred at 120 °C for 3 h (Scheme 2). The reaction produced a blue-green suspension. The product was filtered, dried, and purified by silica gel column chromatography, which gave octaethylphthalocyanine (6) with the four DSDTF units in 33% yield (66.4 mg).12 Compound 6 was further reacted with nickel (II) acetate at 155 °C for 3 h to produce complex 6-Ni in 54% yield.13 Compounds 6 and 6-Ni are soluble in dichloromethane, chloroform, and THF. 1H NMR of 6 and 6-Ni showed clear signals for the ethyl and the butylthio groups. The inner pyrrole protons of 6 were observed at δ = –1.41 ppm in the spectrum. Since 6 and 6-Ni have eight selenium atoms, we could characterize the molecules by 77Se NMR spectroscopy. In the spectra, we could observe one signal at δ = 575.8 ppm for 6 and at δ = 578.7 ppm for 6-Ni. These signals appeared at the slightly higher magnetic field than that of 5 (δ = 587.4 ppm) and at the lower magnetic field than that of β-octakis(benzylseleno)octaethylphthalocyanine (δ = 356.0 ppm).6 Matrix assisted laser desorption ionization time of flight mass spectrometry (MALDI-TOF MS) experiments showed the molecular ion peak: m/z = 2529.3 [M+] for 6; m/z = 2585.6 [M+] for 6-Ni, which suggest the presence of the four DSDTF units on the central Pc core.
In the UV-vis spectrum, the Q-band absorption of 6 was observed at 759.5 nm (Figure 1a). In contrast, 6-Ni showed the Q band absorption at 734.0 nm as a sharp signal. While the Q band absorption of 6 appeared at the lower energy region about 25 nm than that of 6-Ni, it was the higher energy absorption about 10 nm than that of Pc(TTF)4 (770 nm). To determine the electrochemical property of 5, 6, and 6-Ni, redox potentials of them were measured by cyclic voltammetry using Ag/AgNO3 as a reference electrode (Table 1). In the voltammogram of 6, we could observe two quasi-reversible couples for oxidation (E1/2 = 0.36 and 0.78 V) and two quasi-reversible couples for reduction (E1/2 = –1.34 and –1.09 V). Although the voltammogram of 6-Ni was ambiguous compared with that of 6, we could found two quasi-reversible couples for oxidation (E1/2 = 0.35 and 0.79 V) and one irreversible peak potential for reduction (Ep = –1.20 V). While the oxidation potential of TTF is lower than that of the DSDTF and the potential difference is 0.07 V,14 the first oxidation potential of 5 is similar to that of TTF derivative (7) (difference: 0.01 V). It appears that the first oxidation potentials of 6 and 6-Ni are lower than those of 5 and 7. To estimate the HOMO–LUMO gap of the products, we calculated the potential difference (Eox1–Ered1) between the first oxidation and the first reduction potential. These potential differences could be correlated to the HOMO–LUMO gap (ΔEcalcd) calculated from the λmax values of the Q band absorption. The Q band absorption of 6 and 6-Ni could be changed by addition of an excess amount of iodine. The Q band absorption gradually decreased and new absorption appeared at the longer wavelength as a shoulder peak (Figure 1a). Apparently, 6 and 6-Ni would produce electron transfer complexes in the solution on treatment with iodine.
Next, iodine was added to 6 and 6-Ni, respectively, and both samples were measured with 1H NMR. However, no signals for the ethyl groups at the α-positions and for the butyl groups on the DSDTF units were observed in the spectra. On measuring the solution of 6/iodine with ESR spectroscopy, one strong broadened signal was found at g = 2.0139 in the spectrum. Similarly, we could observe one strong broadened signal for 6-Ni/iodine at g = 2.0147 (Figure 1b). When the solution of Pc(TTF)4/iodine was measured by the similar condition, one strong broadened signal was found at g = 2.0078. The g value of Pc(TTF)4/iodine can be related to a radical cation of TTF.15 In contrast, the g values of 6/iodine and 6-Ni/iodine are similar to that of the copper complex of pyrazinodiselenadithiafulvalene and smaller than that of the TSF radical cation.9 These results suggest that the electron transfer complexes generated from 6 and 6-Ni have a radical cationic character, and the charge generated on the DSDTF unit can delocalize on the π-aromatic system.
In conclusion, phthalocyanine (6) and its nickel complex (6-Ni), fused with the four DSDTF units, were prepared from 5,6-dibromo-4,7-diethylbenzotriselenole (1) via five and six step reactions, respectively. The first oxidation potentials of 6 and 6-Ni are lower than that of Pc(TTF)4. The electron transfer complexes, generated from 6 and 6-Ni on treatment with iodine, showed a radical cationic character, and the charge generated on the DSDTF unit could be delocalized on the whole molecule.
References
1. K. M. Kadish, K. M. Smith, and R. Guilard, ‘The Porphyrin Handbook’, Vols. 15-20, Academic Press, San Diego, 2003; J. Mack and N. Kobayashi, Chem. Rev., 2011, 111, 281. CrossRef
2. N. Kobayashi, H. Ogata, N. Nonaka, and E. A. Luk’yanets, Chem. Eur. J., 2003, 9, 5123; CrossRef P. M. Burnham, M. J. Cook, L. A. Gerrard, M. J. Heeney, and D. L. Hughes, Chem. Commun., 2003, 2064; CrossRef T. Fukuda, S. Masuda, M. Wahadoszamen, N. Ohta, and N. Kobayashi, Daiton Trans., 2009, 6065.
3. a) M. A. Blower, M. R. Bryce, and W. Devonport, Adv. Mater., 1996, 63; CrossRef b) M. J. Cook, G. Cooke, and A. Jafari-Fini, Chem. Commun., 1996, 1924; c) C. Farren, C. A. Christensen, S. FitzGerald, M. R. Bryce, and A. Beeby, J. Org. Chem., 2002, 67, 9130; CrossRef d) J. Sly, P. Kasák, E. Gomar-Nadal, C. Rovira, L. Górriz, P. Thordarson, D. B. Amabilino, A. E. Rowan, and R. J. M. Nolte, Chem. Commun., 2005, 1255; CrossRef e) R. Hou, B. Li, K. Zhong, H. Li, L.-Y. Jin, and B. Yin, Eur. J. Org. Chem., 2012, 1138. CrossRef
4. C. Loosli, C. Jia, S.-X. Liu, M. Haas, M. Dias, E. Levillain, A. Neels, G. Labat, A. Hauser, and S. Decurtins, J. Org. Chem., 2005, 70, 4988; CrossRef C. A. Donders, S.-X. Liu, C. Loosli, L. Sanguinet, A. Neels, and S. Decurtins, Tetrahedron, 2006, 62, 3543. CrossRef
5. T. Kimura, D. Watanabe, and T. Namauo, Heterocycles, 2008, 76, 1023; CrossRef T. Kimura, T. Namauo, N. Takahashi, Y. Takaguchi, T. Hoshi, and N. Kobayashi, J. Porphyrins Phthalocyanines, 2011, 15, 547; CrossRef T. Kimura, N. Takahashi, T. Tajima, and Y. Takaguchi, Heterocycles, 2012, 84, 333. CrossRef
6. T. Kimura, A. Yomogita, T. Matsutani, T. Suzuki, I. Tanaka, Y. Kawai, Y. Takaguchi, T. Wakahara, and T. Akasaka, J. Org. Chem., 2004, 69, 4716; CrossRef T. Kimura, N. Kanota, K. Matsui, I. Tanaka, T. Tsuboi, Y. Takaguchi, A. Yomogita, T. Wakahara, S. Kuwahara, F. Nagatsugi, and T. Akasaka, Inorg. Chem., 2008, 47, 3577. CrossRef
7. K. Kikuchi, I. Ikemoto, and K. Kobayashi, Synthetic Metals, 1987, 19, 551; CrossRef K. Kikuchi, M. Kikuchi, T. Namiki, K. Saito, I. Ikemoto, K. Murata, T. Ishiguro, and K. Kobayashi, Chem. Lett., 1987, 931; CrossRef K. Kanoda, T. Takahashi, K. Kikuchi, K. Saito, Y. Honda, I. Ikemoto, K. Kobayashi, K. Murata, and H. Anzai, Solid State Commun., 1989, 69, 415. CrossRef
8. M. Ashizawa, A. Akutsu, B. Noda, H. Nii, T. Kawamoto, T. Mori, T. Nakayahiki, Y. Misaki, K. Tanaka, K. Takimiya, and T. Otsubo, Bull. Chem. Soc. Jpn., 2004, 77, 1449; CrossRef T. Imakubo, T. Shirahata, M. Kibune, and H. Yoshino, Eur. J. Inorg. Chem., 2007, 4727; CrossRef Y. Bando, M. Ashizawa, T. Kawamoto, and T. Mori, Bull. Chem. Soc. Jpn., 2008, 81, 947; CrossRef M. Yasuda, E. Fujiwara, S. Aonuma, H. Fujiwara, T. Sugimoto, T. Nakanishi, K. Tanaka, K. Takahashi, H. Kobayashi, and Y. Misaki, Bull. Chem. Soc. Jpn., 2011, 84, 79; CrossRef H. Kamo, A. Ueda, T. Isono, K. Takahashi, and H. Mori, Tetrahedron Lett., 2012, 53, 4385. CrossRef
9. S. Ichikawa and H. Mori, Inorg. Chem., 2009, 48, 4643; CrossRef H. Kobayashi, H. Tomita, T. Naito, A. Kobayashi, F. Sakai, T. Watanabe, and P. Cassoux, J. Am. Chem. Soc., 1996, 118, 368. CrossRef
10. 4,5-Bis(butylthio)-1,3-dithiol-2-thione was prepared as an oily liquid by the procedure reported previously; G. Steimecke, H.-J. Sieler, R. Kirmse, and E. Hoyer, Phosphorus, Sulfur Silicon Relat. Elem., 1979, 7, 4.
11. 4,7-Diethyl-5,6-dicyano-2-(4’,5’-bis(butylthio)-1’,3’-dithiol-2’-ylidene)benzo[d]-1,3-diselenole (5): orange crystal; mp 148-149 °C; 1H NMR (500 MHz, CDCl3) δ 0.93 (t, J = 7.0 Hz, 6H, CH3), 1.30 (t, J = 7.6 Hz, 24H, CH3), 1.49 (sext, J = 7.4 Hz, 16H, CH2), 1.63 (quint, J = 7.4 Hz, 16H, CH2), 2.84 (t, J = 7.4 Hz, 16H, SCH2), 2.85 (q, J = 7.6 Hz, 4H, CH2); 13C NMR (126 MHz, CDCl3) δ 12.7, 13.4, 21.5, 31.6 (2 signals), 36.0, 93.2, 113.4, 114.5, 122.2, 128.2, 142.6, 146.9; 77Se NMR (95 MHz, CDCl3) δ 587.4; HRMS (EI): calcd for C24H28N2S4Se2, 631.9466; found, 631.9464 (M+).
12. 6: 1H NMR (500 MHz, CDCl3) δ -1.41 (s, 2H, NH), 0.98 (t, J = 7.4 Hz, 24H, CH3), 1.45-1.59 (m, 40H, CH3, CH2), 1.69 (quint, J = 7.3 Hz, 4H, CH2), 2.91 (t, J = 7.3 Hz, 8H, CH2), 4.26 (bs, 16H, CH2); 77Se NMR (95 MHz, CDCl3) δ 575.8; MALDI-TOF-MS (m/z) 2529.3 [M+].
13. 6-Ni: 1H NMR (500 MHz, CDCl3) δ 0.96 (t, J = 7.4 Hz, 24H, CH3), 1.37 (t, J = 7.5 Hz, 24H, CH3), 1.49 (sext, J = 7.4 Hz, 16H, CH2), 1.68 (quint, J = 7.4 Hz, 16H, CH2), 2.90 (t, J = 7.4 Hz, 16H, SCH2), 4.18 (bs, 16H, CH2); 77Se NMR (95 MHz, CDCl3) δ 578.7; MALDI-TOF-MS (m/z) 2585.6 [M+].
14. E. M. Engler, F. B. Kaufmen, D. C. Green, C. E. Klots, and R. N. Compton, J. Am. Chem. Soc., 1975, 97, 2921. CrossRef
15. F. Wudl, G. M. Smith , and E. J. Hufnagel, J. Chem. Soc., Chem. Commun., 1970, 1453.